Abstract
Intrahepatic cholangiocarcinoma (ICC), a malignant neoplasm of the biliary epithelium, is usually fatal because of difficulty in early diagnosis and lack of availability of effective therapy. The genetic mechanisms involved in the development of ICC are not well understood and only a few cytogenetic studies of ICC have been published. Recently, technique of degenerate oligonucleotide primed (DOP)-PCR comparative genomic hybridization (CGH) permits genetic imbalances screening of the entire genome using only small amounts of tumor DNA. In this study chromosomal aberrations in 33 Korean ICC were investigated by DOP-PCR CGH. The common sites of copy number increases were 20q (67%), 17 (61%), 11q11-q13 (42%), 8p12-qter (39%), 18p (39%), 15q22-qter (36%), 16p (36%), 6p21 (30%), 3q25-qter (27%), 1q41-qter (24%), and 5p14-q11.2 (24%). DNA amplification was identified in 16 carcinomas (48%). The frequent sites of amplification were 20q, 17p, 17q23-qter, and 7p. The most frequent sites of copy number decreases were 1p32-pter (21%) and 4q (21%). The recurrent chromosomal aberrations identified in this study provide candidate regions involved in the tumorigenesis and progression of ICC.
Cytogenetic analysis plays an important role in the elucidation of the pathogenesis and prognosis of cancers. Several techniques are now available for genome-wide screening of alterations in copy number. Comparative genomic hybridization (CGH) is a molecular cytogenetic tool that allows the screening of copy number change, and has now become one of the most popular genome screening techniques (1). The degenerate oligonucleotide primed polymerase chain reaction (DOP-PCR) CGH technique permits genetic imbalance screening of the entire genome using only small amounts of tumor DNA. It has also been successfully applied to microdissected archival paraffin-embedded tissue by several investigators (2, 3).
Intrahepatic cholangiocarcinoma (ICC) is the second most common malignant tumor in the liver, arising from epithelial cells in the intrahepatic bile duct (4). Although the frequency of ICC is low, its incidence in Southeast Asian is higher than that in Europe and North America. ICC is refractory to conventional chemotherapy and radiation treatment and curative surgical resection is not effective due to the high recurrence rate (5). While risk factors including liver fluke infection, hepatolithiasis, congenital cysts, and sclerosing cholangitis have been reported (6), the genetic mechanisms involved in the development of ICC are not well understood and only a few cytogenetic studies of ICC have been published. Chromosomal gains of 5p, 7p, 8q, 13q, 15q, 17q, and 20q, and losses of 3p, 4q, 6q, 9p, 16q, 17p, and 18q were reported in ICC (7, 8). The structural abnormalities of chromosomes 1, 5, 7, and 12 were detected in ICC cell lines (9). Our previous study demonstrated the chromosomal aberrations in chromosomes 3, 6-8, 12, 14, 17, and 18 were analyzed by cross-species color banding (10). Loss of heterozygosity (LOH) studies of ICC has shown frequent allelic losses from various chromosomes including 1p, 1q, 3p, 6q, 8p, 9q, 14q, 17p, and 18q (11). Whereas specific genes responsible for the development of ICC, genetic alterations of APC, c-erbB2, K-ras, p53, and p16INK4A are proposed to be closely related to ICC progression (12-14).
In this study, the DNAs from 33 Korean ICC specimens were analyzed by DOP-PCR CGH with the aim of identifying the non-random chromosomal aberrations of Korean ICC.
A total 33 formalin-fixed, paraffin-embedded specimens of ICC were collected. Using a 4 µm hematoxylin and eosin stained adjacent section as a guide, tumor-containing areas were microdissected from sectioned 10 µm specimens with a sterile 25-gauge needle under a microscope. Normal DNA from human peripheral blood and tumor DNA from microdissected cells were prepared by standard protocols.
DOP-PCR was performed in two separate steps according to the published protocol with some modifications (15, 16). Briefly, in the preamplification step, the four initial cycles were performed at low stringency conditions (denaturation at 95℃ for 3 min followed by 4 cycles of 94℃ for 1 min; 25℃ for 1 min; 56℃ for 3 min; 74℃ for 2 min), followed by 35 cycles in high stringency conditions (at 94℃ for 1 min; 56℃ for 1 min; 72℃ for 2 min). Labellings of tumor DNA with biotin-16dUTP and control DNA with digoxigenin-11dUTP were performed by nick translation (Nick Translation System, Gibco/BRL, Rockville, MD, U.S.A.). Equal amounts of labeled normal control and tumor DNA including 40 µg of Cot-1 DNA (Gibco/BRL) and 1 µg salmon sperm DNA were precipitated with ethanol. Labeled DNA probes were mixed with hybridization buffer, denatured at 75℃ for 5 min and then applied onto the denatured metaphase slides. After incubation for 3 days, the slides were washed and the hybridization signal was detected using an avidin-fluorescein isothiocyanate (FITC, 1:400) and mouse anti-digoxigenin (1:100). Metaphase slides were counterstained with DAPI at a concentration of 0.1 µg/mL in an antifade solution (Oncor, Gaithersburg, MD, U.S.A.) for chromosomal identification. Mean ratio profiles were determined from analyses of 10-15 metaphase cells. Thresholds for gains and losses were defined as the theoretical value of 1.25 and 0.75, respectively. The following regions were excluded for the analyses; centromere, acrocentric short arms, telomere, and heterochromatin-rich region (17).
Thirty-three intrahepatic cholangiocarcinomas were analyzed using DOP-PCR CGH. The various chromosomal aberrations were detected in all tumors studied. All chromosomes were involved in imbalances and the mean number of imbalances per tumor specimen was 9 (range, 2-18). Table 1 summarizes clinical and chromosomal findings in the 33 entire cases. A schematic summary of copy number changes as detected by CGH analysis in 33 abnormal cases is shown in Fig. 1.
Among the 33 cases, the common sites of copy number increases, in order of frequency, were, 20q (67%), 17 (61%), 11q11-q13 (42%), 8p12-qter (39%), 18p (39%), 15q22-qter (36%), 16p (36%), 3q25-qter (27%), 1q41-qter (24%), and 5p14-q11.2 (24%) (Fig. 1). Sixteen tumors exhibited one or more sites of DNA amplification; the frequent sites of amplification were 20q13.2-qter (6 cases), 17p (3 cases), 17q23-qter (2 cases), and 7p (2 cases). The most frequent sites of copy number decreases were 1p32-pter (21%) and 4q (21%). Examples of these gains and losses are shown in Fig. 2. Gains of 11q11-q13, 17, and 18p were more commonly detected in moderately and poorly differentiated tumors. Gain of 3q25-qter was more frequently observed in moderately differentiated ICC.
The analysis of recurring chromosomal aberrations has become an integral part of the diagnostic and prognostic workup in many human cancers, and their molecular analyses have facilitated the identification of genes related to the pathogenesis of cancers. However, the characterization of complex chromosomal rearrangements was limited when conventional cytogenetic methods were used. In this study thirty-three ICC were successfully analyzed using DOP-PCR CGH.
In consistent with our result, a few alteration such as copy number increases of 5p, 8q, 15q, 17q, and 20q were previously reported in ICC (7, 8). The most common gain region was 20q, with eight tumors showing high amplification of 20q13-qter. Gains of 17q and 20q are common finding in several solid tumors including hepatocellular carcinoma, pancreas, and breast tumors (18-20). Chromosome arm 20q contains many genes such as E2F1 (20q11), AIB1 (20q12), BTAK/STK15 (20q13), and NABC14 (20q13.2), which are involved in several cancers (21-25). Gene amplification and protein expression of c-erbB-2 (HER-2/neu) on 17q21 were observed in ICC and breast cancer (26, 27).
In particular, gains of 3q26-qter, 6p21, 11q11.2-q13, 16p and 18p are frequently observed in this study (Fig. 2A, D, E). Chromosome region 3q26-qter harbors PIK3CA and ECT2 proto-oncogenes that are related to cell proliferation and carcinogenesis (28). The frequent over-represented PIK3CA has also been reported in ovarian, head and neck, and stomach cancers (29-31). Gain of 6p with a minimum overlapping region at 6p21 was frequently observed in this study. Among the genes mapped to 6p21, PIM1 was known as a proto-oncogene related to the progression of thymic lymphomas in transgenic mice, although the role of PIM1 in the other cancers is not elucidated (32). In addition, CCND3 on 6p21 shares considerable homology with proto-oncogene cyclin D1 and is involved in the cell cycle regulation at G1 to S phase transition (33). Gain of 11q13 is a common event in human cancers and is associated to poor prognosis (34, 35). Chromosomal band 11q13 contained several cancer related genes such as FGF3, FGF4, and EMS1, and only CCND1 and EMS1 (36, 37).
Chromosomal loss was found to be less frequent in this study. Losses of 1p and 4q have been reported in ICC (7, 8), in consistent with our current result (Fig. 2B, E). Previous reports indicated that 1p loss is related to the early stage of hepatocarcinogenesis (38) and at least three or more tumor suppressor genes including the p73 gene are localized on 1p 36. The deletion of 1p36 was reported to be related to the progression of ICC without metastatic activity (39). Allelic loss from 4q appears to be a fundamental event in the development of HCC (40).
In conclusion, our CGH demonstrated the complexity of genetic aberrations in ICC. Although chromosomal loss was found to be less frequent in this study, we were able to identify several genetic changes, which were previously reported. These findings suggest that the technical improvement in DOP-PCR-CGH technique may reliably enhance the sensitivity and accuracy of the analysis. The recurrent gains and losses of chromosomal regions identified in this study provide candidate regions containing oncogenes or tumor suppressor genes involved in ICC development and progression.
Figures and Tables
Fig. 1
Summary for gains and losses of chromosomal regions in 33 cholangiocarcinomas. Gains are shown on the right side of the chromosome ideograms and losses on the left. Each line illustrates the affected region of the chromosome in a single tumor sample. Thick lines represent high-level amplifications.
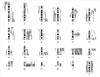
Fig. 2
Examples of the CGH profiles of chromosomal aberrations in ICC. Red and green lines represent thresholds for chromosomal losses (0.75) and gains (1.25), respectively. (A) Gain of 3q26-qter was detected, (B) Deletion of 4p15-qter was observed, (C) Overrepresentation of 8q22-qter with high amplification of 8q24 was found, (D) Gain of 11p11-q13 was detected, (E) Gain of 18p was observed, (F) Gain of 20q was found. *n=number of chromosomes evaluated.
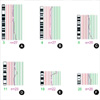
ACKNOWLEDGEMENTS
This work was supported by a grant from Medical Science Research Center Korea University, the Brain Korea 21 Project, and a Grant R04-2000-000-00047-0 from the Basic Research Program of the Korea Science and Engineering Foundation.
References
1. Forozan F, Karhu R, Kononen J, Kallioniemi A, Kallioniemi OP. Genome screening by comparative genomic hybridization. Trends Genet. 1997. 13:405–409.


2. Telenius H, Carter NP, Bebb CE, Nordenskjold M, Ponder BA, Tunnacliffe A. Degenerate oligonucleotide-primed PCR: general amplification of target DNA by a single degenerate primer. Genomics. 1992. 13:718–725.


3. Huang Q, Schantz SP, Rao PH, Mo J, McCormick SA, Chaganti RS. Improving degenerate oligonucleotide primed PCR-comparative genomic hybridization for analysis of DNA copy number changes in tumors. Genes Chromosomes Cancer. 2000. 28:395–403.


4. Kim YI, Park CK, Kim JR, Chang JJ. Primary malignant epithelial neoplasms of the liver. J Korea Cancer Assoc. 1980. 12:33–53.
5. Pederson LC, Buchsbaum DJ, Vickers SM, Kancharla SR, Mayo MS, Curiel DT, Stackhouse MA. Molecular chemotherapy combined with radiation therapy enhances killing of cholangiocarcinoma cells in vitro and in vivo. Cancer Res. 1997. 57:4325–4332.
6. Holzinger F, Z'graggen K, Buchler MW. Mechanisms of biliary carcinogenesis: a pathogenetic multi-stage cascade towards cholangiocarcinoma. Ann Oncol. 1999. 10:Suppl 4. 122–126.


7. Koo SH, Ihm CH, Kwon KC, Park JW, Kim JM, Kong G. Genetic alterations in hepatocellular carcinoma and intrahepatic cholangiocarcinoma. Cancer Genet Cytogenet. 2001. 130:22–28.


8. Wong N, Li L, Tsang K, Lai PB, To KF, Johnson PJ. Frequent loss of chromosome 3p and hypermethylation of RASSF1A in cholangiocarcinoma. J Hepatol. 2002. 37:633–639.


9. Storto PD, Saidman SL, Demetris AJ, Letessier E, Whiteside TL, Gollin SM. Chromosomal breakpoint in cholangiocarcinoma cell line. Genes Chromosomes Cancer. 1990. 2:300–310.
10. Kim DG, Park SY, You KR, Lee GB, Kim H, Moon WS, Chun YH, Park SH. Establishment and characterization of chromosomal aberrations in human cholangiocarcinoma cell lines by cross-species color banding. Genes Chromosomes Cancer. 2001. 30:48–56.


11. Kang YK, Kim YI, Kim WH. Allelotype analysis of intrahepatic cholangiocarcinoma. Mod Pathol. 2000. 13:627–631.


12. Kang YK, Kim WH, Lee HW, Lee HK, Kim YI. Mutation of p53 and K-ras, and loss of heterozygosity of APC in intrahepatic cholangiocarcinoma. Lab Invest. 1999. 79:477–483.
13. Terada T, Ashida K, Endo K, Horie S, Maeta H, Matsunaga Y, Takashima K, Ohta T, Kitamura Y. C-erbB-2 protein is expressed in hepatolithiasis and cholangiocarcinoma. Histopathology. 1998. 33:325–331.


14. Tannapfel A, Benicke M, Katalinic A, Uhlmann D, Kockerling F, Hauss J, Wittekind C. Frequency of p16INK4A alterations and k-ras mutations in intrahepatic cholangiocarcinoma of the liver. Gut. 2000. 47:721–727.
15. Kuukasjarvi T, Tanner M, Pennanen S, Karhu R, Visakorpi T, Isola J. Optimizing DOP-PCR for universal amplification of small DNA samples in comparative genomic hybridization. Genes Chromosomes Cancer. 1997. 18:94–101.
16. Kim GJ, Cho SJ, Won NH, Sung JM, Kim H, Chun YH, Park SH. Genomic imbalances in Korean hepatocellular carcinoma. Cancer Genet Cytogenet. 2003. 142:129–133.


17. el-Rifai W, Larramendy ML, Bjorkqvist AM, Hemmer S, Knuutila S. Optimazation of comparative genomic hybridization using fluorochrome conjugated to dCTP and dUTP nucleotides. Lab Invest. 1997. 77:699–700.
18. Mahlamaki EH, Hoglund M, Gorunova L, Karhu R, Dawiskiba S, Andren-Sandberg A, Kallioniemi OP, Johansson B. Comparative genomic hybridization reveals frequent gains of 20q, 8q, 11q, 12p and 17q and losses of 18q, 9p, and 15q in pancreatic cancer. Genes Chromosomes Cancer. 1997. 20:383–391.
19. Fukushige S, Waldman FM, Kimura M, Abe T, Furukawa T, Sunamura M, Kobari M, Horii A. Frequent gain of copy number on the long arm of chromosome 20 in human pancreatic adenocarcinoma. Genes Chromosomes Cancer. 1997. 19:161–169.


20. Tirkkonen M, Tanner M, Karhu R, Kallioniemi A, Isola J, Kallioniemi OP. Molecular cytogenetics of primary breast cancer by CGH. Genes Chromosomes Cancer. 1998. 21:177–184.


21. Saito M, Helin K, Valentine MB, Griffith BB, Willman CL, Harlow E, Look AT. Amplification of the E2F1 transcription factor gene in the HEL erythroleukemia cell line. Genomics. 1995. 25:130–138.


22. Anzick SL, Kononen J, Walker RL, Azorsa DO, Tanner MM, Guan XY, Sauter G, Kallioniemi OP, Trent JM, Meltzer PS. AIB1, a steroid receptor coactivator amplified in breast and ovarian cancer. Science. 1997. 277:965–968.


23. Collins C, Rommens JM, Kowbel D, Godfrey T, Tanner M, Hwang SI, Polikoff D, Nonet G, Cochran J, Myambo K, Jay KE, Froula J, Cloutier T, Kuo WL, Yaswen P, Dairkee S, Giovanola J, Hutchinson GB, Isola J, Kallioniemi OP, Palazzolo M, Martin C, Ericsson C, Pinkel D, Gray JW, Albertson D, Li WB. Positional cloning of ZNF217 and NABC1: genes amplified at 20q13.2 and overexpressed in breast carcinoma. Proc Natl Acad Sci USA. 1998. 95:8703–8708.


24. Ghadima BM, Schrock E, Walker RL, Wangsa D, Jauho A, Meltzer PS, Ried T. Specific chromosomal aberrations and amplification of the AIB1 nuclear receptor coactivator gene in pancreatic carcinomas. Am J Pathol. 1999. 154:525–536.
25. Zhou H, Kuang J, Zhong L, Kuo WL, Gray JW, Sahin A, Brinkley BR, Sen S. Tumour amplified kinase STK15/BTAK induces centrosome amplification, aneuploidy and transformation. Nat Genet. 1998. 20:189–193.


26. Ukita Y, Kato M, Terada T. Gene amplification and mRNA and protein overexpression of c-erbB-2 (HER-2/neu) in human intrahepatic cholangiocarcinoma as detected by fluorescence in situ hybridization, in situ hybridization, and immunohistochemistry. J Hepatol. 2002. 36:780–785.


27. Meenakshi A, Kumar RS, Siva Kumar N, Genesh V. Immunofluorescent localization of C-erbB-2 oncoprotein in breast cancer: a preliminary study. Hum Antibodies. 2002. 11:73–77.


28. Takai S, Long JE, Yamada K, Miki T. Chromosomal localization of the human ECT2 proto-oncogene to 3q26.1→q26.2 by somatic cell analysis and fluorescence in situ hybridization. Genomics. 1995. 27:220–222.
29. Shayesteh L, Lu Y, Kuo WL, Baldocchi R, Godfrey T, Collins C, Pinkel D, Powell B, Mills GB, Gray JW. PIK3CA is implicated as an oncogene in ovarian cancer. Nature Genet. 1999. 21:99–102.


30. Byun DS, Cho K, Ryu BK, Lee MG, Park JI, Chae KS, Kim HJ, Chi SG. Frequent monoallelic deletion of PTEN and its reciprocal association with PIK3CA amplification in gastric carcinoma. Int J Cancer. 2003. 1004:318–327.
31. Redon R, Muller D, Caulee K, Wanherdrick K, Abecassis J, du Manoir S. A simple specific pattern of chromosomal aberrations at early stages of head and neck squamous cell carcinomas: PIK3CA but not p63 gene as a likely target of 3q26-qter gains. Cancer Res. 2001. 61:4122–4129.
32. Feldman BJ, Reid TR, Cleary ML. Pim1 cooperates with E2a-Pbx1 to facilitate the progression of thymic lymphomas in transgenic mice. Oncogene. 1997. 15:2735–2742.


33. Inaba T, Matsushime H, Valentine M, Roussel MF, Sherr CJ, Look AT. Genomic organization, chromosomal localization, and independent expression of human cyclin D genes. Genomics. 1992. 13:565–574.


34. Akervall JA, Jin Y, Wennerberg JP, Zatterstrom UK, Kjellen E, Mertens F, Willen R, Mandahl N, Heim S, Mitelman F. Chromosomal abnormalities involving 11q13 are associated with poor prognosis in patients with squamous cell carcinoma of the head and neck. Cancer. 1995. 76:853–859.


36. Dickson C, Fantl V, Gillett C, Brookes S, Bartek J, Smith R, Fisher C, Barnes D, Peters G. Amplification of chromosome band 11q13 and a role for cyclin D1 in human breast cancer. Cancer Lett. 1995. 90:43–50.


37. Schuuring E. The involvement of the chromosome 11q13 region in human malignancies: cyclin D1 and EMS1 are two new candidate oncogenes-a review. Gene. 1995. 159:83–96.


38. Piao Z, Park C, Park JH, Kim H. Allelotype analysis of hepatocellular carcinoma. Int J Cancer. 1998. 75:29–33.


39. Momoi H, Okabe H, Kamikawa T, Satoh S, Ikai I, Yamamoto M, Nakagawara A, Shimahara Y, Yamaoka Y, Fukumoto M. Comprehensive allelotyping of human intrahepatic cholangiocarcinoma. Clin Cancer Res. 2001. 7:2648–2655.