Abstract
We evaluated DNA protection effect of heat shock protein (HSP) against cytotoxic effects of exogenous nitric oxide (NO) and reactive oxygen intermediate (ROI). Cultured human corneal fibroblasts were divided into 4 groups. Control (Group I) was not exposed to a sub-lethal heat treatment. Other 3 groups were exposed to 43℃ for 1 hr, then incubated at 37℃ during different duration (1, 6, 24 hr, Group II, III, IV, respectively). Expression pattern of HSP 70 was analyzed by Western blot. Cell viability was measured by MTT assay and the relationship between HSP 70 expression and DNA damage was examined by terminal deoxyribonucleotidyl transferase mediated dUTP-digoxigenin nick and labeling (TUNEL) stain and single cell gel electrophoresis. Expression pattern of HSP 70 was dependent on recovery times. Cell viability following heat treatment was significantly increased and the TUNEL positive cell number was decreased at 6 hr. In single cell gel electrophoresis, tail moments were increased in a dose-dependent manner by SNAP and X/XO. Following heat treatment, tail moments showed decreased significantly at 6 hr. These results suggest that induction of HSP 70 by sub-lethal heat treatment is closely related with cytoprotective effects against oxidative stresses in human corneal fibroblasts.
Heat shock causes the intracellular expression of a specific group of proteins called heat shock proteins (HSPs) that have broad cytoprotective properties. The first demonstration of HSP-mediated cytoprotection involved the phenomenon of thermotolerance, whereby a brief heat shock conferred protection. Subsequent studies demonstrated that the induction of HSPs also protected cells and whole organs against nonthermal cytotoxic agents such as oxidants, tumor necrosis factor-α, and endotoxin (1). Oxidative stresses such as nitric oxide (NO) and reactive oxygen intermediates (ROI) are known to be major mediators of cell damages during inflammation (2, 3).
HSPs can be divided into six subfamilies according to their molecular weights: the large HSPs of 100-110 kDa, the HSP 90 family, the HSP 70 family, the HSP 60 family, the HSP 40 family, and the small HSPs of 18-30 kDa (4). The HSP 70 family is the most conserved and best-studied family. Human cells contain several HSP 70 family members including highly stress-inducible HSP 70 (also known as HSP 72 or HSP 70i), constitutively expressed heat shock cognate protein HSC 70 (HSP 73), a mitochondrial HSP 75 (mtHSP 75) and Grp78 (BiP) localized in the endoplasmic reticulum (5, 6). Under normal conditions, HSP 70 proteins function as ATP-dependant molecular chaperones, by which assist in the folding of newly synthesized polypeptides, the assembly of multiprotein complexes and the the transport of proteins across cellular membranes. Under various stress conditions, the synthesis of stress-inducible HSP 70 enhances the ability of stressed cells to cope with increased concentrations of unfolded and/or denatured proteins. Moreover, HSP 70 can effectively inhibit cellular death processes, apoptosis or necrosis, and thereby increase the survival of cells exposed to a wide range of lethal stimuli (2). The mechanisms of HSPs confer protection from NO and ROI have not been completely understood.
DNA single strand breaks can be used as a biomarker of oxidant exposure and cell damages. The single cell gel electrophoresis (SCGE) assay, known as comet assay, is reported to be a simple and sensitive assay for DNA single strand breaks in many types of cells (7). Compared to traditional assays for single strand breaks such as the alkaline elution assay, or gradient centrifugation assay, the SCGE assay requires a smaller number of cells, is less time-consuming, does not use radioactive materials, and can be more sensitive than traditional methods for analyzing DNA single strand breaks (8). However, the SCGE assay has rarely been used in ophthalmologic studies.
We purposed to examine the pattern of HSP 70 expression induced by sub-lethal heat treatment in order to determine the major heat shock protein and its protective effects against DNA damage caused by NO and ROI. This was done using Western blot, RT-PCR, MTT assay, terminal deoxyribonucleotidyl transferase mediated dUTP-digoxigenin nick and labeling (TUNEL) assay and SCGE.
Human corneal fibroblasts were prepared from remnant donor eye tissue for penetrating keratoplasty within 8 hr post mortem. The cells were grown and maintained in Dulbecco's minimum essential medium-F12 (DMEM-F12, Gibco, NY, U.S.A.) containing 10% fetal bovine serum (FBS, Gibco, NY, U.S.A.), sodium bicarbonate (Sigma, St. Louis, MO, U.S.A.), penicillin and streptomycin of 100 units/mL (Gibco, NY, U.S.A.). The cultures were maintained in a 5% CO2 in air, humidified atmosphere at 37℃ incubator. Approximately 3×105 cells were plated in 6-well plates respectively.
The culture medium was changed to a serum-free medium pre-warmed to 37℃. The cells were divided into four groups. Group I was the control group, therefore it was not exposed to sub-lethal heat treatment. The cells of the other groups were transferred to an incubator and maintained at 43℃ for 1 hr. For recovery following stress, the cells were returned to a 37℃ incubator and allowed to recover for 1, 6 or 24 hr (Group II, III, and IV, respectively).
Following subsequent treatment, 10 µg of cell lysate (standardized by Bradford method) was dissolved into a gel sample buffer containing 0.63 M Tris-HCl pH 6.8, 8 M urea, 1% sodium dodecyl sulfate (SDS), 10% glycerol and 1% β-mercaptoethanol. 35 µL of each preparation was resolved with 10% SDS and electrophoretically transferred to a nitrocellulose membrane and processed for Western blot analysis. The monoclonal antibody to HSP 70 (StressGen Biotechnologies Co., Victoria, Canada) was diluted 1:1,000 in Trisbuffered saline (TBS) containing 1 mg/mL bovine serum albumin. Horseradish peroxidase-conjugated anti-mouse IgG (Bio-Rad, CA, U.S.A.) was used as the secondary antibody. Immunoreactive proteins were visualized as exposed bands on radiography film using the Immune-star chemiluminescent protein detection system (Pierce, Illinois, U.S.A.). Densitometric analysis was done by using the Image gauge 3.11 program in the Japanese FUJIFILM BAS-2500 system.
After each recovery time of 1, 3, and 6 hr in the reverse transcription polymerase chain reaction (RT-PCR) experiments, product #STM-507 (HSP 70B prime gene) by Stress-Gen, and modified protocol were used. 5 µL of synthesized cDNA, 5 µL of 10× polymerase buffer, 0.5 µL of Taq DNA polymerase, 2 µL of 10 mM dNTPs, 2 µL of HSP 70 sense primer, 33.5 µL of DEPC-DW and the primers necessary to amplify HSP 70 cDNA, 2 µL of HSP 70 sense primer (5'-CTCCAGCATCCGACAAGAAGC-3', 20 pmol), and 2 µL HSP 70 antisense primer (5'-ACGGTGTTGTGGGGGTTCAGG-3', 20 pmol) were added to make the total volume of 50 µL. After adding 40 µL of mineral oil, the reaction mixture was denatured at 95℃ for 3 min, put under 35 denaturing cycles at 94℃ for 1 min, annealed at 58℃ for 1 min elongated at 72℃ for 1 min, and lastly extended for 10 min at 72℃. At the same time, to compare HSP 70 cDNA amplification conditions, amplification was performed using a sense primer (5'-TGAAGGTCGGAGTCAACGGATTTCCGT-3') of G3PDH (glyceraldehyde-3-phosphatedehydrogenase) and antisense primer (5'-CATGTGGGCCATGAGGTCCAC-3'). After making 1.5 mL of agarose gel by placing 1.5 g of agarose powder per 100 cc of 1× TAE buffer and 50 µL of ethidium bromide, a 2 µL of sample buffer was created and electrophoresis was performed on the RT-PCR product at 100 V for 15 min.
S-nitroso-N-acetyl-D,L-penicillamine (SNAP) and xanthine/xanthine oxidase (X/XO) (Sigma, St. Louis, U.S.A.) were dissolved in phosphate buffered saline (Sigma, St. Louis, U.S.A.) and stored at 4℃. 15% cell growth inhibition concentration (IC15 value), which was determined using MTT assay in a preliminary toxic test, was considered an intermediate concentration. We set up 1/2×IC15, IC15, and 2×IC15, as experimental concentrations. After placing 2,500 cells in each well in a 96-well tissue culture plate, the plate was placed in a 43℃ incubator for 1 hr, and after each recovery time of 1, 6 and 24 hr, a 37℃, 5% CO2 incubator. Each well was treated with SNAP (no treatment, 1 mM, 2 mM, 4 mM, respectively) and X/XO (no treatment, 0.005 mM/0.005 units, 0.01 mM/0.01 units, 0.02 mM/0.02 units, respectively). After 24 hr, 10 µL of MTT (3-[4,5-dimethylthiazol-2-yl]-2,5-diphenyltetrazolium bromide) was added to each well and incubated at 37℃ at 5% CO2 for 6 more hours. 90 µL of 10% SDS was added to the mixture to stop the reaction. Optical density (OD) was measured at 540 nm with a spectrophotometer (ELISA reader) for each 96-well plate. The measured OD was expressed in percent, determined cell survival rate in comparison to the control cell group, and calculated the statistical difference in the cell survival rate with one-way ANOVA. To avoid an induction of unwanted heat treatment response, media and phosphate buffered saline (PBS) were equilibrated at 37℃ before use.
In order to determine the effects of HSP expression on corneal fibroblasts, cultured corneal fibroblasts were treated with heat shock with the above method. After a certain period of recovery (1, 6, 24 hr), the cells were treated with SNAP 1 mM or X/XO 0.1/0.01 mM and the medium was discarded. Then, the cells were treated with trypsin and mixed with the medium again before being centrifuged at 4℃ for 5 min. After being centrifuged, the supernatant was discarded. The silanized microscope slide was placed on a cytospin, centrifuged at 500 rpm at room temperature for 5 min and dried. After washing the slide with a 0.05 M phosphate buffer solution for three times, the cells were treated in a balanced buffer solution for 15 min at room temperature, and under the presence of 11-digoxigenin dUTP, kept at 37℃ in TdT for 90 min. After placing the cells in a stop/wash buffer at 37℃ for 30 min, the cells were placed in bovine fetal serum to stop the immune reaction for 10 min, and again placed in anti-digoxigenin-peroxide for 30 min. After being stained with 2.24 mM diamonobenzidine, the cells were counterstained with hematoxylin and observed with an optical microscope. Under the low magnification of 100×, the number of positively stained cells was counted 5 times and the averaged. The results were compared with those from the control cells with no heat shock. ANOVA (analysis of variance) was performed.
Cells were collected by centrifuge at 1,000 rpm for 5 min, and the pellet was suspended in 50 µL 0.5% low-melting temperature agarose (type VII; Sigma) in phosphate buffered saline at 37℃. The cell mixture was pipetted onto a frosted glass slide precoated with a basal layer of 0.5% normal agarose (type III-a; Sigma) to promote cell adhesion. A coverslip was placed over the slide, and it was kept at 4℃ for 5 min. Once the coverslip was removed, an additional 200 µL 0.5% normal agarose was pipetted onto the cells. The slide was once again covered and kept at 4℃ for 5 min. After removing the coverslip, the slide was incubated in the dark at 4℃ and for 2 hr with a lysis buffer (2.5 M NaCl, 100 mM Na2EDTA, 10 mM Tris, pH 10, 1% Triton X-100; Sigma). The slide was soaked in an electrophoresis buffer (300 mM NaOH, 10 mM Na2EDTA) for 20 min. The buffer covered the slide to a 2-mm depth, and the slide was subjected to electrophoresis for 20 min at 12 V, 250 mA. The slide was gently washed with 3 changes of 0.4 M Tris (hydroxymethyl) aminomethane for 5 min to remove any excess alkali and was stained with 150 µL of ethidium bromide (2 µg/mL). The slide was then examined with a fluorescent microscope with an excitation filter of 515-560 nm and a barrier filter of 590 nm. This assay utilizes the principle that DNA containing more breaks will migrate a greater distance in an electrophoretic field due to a smaller mass. Tail moments (Fig. 1) were measured from a total of 50 cells per slide and two slides were viewed per sample using image analyzer software (Komet 3.1, Kinetic Imaging Co., Liverpool, U.K.). Descriptive data was expressed through means and standard deviation (mean±standard deviation). ANOVA (analysis of variance) was performed.
Fig. 2 demonstrates that subjecting cells to sub-lethal heat treatment leads to the rapid expression of HSP 70. The expression patterns of the control group and the heat-treated groups were as follows: A remarkable increase in the expression of HSP 70 (70.5 kDa) was observed after recovery from the sublethal heat shock. A slight expression of HSP 70 was revealed in group I (640 psl, in the Image Analyser 3.11 program), then increased substantially (group II, 34,660 psl), was expressed at maximum levels at 6 hr (group III, 62,370 psl), and persisted for 24 hr (group IV, 41,520 psl). These data demonstrate that heat shock can induce HSP 70 in human corneal fibroblasts prominently.
In RT-PCR, the expression of HSP 70 mRNA was not observed in the control group, but was slowly expressed after 1 hr of recovery from the sub-lethal heat treatment, reached a maximum level at 3 hr (prior to the elevation of protein level), and started to decrease following 6 hr of recovery time (Fig. 3). Protein synthesis was occurred after mRNA transcription, thus it may considered that maximum expression of HSP 70 mRNA transcription was showen at 3 hr.
Fig. 4 demonstrates that the treatment with NO and ROI decreased the viability of human corneal fibroblasts in a concentration dependant manner. Additionally, the cell viability, which was treated by NO and ROI following sub-lethal heat treatment, increased significantly in comparison to group I (control group), particularly after 6 hr of recovery time (group III, p<0.05).
Compared with the control group, the number of TUNEL positive corneal fibroblasts was significantly decreased at 6 hr after heat shock (p<0.05) (Table 1).
While the "comet tail" of fluorescent strands of DNA seen outside the cell is the characteristic feature of single strand DNA breaks, four other patterns of DNA distribution were seen using fluorescent microscopy (Fig. 5). These included a central core of DNA, a peripheral aggregation or clumping of DNA, a comet tail of DNA strands outside the cell, and diffusely dispersed DNA tending toward one pole of the nucleus. In the group not treated with SNAP or X/XO, the central core and peripheral pattern of DNA was dominant. When the concentration of SNAP or X/XO was increased, the length of the comet tail was elongated. And the pattern outside the cell and of diffusely dispersed DNA was more dominant (data not shown).
The fraction of DNA in the tail (DNA content in tail/DNA content in nucleus+tail) multiplied by the tail length is defined as the comet "tail moment" and is considered one of the most exact measures of DNA breakage making use of this assay. Accordingly, the mean (±standard deviation) tail moment was used to compare the data obtained from the different treatments (Fig. 6). The tail moments of cells without treatment of SNAP or X/XO in each group showed no significant difference (N). The tail moment increased in a dose-dependent manner in the SNAP and X/XO treated groups. However, the tail moments of group II, III, and IV showed a decrease as compared to group I. An especially significant decrease (p<0.05) in tail moment was observed in group III (6 hr of recovery time after sub-lethal heat treatment) (Fig. 6).
Stresses such as heat, ischemia, inflammation, exposure to oxidizing agents and radiation can induce different cellular responses depending on their strength. According to the strength, stresses can induce a protective heat shock response, apoptosis or necrosis. The heat shock, or stress response, is the synthesis of HSPs in response to a mild stress, which allows cells to adapt to gradual changes in their environment and to survive in otherwise lethal conditions (2, 9). It may well be that the "beneficial effects of fever" already described by Hippocrates actually relate to increased HSP expression during fever, and to their protective effects (10).
The first report on stress response dates back to 1962, when Ritossa discovered a novel heat-inducible 'puffing' pattern in fruit fly salivary gland chromosomes (11). The heat-induced puffing of the chromosomes, indicative of specific gene activity, was found to be associated with an increase in the synthesis of proteins with molecular masses of 70 kDa (12).
Conveniently, the constitutive and inducible form of the HSP 70 family can be well distinguished immunologically. The constitutive forms are expressed in unstressed cells, and the inducible forms are expressed only in stressed cells. Synthesis of both forms increases after hyperthermia (13, 14). The major heat shock protein HSP 70 can be synthesized in a wide variety of cells such as rat islet cell, cardiomyocyte or cultured hepatocyte in response to heat or cold treatment. It is known that the degree of HSP 70 induction varies according to recovery time after heat shock (15-17). A major up-regulation in the transcription rate of HSP 70 was observed between 0.75 and 1.50 hr after hyperthermia in the cerebellum and the retina of rabbits. Gel-mobility shift assays reveal that the conversion time of a heat-shock transcription factor (HSF 1) to a DNA-binding form parallel the transcriptional induction profile of HSP 70 (18).
In our other experiments, the expression patterns of HSP 27, 47, 70 and 90 were examined by Western blot under the same conditions of this experiment and the major protective protein, HSP 70, was detected. In this experiment, HSP 70 increased significantly after 1 hr and showed maximum expression after 6 hr. When SNAP or X/XO were treated, cell viability was observed to increase in comparison to the control group. As a result, the experiment suggests that HSP 70 is expressed by heat shock and plays an important role in protecting cells from stresses experienced in human corneal fibro-blasts.
The mechanisms by which HSPs confer protection from NO and ROI are not completely understood, but recent studies suggest several possible mechanisms. Both NO and ROI are known to induce DNA damage in islet cells which results in activation of the DNA repair enzyme poly (ADP-ribose) polymerase (PARP). The protective heat shock response involves down-regulation of PARP activity, which prevents NO-mediated decreases in cellular ATP levels because of NAD+ depletion in radical-damaged cells (19).
When TUNEL positive corneal fibroblasts were measured following treatment with SNAP or X/XO, it was observed that the number decreased significantly beginning 6 hr after heat shock. Additionally, the fact that the tail moment, which equates to DNA damage, was significantly decreased 6 hr after heat shock in SCGE, supports the proposition that expressed HSP 70 prevents apoptosis or DNA damage and is ultimately related to the inhibition of cell death.
In all cases of corneal surgery, the change in the corneal stromal environment, including the NO produced by inflammatory cells, the ROS, or cytokines, create cytotoxic stresses, which result in cell death and eventually contribute to the production of corneal opacity (20-22). Research shows that if heat shock is induced 6 hr in advance to maximize the expression of HSP 70, cellular loss caused by apoptosis or necrosis as well as corneal fibroblast loss caused due to cytotoxic stresses from inflammatory cells decreases in all types of ocular surface diseases with inflammation.
Additionally, our results demonstrate that SCGE (comet) assay is clear and sensitive, and its application to the determination of DNA damage in ophthalmology is relatively new (8). According to other authors (23-25), the advantages of this technique include simplicity, cost effective, a small cell number requirement (as any eukaryotic cell population can be used), the ability to assess DNA damage in individual cells and the ability to assess distribution thus providing detailed pattern of damage. Clearly, this technique has many possible applications. We applied the thermal treatment for induction of HSP before photo refractive keratectomy (PRK) and have a result after procedure. Some reports support our clinical result of thermal therapy before PRK (27, 28). Corneal wound healing was completed without any problems such as corneal opacity. This heating was very effective preoperative treatment before any operation expected corneal damage and would be extended to treatment for any inflammatory corneal disease.
Figures and Tables
Fig. 1
The definition of parameter of single cell gel electrophoresis (SCGE) in Komet 3.0 image analyzer. The fraction of DNA in the tail (DNA content in tail/DNA content in nucleous+tail) multiplied by the tail length is defined as the comet "tail moment" and it is considered one of the most exact measures of DNA breakage making use of this assay.
A+B, DNA migration; Tail moment, tail distance×% DNA in tail; Tail distance, center position of tail-center position of head.

Fig. 2
Western blot analysis of HSP 70. It is time course of HSP 70 expression in human corneal fibroblasts after sublethal heat treatment (43℃ for 1 hr). I represents control cells incubated at 37℃ and without heat treatment, and II, III, IV represent 1 hr, 6 hr, and 24 hr after sublethal heat treatment, respectively. A little expression of HSP 70 are revealed in group I, then substantially increased (II), expressed maximally at 6 hr (III) and persisted for 24 hr (IV). The gel shown is representative of three separate experiments.
I: control. incubated at 37℃ (without sublethal heat treatment).
II: recover for 1 hr after sublethal heat treatment.
III: recover for 6 hr after sublethal heat treatment.
IV: recover for 24 hr after sublethal heat treatment.
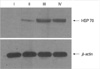
Fig. 3
Time course of HSP 70 mRNA induction in sublethal heat treated human corneal fibroblasts. HSP 70 mRNA transcription is expressed maximum at 3 hr. Protein synthesis occurred after mRNA transcription, thus it may considered that maximum expression of HSP 70 mRNA transcription was preceeded at 3 hr. The position of molecular size (bp) markers is indicated on the left.
Lane 1: incubated at 37℃ without sublethal heat treatment.
Lane 2: recover for 1 hr after sublethal heat treatment.
Lane 3: recover for 3 hr after sublethal heat treatment.
Lane 4: recover for 6 hr after sublethal heat treatment.

Fig. 4
The viability (MTT assay) of human corneal fibroblasts by SNAP (A) or X/XO (B) following recovery times after sublethal heat treatment. Treatment of NO and ROI decreased viability in a concentration dependant manner. And cell viability which were treated by NO and ROI after sublethal heat treatment was significantly increased, especially at 6 hr of recovery time.
*: significantly different from those of control (p<0.05).
group I: control. incubated at 37℃ (without sublethal heat treatment). group II: recover for 1 hr after sublethal heat treatment. group III: recover for 6 hr after sublethal heat treatment. group IV: recover for 24 hr after sublethal heat treatment. L: treated with 1 mM of SNAP or 0.005 mM/0.005 units of X/XO. M: treated with 2 mM of SNAP or 0.01 mM/0.01 units of X/XO. H: treated with 4 mM of SNAP or 0.02 mM/0.02 units of X/XO.

Fig. 5
Fluorescent photomicrographs of human corneal fibroblasts in SCGE (comet) assay after SNAP or X/XO treatment (×1,600). While the "comet tail" of fluorescent strands of DNA seen outside the cell is the characteristic feature of single strand DNA breaks, four other patterns of DNA distribution are seen. These are a central core of DNA (A), peripheral aggregation or clumping of DNA (B), a comet tail of DNA strands outside the cell (C), and diffusely dispersed DNA tending toward one pole of the nucleus (D). In the control group, central core and peripheral pattern of DNA were dominant. When the concentration of SNAP or X/XO was increased, length of comet tails was elongated and the patterns of outside the cell and diffusely dispersed DNA were dominant.

Fig. 6
Histogram of the tail moments in human corneal fibroblasts induced by SNAP (A) and X/XO (B). The tail moment induced in human corneal fibroblasts by SNAP and X/XO increases dose-dependenthy. The tail moment which treated by NO or ROI after sublethal heat treatment was significantly decreased, especially at 6 hr (*p<0.05). *: significantly different from those of control (p<0.05). (A) SNAP (nitric oxide donor; S-nitroso-N-acetyl-D,L-penicillamine). (B) X/XO (reactive oxygen intermediate donor; xanthine/xanthine oxidase).
group I: control.incubated at 37℃ (without sublethal heat treatment). group II: recover for 1 hr after sublethal heat treatment. group III: recover for 6 hr after sublethal heat treatment. group IV: recover for 24 hr after sublethal heat treatment. N: no treatment with SNAP or X/XO. L: treated with 1 mM of SNAP or 0.005 mM/0.005 units of X/XO. M: treated with 2 mM of SNAP or 0.01 mM/0.01 units of X/XO. H: treated with 4 mM of SNAP or 0.02 mM/0.02 units of X/O.
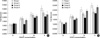
References
1. Wong HR, Ryan M, Menendez IY, Denenberg A, Wispe JR. Heat shock protein induction protects human respiratory epithelium against nitric oxide-mediated cytotoxicity. Shock. 1997. 8:213–218.


2. Jäättela M. Heat shock proteins as cellular lifeguards. Ann Med. 1999. 31:261–271.
3. Wong HR, Mannix RJ, Rusnak JM, Boota A, Zar H, Watkins SC, Lazo JS, Pitt BR. The heat shock response attenuates lipopolysaccharide-mediate apoptosis in cultured sheep pulmonary artery endothelial cells. Am J Respir Cell Mol Biol. 1996. 15:745–751.
4. Lindquist S, Craig EA. The heat shock proteins. Annu Rev Genet. 1988. 22:631–677.
5. Kiang JG, Tsokos GC. Heat shock protein 70kDA: molecular biology, biochemistry, and physiology. Pharmacol Ther. 1998. 80:183–201.
6. Tavaria M, Gabriele T, Kola I, Anderson RL. A hitchhiker's guide to human Hsp 70 family. Cell Stress Chaperones. 1996. 1:23–28.
7. Hughes CM, McKelvey-Martin VJ, Lewis SEM. Human sperm DNA integrity assessed by the Comet and ELISA assays. Mutagenesis. 1999. 14:71–75.
8. Lee JG, Madden MC, Reed W, Adler K, Devlin R. The use of the single cell gel electrophoresis assay in detecting DNA single strand breaks in lung cells in vitro. Toxicol Appl Pharmacol. 1996. 141:195–204.
9. Berra A, Dutt JE, Nouri M, Foster CS. Heat shock protein expression in human conjunctiva. Invest Ophthalmol Vis Sci. 1994. 35:352–357.
10. Polla BS, Cossarizza A. Feige U, Morimoto RI, Yahara I, Polla BS, editors. Stress proteins in inflammation. Stress-inducible cellular responses. 1996. 77. Basel: Birkhauser Verlag;375–391.


11. Ritossa F. A new puffing pattern induced by temperature shock and DNP in Drosophila. Experimentia. 1962. 18:571–573.


12. Tissieres A, Mitchell HK, Tracy UM. Protein synthesis in salivary glands of Drosophila melanogaster: Relation to chromosome puffs. J Mol Biol. 1974. 84:389–398.
13. Jakob U, Buchner J. Assisting spontaneity: the role of HSP 90 and small HSP as molecular chaperones. Trends Biochem Sci. 1994. 19:205–211.
14. Muchowski PJ, Valdez MM, Clark JI. AlphaB-crystallin selectively targets intermediate filament proteins during thermal stress. Invest Ophthalmol Vis Sci. 1999. 40:951–958.
15. Bellmann K, Wenz A, Radons J, Burkart V, Kleemann R, Kolb H. Heat shock induces resistance in rat pancreatic islet cells against nitric oxide, oxygen radicals and streptozotocin toxicity in vitro. J Clin Invest. 1995. 95:2840–2845.


16. Laios E, Rebeyka IM, Prody CA. Characterization of cold-induced heat shock protein expression in neonatal rat cardiomyocytes. Mol Cell Biochem. 1997. 173:153–159.
17. Kim YM, de Vera ME, Watkins SC, Billar TR. Nitric oxide protects cultured rat hepatocytes from tumor necrosis factor-α-induced apoptosis by inducing heat shock protein 70 expression. J Biol Chem. 1997. 272:1402–1411.


18. D'Souza CA, Rush SJ, Brown IR. Effect of hyperthermia on the transcription rate of heat-shock genes in the rabbit cerebellum and retina assayed by nuclear run-ons. J Neurosci Res. 1998. 52:538–548.
19. Bellmann K, Jäättela M, Wissing D, Burkart V, Kolb H. Heat shock protein HSP 70 overexpression confers resistance against nitric oxide. FEBS Lett. 1996. 391:185–188.
20. Han JA, Kwak IH, Kim JC. Ultrastructural changes of corneal edema in endotoxin-induced uveitis model. J Korean Ophthalmol Soc. 1999. 40:2103–2110.
21. Joo MJ, Lee KJ, Shin KH, Kim JC. Nitric oxide-mediated neurotoxicity after excimer laser photorefractive keratectomy. J Korean Ophthalmol Soc. 1998. 40:688–698.
22. Ryou JH, Bae NY, Shyn KH, Kim JC. The change of interleukin-1β and nitric oxide in tear after excimer laser photorefractive keratectomy. J Korean Ophthalmol Soc. 1998. 39:25–36.
23. Jäätela M, Wissing D, Bauer PA, Li GC. Major heat shock protein HSP 70 protects tumor cells from tumor necrosis factor cytotoxicity. EMBO J. 1992. 11:3507–3512.
24. Singh NP, McCoy MT, Tice RR, Schneider EL. Modification of alkaline microgel electrophoresis for sensitive detection of DNA damage. Int J Radiat Biol. 1994. 66:23–28.
25. Singh PN, Michael TM, Raymond RT, Edward L. A simple technique for quantitation of low levels of DNA damage in individual cells. Exp Cell Res. 1988. 175:184–191.


26. Kitazawa T, Tokoro T, Ishii Y. The efficacy of cooling on eximer laser photorefractive keratectomy in the rabbit eye. Surv Ophthalmol. 1997. 42:S82–S88.