Abstract
Effects of pH on vascular tone and L-type Ca2+ channels were investigated using Mulvany myograph and voltage-clamp technique in rabbit basilar arteries. In rabbitbasilar arteries, high K+ produced tonic contractions by 11±0.6 mN (mean±S.E., n=19). When extracellular pH (pHo) was changed from control 7.4 to 7.9 ([alkalosis]o), K+-induced contraction was increased to 128±2.1% of the control (n=13). However, K+-induced contraction was decreased to 73±1.3% of the control at pHo 6.8 ([acidosis]o, n=4). Histamine (10 µM) also produced tonic contraction by 11±0.6 mN (n=17), which was blocked by post-application of nicardipine (1 µM). [alkalosis]o and [acidosis]o increased or decreased histamine-induced contraction to 134±5.7% and 27±7.6% of the control (n=4, 6). Since high K+- and histamine-induced tonic contractions were affected by nicardipine and pHo, the effect of pHo on voltage-dependent L-type Ca2+ channel (VDCCL) was studied. VDCCL was modulated by pHo: the peak value of Ca2+ channel current (IBa) at a holding of 0 mV decreased in [acidosis]o by 41±8.8%, whereas that increased in [alkalosis]o by 35±2.1% (n=3). These results suggested that the external pH regulates vascular tone partly via the modulation of VDCC in rabbit basilar arteries.
One of the major mechanisms for controlling blood circulation is the regulation of vascular resistance through the change in vascular tone. Vascular tone is affected by the changes in extracellular and intracellular pH (pHo and pHi) and it influences blood flow. It is well known that vascular response during acidosis causes significant alteration in blood circulation. Tian et al. (1) reported that hypercapnic acidosis induced vasodilation. And it has been known about role of pHo in vascular function; extracellular acidosis ([acidosis]o) induces relaxation and extracellular alkalosis ([alkalosis]o) develops vice versa. Since cerebral arterial relaxation by [acidosis]o was maintained when the level of pHi was kept constant (1-3), pHo is also very important for the regulation of vascular function.
pH has been shown to modulate calcium influx and to regulate intracellular calcium concentration (4-6). As known well, the change in the intracellular Ca2+ concentration ([Ca2+]i) is responsible for smooth muscle contraction, and H+ also is an important regulator of contractility. In addition, both ions are connected to and changed by each other. Physiologically the alteration of these ions, due to hypoxia or other stimuli, can also affect the distribution of blood and the maintenance of blood pressure. Therefore, the regulation of [Ca2+]i by pH is very important in vascular smooth muscle. In smooth muscle, changes in [Ca2+]i are critical factors for contraction or relaxation and the initiation of other cellular responses. Changes in [Ca2+]i reflect an influx of Ca2+ via the sarcolemmal channels and/or a release from cytosolic Ca2+ stores (sarcoplasmic reticulum, SR) through the processes of Ca2+-induced Ca2+ release (CICR) or through the inositol 1,4,5-trisphosphate (InsP3)-mediated Ca2+ release (7). Among them, Ca2+ influx pathway through voltage-dependent Ca2+ channel (VDCC) in arterial smooth muscle is important for the contractile response, including the maintenance of the basal tone (8, 9). In basilar arteries of rabbit, two types of Ca2+ channels (T and L-type) were reported (12-16). Among them, L-type Ca2+ channel largely contributes to the Ca2+ influx in vascular smooth muscle. Although we could not find T-type Ca2+ channel or test the effects of pHo on that channel in this study, L-type Ca2+ channel is also known to be more sensitively affected by H+ ions in cerebrovascular artery than T-type Ca2+ channel (16, 17, 23). Conclusively, Ca2+ channel especially L-type Ca2+ channel regulations by pH could clearly account for the changes in [Ca2+]i and also in tone of vascular smooth muscle (10-12). In vascular smooth muscle, the regulation of VDCC by pH was reported in pial and porcine coronary arteries (4, 5), including basilar arteries of guinea pigs (16).
Although the effects of pHo on Ca2+ channel of vascular smooth muscle were reported in several cases, the more study about the regulation of Ca2+ channel and contraction by pHo in rabbits basilar arteries is still needed. In addition, some reports suggested that the regulatory effect of Ca2+ channels by pHo was observed, but there was no significant effect on contractile responses (4, 5, 18). For these reasons, this study was designed to verify the role of pHo in the relation between the contractile response and Ca2+ channel in basilar arteries of rabbits.
Single smooth muscle cells were enzymatically isolated from the rabbit basilar arteries. Rabbits (white rabbits from NewZealand, 1.5-2 kg) were anesthetized with sodium pentobarbital (40 mg/kg, i.v.) and exsanguinated. The brain was rapidly removed and placed in phosphate-buffered cold Tyrode's solution containing (in mM): NaCl 145, KCl 5, MgCl2 2, CaCl2 2, glucose 10, NaH2PO4 0.42, Na2HPO4 1.81, HEPES 10, pH 7.4. Then the segment of basilar artery was gently dissected from the surface of the brain stem and was placed in a Ca2+-free phosphate-buffered Tyrode's solution (CaCl2 was omitted from the above compositions). Tunica adventitia, surrounding connective tissue, and side branches were removed gently under a stereomicroscope. The artery was longitudinally dissected and rinsed to remove the residual blood in vessel. The artery was first moved to a nominally Ca2+-free Tyrode's solution containing (in mM): NaCl 145, KCl 5, MgCl2 2, glucose 10, HEPES 10 (pH 7.4 with Tris). It was then cut into small pieces and the segments were stored at 4℃ for 15 min. Collagenase (1.5-1.8 mg; Wako pure chemicals) and dithioerythreitol (0.5 mg; Sigma Chem. Co., St. Louis, MO, U.S.A.), bovine serum albumin (2 mg; Sigma), and trypsin inhibitor (1 mg; Sigma) were dissolved into 1 mL of Ca2+-free Tyrode's solution. Then the arterial segments were incubated in this enzyme cocktail at 35℃ for 20-25 min. After collagenase treatment, segments were transferred to modified K-B solution (see the composition in solution and drug subsection below) and single cells were dispersed by gentle agitation with glass pipette. Isolated single cells were stored at 4℃ until use.
An aliquot of single basilar arterial smooth muscle cells in suspension was added to the recording chamber (0.1 mL) mounted on an inverted microscope (Olympus, IMT-2, Japan). Solutions were superfused through the chamber by gravity at the rate of 2-3 mL/min. Experiments were performed at room temperature. Single cells were voltage-clamped, and membrane currents were measured using the conventional and perforated whole-cell configurations of patch-clamp technique with a patch-clamp amplifier (Axon Instruments, Axopatch-1D, U.S.A.; 19, 20). Patch pipettes were made from borosilicate glass capllaries (inner diameter: 1.5 mm), pulled on a two-step vertical puller (Narishige, PP-83, Japan), and fire-polished with a microforge (Narishige, MF-83); the pipettes had resistances of 3-5 MΩ. To measure whole-cell currents after gigaseal formation, access to the cell interior was obtained by rupturing the membrane at the tip of the pipette with additional negative pressure. Series resistance was not compensated.
Membrane currents were monitored on oscilloscope (Philips, PM 3350, Japan), and data were digitized on-line with an analog-to-digital interface (Axon Instruments, Labmaster TL-1 DMA interface, U.S.A.). Data that were digitized on-line were stored in an IBM-compatible computer. Whole-cell currents were filtered at 5.0 kHz and digitized. All data were analyzed with pClamp 5.5.1 software (Axon Instruments). Leakage current subtraction was performed on data, if necessary.
For the perforated mode patch clamp, amphotericin was dissolved in dimethyl sulfoxide (DMSO) as a stock solution (0.15 mg/2 mL) and added to the back-filling pipette solution (0.15 mg/mL).
Isolated basilar artery was placed in the HEPES-buffered Tyrode's solution, and the vessel ring was prepared (1.5 mm in width). The endothelium of the artery was not removed. Each ring was mounted vertically in organ bath containing 0.5 mL of Ca2+ physiological salt solution (PSS) containing (in mM): NaCl 135, KCl 5, CaCl2 1.8, MgCl2 1, glucose 10, and HEPES (N-[2-hydroxyethyl] piperazine-N'-[2-ethanesulphonic acid]) 10 which was adjusted to pH 7.4 with NaOH. For the 50 mM of K+ Ca2+ PSS solution, equimolar concentration of NaCl was replaced by KCl. The Ca2+ PSS solution was maintained at 37℃ and was continuously aerated with 100% O2. The isometric tension was measured with a force-transducer (Harvard, U.S.A.). Each ring was stretched passively to a resting tension of 300 mg. After equilibration for more than 90 min, contractile response of the strip to the solution containing 50 mM K+ was repeated two or three times until the responses were reproducible.
For the studies of mechanical contractions under various levels of pH, each solution was titrated at 37℃ to various levels of pHs. Then these solutions were pre-incubated with continuous aeration by 100% O2 in water bath (37℃) until the application to the myograph.
Ca2+ PSS containing (in mM) NaCl 135, KCl 5, CaCl2 1.8, MgCl2 1, glucose 10, and HEPES 10, was adjusted to pH 7.4 with NaOH. Modified KB solution (21) containing (in mM) L-glutamate 50, KCl 50, taurine 20, KH2PO4 20, MgCl2 3, glucose 10, HEPES 10, and ethyleneglycol bis-(β-aminoethyl ether-N,N,N',N'-tetraacetic acid (EGTA) 0.5, was adjusted to pH 7.4 with KOH. For conventional whole-cell recording, the following intracellular solution containing (in mM): CsCl 110, TEA 20, EGTA 10, HEPES 10, Na2ATP 3, and MgCl2 3.5, was adjusted to pH 7.3 with TRIZMA or CsOH. For perforated patch experiments, electrodes were filled with solution containing (in mM): CsCl 110, TEA-Cl 20, EGTA 10 and HEPES 10 was adjusted to pH 7.3 with TRIZMA or CsOH. All drugs used in this study were purchased from Sigma.
To exclude the effects of nitric oxide (NO) released from endothelial cells, nitro-L-arginine (L-NNA), an inhibitor of NO synthase, was used. In all experiments, 100 µM L-NNA was pretreated before the application of high K+ solution or the agonist. The pretreatment with L-NNA increased basal tone slightly by 1.1±0.2 mN (n=6, p>0.05, Fig. 1A). When high K+ solution was applied at the normal pHo, the arterial tone was greatly enhanced by 11.4±0.6 mN (n=19) and maintained at a sustained level (Fig. 1, 2). These tonic contractions was almost completely blocked by nicardipine (1 µM) to 7±3.9% (n=7) of the control (Fig. 5A). To elucidate the effects of pHo on K+-induced contraction, pHo was changed from 7.4 to 7.9 [alkalosis]o or to 6.8 [acidosis]o. When the pH of the bath solution was changed from 7.4 to 7.9, the high K+-induced contraction was enhanced reversibly to 128±2.1% (n=13, p<0.01, Fig. 1A, D). However, K+-induced contraction decreased to 87±1.0% and to 73±1.3% of the control at pHo 7.0 and 6.8 in a reversible manner, respectively (n=4, Fig. 1B-D). The effects of [alkalosis]o or [acidosis]o on the K+-induced contraction were also observed in the same tissue. As shown in Fig. 2A, B, enhancing and suppressing effects of pHo on high K+-induced tonic contractions were observed in the same tissue. The enhancing effects of [alkalosis]o on high K+-induced tonic contraction were also observed under the pre-application of pHo 7.9 (Fig. 2C).
As shown in Fig. 3A, histamine (1-20 µM) produced a contraction in a concentration-dependent manner, and maximal contraction was observed approximately at 10 µM of histamine (22). In a few cases, oscillatory small phasic contractions were observed after the application of histamine (Fig. 3B). Histamine (10 µM) developed a tonic contraction with the amplitude of 11±0.6 mN (n=17, Fig. 4), which was blocked by post-application of nicardipine (1 µM), known as a blocker of Ca2+ channel (L-type) (Fig. 3C). From the 14 tested tissues, histamine-induced sustained contractions were suppressed by nicardipine (1 µM) to 21±7.3% of the control (Fig. 5B). These results suggest that Ca2+ influx through Ca2+ channel (L-type) might be important in the histamine-induced tonic contraction. Histamine-induced contractions were enhanced or suppressed under the condition of [alkalosis]o or [acidosis]o to 134±5.7% and to 27±7.6% of the control in a reversible manner (Fig. 4; n=5, 6, p<0.01), respectively.
Since high K+- and histamine-induced sustained contractions were affected by nicardipine and pH, we also studied about the possibility whether the regulatory effects of pHo on high K+- and histamine-induced contractions are associated with Ca2+ channel regulation (Fig. 5). As shown in Fig. 5A, high K+-induced contraction is absolutely nicardipine-sensitive and it is not significantly reversed by [alkalosis]o (9±5.8% of the control, n=7, p>0.05). And also nicardipine-sensitive portion of histamine-induced contraction was not significantly reversed by [alkalosis]o in Fig. 5B (28±10.2% of the control, n=13, p>0.05). These results might imply apparent involvement of Ca2+ channel regulation by pH in high K+- and histamine-induced sustained contractions. Therefore, we studied Ca2+ channel regulation by pH using basilar arterial single myocytes in next step.
Ca2+ current (ICa) was recorded at the physiological calcium concentration of 2 mM under conventional whole-cell configuration (Fig. 6A, B). From a holding potential of -80 mV, depolarization above -30 mV produced an inward current showing a peak current at 0-10 mV, and a reversal around 50 mV (Fig. 6B, open circle). The effects of 1 µM nicardipine on ICa were shown in Fig. 6A, B. In current/voltage (I/V) relation, the peak current of ICa was markedly suppressed at the whole test potential range after the application of nicardipine (1 µM) (Fig. 6B; 6.9±8.4% of the control at 10 mV, n=4). Since the calcium channel current recorded in replacing 2 mM Ca2+ with 10 mM Ba2+ (IBa) is much larger in amplitude, direct effect of pHo on IBa was studied under perforated-patch clamp configuration. From our unpublished data, currents recorded under perforated mode are not different from those under conventional whole-cell mode. The membrane potential was held at -80 mV, and step 0 mV depolarizing pulse was applied for 500 msec every 15 sec. When the peak amplitude of IBa reached a steady-state level, the effects of [alkalosis]o and [acidosis]o on IBa were observed. As shown in Fig. 6C, D, the peak amplitude of IBa increased or decreased under the condition of [alkalosis]o or [acidosis]o. When [alkalosis]o was developed in bath solution, the peak current increased by 35±2.1% (n=3); however, [acidosis]o decreased the peak current by 41±8.8% at 0 mV (n=3).
The results obtained from this experiment suggested that enhancing or suppressing effects of pHo change on the vascular tone are associated with the modulation of VDCC in rabbit basilar arteries. To elucidate the involvement of Ca2+ channel activity in the regulation of high K+- and histamine-induced tonic contraction by pHo, we studied the effects of [alkalosis]o on those contractions in the presence of nicardipine (1 µM). As shown in Fig. 5A, B, nicardipine-sensitive tonic contractions of high K+ and histamine was not significantly recovered by [alkalosis]o. That strongly means that VDCCL is a major target for the regulation of high K+-induced tonic contraction by [alkalosis]o. This experimental procedure enabled us to find the possible mechanism of Ca2+ channel modulation of those contractions by pH.
As a potent modulator of cerebrovascular tone, histamine causes a cerebral vasoconstriction and dilation (8, 9). To date, histamine in vascular smooth muscles has been known to provoke following representative changes in the cytosolic level. Firstly, it can induce IP3 production in smooth muscle, thereby resulting in IP3-induced mobilization of Ca2+ from SR (8). Secondly, it depolarizes vascular smooth muscle cells through the activation of nonselective cation channels (NSCC), thereby increasing Ca2+ influx through VDCC resulting in tonic contraction (8, 9, 24, 29, 30). Thirdly, it can also directly increase Ca2+ current in some smooth muscles (31, 32). Among them, regulation of Ca2+ current is very important since Ca2+ current is associated with tonic contraction. These tonic contraction is simultaneously linked to depolarization and a primary physiological role of agonist-induced depolarization is an activation of VDCC with subsequent influx of Ca2+ and then stimulation of contraction (24-27). In cerebral artery, it was reported that histamine and high K+ produced a sustained tonic contraction that was blocked by 1 µM of nicardipine. Gokina and Bevan (8, 9) reported that Ca2+ influx through VDCC might be responsible for over the 80% of histamine-induced sustained contraction (26). The same result was also reported in human cerebral arteries (33) and observed in this study (Fig. 3C, 4, 5B). These results suggests that the maintenance of histamine-induced sustained tonic contraction might also be responsible for the activation of Ca2+ channel (L-type) through depolarization. Meanwhile, high K+ is also well known to produce depolarization and associated dihydropyridine-sensitive tonic contractions (Fig. 5A) (8). These observations suggest the possibility that the regulation of those tonic contraction through modulation of Ca2+ channel.
Although we suggested the functional role of Ca2+ channel regulation by pHo, some differences in the extent of the effects of pHo between pHo effect on the actions on the Ca2+ channel (L-type) current and that on the K+-induced contraction were also observed. [acidosis]o decreased the peak current of IBa by 41% of the control but reduced K+-induced contraction by 27% of the control (Fig. 1D, 6D). Meanwhile, [alkalosis]o increased the peak amplitude of IBa by 35% of the control, but enhanced K+-induced contraction to 23% of the control (Fig. 1D, 6C). A greater difference was observed in the effects of [acidosis]o on histamine-induced contraction. When [acidosis]o was applied to the histamine-induced contraction, histamine-induced contraction was reduced to 27% of the control (Fig. 3). Eventually, these differences might be originated from the different extent of the activation of VDCC between the tissue and a single cell. According to the other report, high K+ solution (35-66 mM) produced membrane depolarization around -30~-20 mV (8). Under the 50 mM of high K+ solution for the K+-induced contraction, membrane potential in rabbit basilar arteries is expected to be depolarized between -30~-20 mV. Ca2+ channel (L-type) can be activated at these membrane potentials but very small portion of current (approximately 15% of the peak current at 0 mV) will be activated in I/V relation (Fig. 6B). In addition, there might be another possibility that the changes of pHo can affect different ionic conductances such as K+ channels and then lessens the effects of the pHo on Ca2+ channel (L-type). In 1998, the effects of acidosis on Ca2+-activated K+ channel (KCa channel) and ATP-sensitive K+ channel (KATP channel) in coronary artery were reported (7, 34). Although the data were not shown, we observed the effects of TEA and glibenclamide, which are known to be the blockers of KCa channel and KATP channel. Glibenclamide (10 µM) did not show any significant effects on acidosis-induced relaxation of histamine-induced contractions (n=2). However, TEA partially reversed acidosis-induced relaxation of histamine-induced contraction. Therefore, co-involvement of KCa channel activation is suggested with the modulation of Ca2+ channels by [acidosis]o in histamine-induced contraction. Although above several possible ionic conductances which might be involved in the regulation of histamine-induced contraction by the change in pHo were discussed, direct modulatory effect of pHo on histamine-induced contraction should also be considered. To date, in fact, most regulatory effects of pH in the regulation of vascular tone have been studied to determine the interaction between pH, [Ca2+]i and ionic conductances. From these reasons, the interpretation of the effect of pH on vascular tone should be careful until direct effect of pH is established.
As shown in Fig. 3A, histamine produced tonic contractions in a concentration-dependent manner. When various concentrations (1-20 µM) of histamine were applied to bath solution, significant contractions was recorded from 0.5 µM of histamine (Fig. 3B) and maximal contraction was observed at 10 µM of histamine. Histamine (0.5, 1, 3 µM) produced 3±0.9, 42±6.9, and 64±6.4% of the maximal contraction, respectively (n=3, data not shown). Concentration-response relation of histamine in rabbit basilar artery was already reported and our observation is in good agreement to published data (22). In Fig. 3B, some oscillatory vasomotions induced by histamine were observed in rabbit basilar arteries. To date, the physiological significance of active vasomotion in large arteries is not yet clear. Most spontaneous contraction is observed in veins but is rare in large arteries, and it may be induced by neural transmitters including hormonal vasoactive substances (37). However, oscillatory contractions are associated with oscillatory change in [Ca2+]i in vessels (35, 36). In addition, agonist-induced intracellular Ca2+ oscillations were already reported in vascular smooth muscle (37). Such intracellular Ca2+ oscillations may be responsible for the histamine-induced oscillatory vasomotion. We observed the histamine-induced oscillatory contractions in a few cases, and it is known that arteries often produce oscillatory contraction in pathophysiological conditions. Therefore, further study on the oscillatory vasomotion is needed to verify the underlying mechanism of this phenomenon.
In the present study, we tried to elucidate the involvement of VDCC in the changes in pH-induced contraction of vascular smooth muscle. However, the contribution of vascular endothelium to the pH effects on intact vessels should be taken into consideration (38). Two kinds of endothelium-derived mediators have been proposed to account for endothelium-dependent relaxation; one is nitric oxide, and the other an endothelium-derived hyperpolarizing factor (EDHF) (39-41). For these reasons, L-NNA was used to block possible additional involvement of NO-induced inhibitory influences in pHo effects on high K+- and histamine-induced contractions. However, we did not exclude the possible involvement of EDHF in the pHo regulation of contractions in this study. In 1992, Nagao and Vanhoutte suggested that NO-independent relaxation was abolished by high K+ solutions in porcine coronary artery (42). As shown in Fig. 1, [acidosis]o significantly decreased high K+-induced contractions. This findings suggest that inhibitory effects on contractions by [acidosis]o might be EDHF independent relaxation in rabbit basilar arteries. Further studies about the involvement of EDHF in inhibitory effect of [acidosis]o on histamine-induced contraction are needed. To date, regarding the modulation of pHo, most studies on the effects of pH have been done by changing the concentration of the NaHCO3 or PCO2 levels. However, in this study, HEPES-buffered PSS solution was used for recording contractile responses. Since this experiment was designed to compare the effects of pHo both Ca2+ channel current and contraction, the same HEPES-buffered PSS solution (PSS-buffered solution) was used for the studies on contraction and IBa (18).
From these results, it could be concluded that the regulatory effects of pHo on high K+- and histamine-induced tonic contractions might be partly associated with the modulation of L-type Ca2+ channel current in rabbit basilar arteries.
Figures and Tables
Fig. 1
Effects of the alteration of the extracellular pH (pHo) on high K+-induced contraction in basilar arteries of rabbits. Mulvany myograph was used for recording isometric tension of rabbit basilar artery. All contractile experiments in this study were done in the presence of nitro-L-arginine (L-NNA, 100 µM). (A) Superfusion of 50 mM of high K+ solution to the bath provoked tonic contraction. This contraction was enhanced by pHo 7.9 in a reversible manner. (B, C) Under acidic condition such as pHo 7.0 or 6.8 tonic contraction was suppressed in a reversible manner. (D) Bar graphs show mean relative K+-induced contraction by alteration of pHo. Asterisks indicate the data which were considered to be significantly different from control data (**p<0.01).
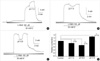
Fig. 2
Effects of the repetitive alteration of pHo on high K+-induced contraction in rabbit basilar artery. Modulatory effects on high K+-induced contraction by the alteration of pHo was monitored by repetitive changes of pHo in rabbit basilar artery. (A) 50 mM of high K+-induced tonic contraction was repeatedly increased by pHo 7.9. (B) Acidotic and alkalotic conditions were induced to 50 mM of high K+-induced tonic contraction. Each condition of pHo 6.8 and 7.9 in a same tissue decreased and increased tonic contraction, respectively, in a reversible manner. (C) In the presence of L-NNA, enhancing effects of alkalotic condition on high K+-induced tonic contraction was studied by pre-application of pHo 7.9. Tonic contraction induced by application of 50 mM of high K+ solution (pHo 7.9) was decreased by post application of normal pH (pH 7.4).
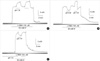
Fig. 3
Histamine-induced contraction in rabbit basilar artery. (A) Histamine (1-20 µM) produced contractions in a concentration-dependent manner. Approximately, 10 µM of histamine produced maximal contraction. (B) In some cases, application of histamine evoked regular small oscillatory phasic contractions. (C) Histamine-induced tonic contraction was suppressed by post-application of nicardipine (1 µM).
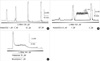
Fig. 4
Effects of alteration of pHo on the histamine-induced contraction in basilar arteries of rabbits. 10 µM of histamine produced tonic contractions in rabbit basilar artery. (A, B) Histamine-induced contraction was decreased or increased by pHo 6.8 or pHo 7.9 in a reversible manner. (C) Bar graphs show mean relative histamine-induced contraction by alteration of pHo. Asterisks indicate the data which were considered to be significantly different from control data (**p<0.01).
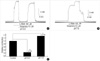
Fig. 5
Effects of pHo in the presence of nicardipine on High K+- and histamine-induced Contraction in Rabbit Basilar Arteries. In A, B, effects of nicardipine and [alkalosis]o in the presence of nicardipine on high K+- and histamine-induced contractions were summarized. Pannel A shows nicardipine completely suppressed high K+-induced sustained contraction and these effect was not reversed by [alkalosis]o significantly. In pannel B, nicardipine suppressed histamine-induced sustained contraction and it was not reversed by [alkalosis]o significantly. Asterisks indicate the data which were considered to be significantly different from control data (**p<0.01).
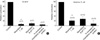
Fig. 6
Effects of alteration of pHo on Ca2+ channel current (IBa) in rabbit basilar artery. The membrane potential was held at -80 mV and 2 mM Ca2+ was used as a charge carrier. Step 10 mV depolarizing pulses from -50 to +60 mV were applied for 500 msec. (A) Depolarizing pulses above -30 mV elicited inward currents (ICa) and nicardipine (1 µM) almost completely blocked ICa. (B) Current/voltage (I/V) relationships of ICa were obtained in the absence and presence of nicardipine (1 µM). In I/V relationships, ICa was significantly suppressed by nicardipine throughout the whole test potential range. 10 mM Ba2+ was used as a charge carrier for the study of regulation of Ca2+ channel current (IBa) by pHo. In C, D effects of alteration of pHo on IBa were studied. Step depolarizing pulse from -80 to 0 mV were applied for 500 msec every 15 sec. When normal external solution (pHo 7.4) was changed to pHo 7.9, IBa was increased in a reversible manner (raw traces of IBa, see insets). However, pHo 6.8 decreased IBa in a reversible manner (raw traces of IBa, insets).
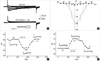
References
1. Tian R, Vogel P, Lassen NA, Mulvany MJ, Andreasen F, Aalkjaer C. Role of extracellular and intracellular acidosis for hypercapnia-induced inhibition of tension of isolated rat cerebral arteries. Circ Res. 1995. 76:269–275.


3. Toda N, Hatano Y, Mori K. Mechanisms underlying response to hypercapnia and bicarbonate of isolated dog cerebral arteries. Am J Physiol. 1989. 257:H141–H146.


4. Klöckner U, Isenberg G. Intracellular pH modulates the avalability of vascular L-type Ca2+ channels. J Gen Physiol. 1994. 103:647–663.
5. Klöckner U, Isenberg G. Calcium channel current of vascular smooth muscle cells: extracellular protons modulate gating and single channel conductance. J Gen Physiol. 1994. 103:665–678.


6. Smith JB, Dwyer SD, Smith L. Lowering extracellular pH evokes inositol polyphosphate formation and calcium mobilization. J Biol Chem. 1989. 264:8723–8728.


7. Ishizaka H, Kuo L. Acidosis-induced coronary arteriolar dilation is mediated by ATP-sensitive potassium channels in vascular smooth muscle. Circ Res. 1996. 78:50–57.


8. Gokina NI, Bevan JA. Histamine-induced depolarization: ionic mechanisms and role in sustained contraction of rabbit cerebral arteries. Am J Physiol Heart Circ Physiol. 2000. 278:H2094–H2104.
9. Gokina NI, Bevan JA. Role of intracellular Ca2+ release in histamine-induced depolarization in rabbit middle cerebral artery. Am J Physiol Heart Circ Physiol. 2000. 278:H2105–H2114.
10. Austin C, Wray S. The effects of extracellular pH and calcium change on force and intracellular calcium in rat vascular smooth muscle. J Physiol. 1995. 488:281–291.


11. Rinaldi CJ, Amando Cattaneo E, Cigolani HE. Interaction between calcium and hydrogen ions in canine coronary arteries. J Mol Cell Cardiol. 1987. 19:773–784.
12. Peng H-L, Jensen PE, Nilsson H, Aalkjaer C. Effect of acidosis on tension and [Ca2+]i in rat cerebral arteries: is there a role for membrane potential? Am J Physiol. 1998. 274:H655–H662.
13. Oike M, Inoue Y, Kitamura K, Kuriyama H. Dual action of FRC8653, a novel dihydropyridine derivative, on the Ba2+ current recorded from the rabbit basilar artery. Circ Res. 1990. 67:993–1006.
14. Tsien RW, Ellinor PT, Horne WA. Molecular diversity of voltage-dependent Ca2+ channels. Trends Pharmacol Sci. 1991. 12:349–354.
15. Worley JF, Quayle JM, Standen NB, Nelson MT. Regulation of single calcium channels in cerebral arteries by voltage, serotonin, and dihydropyridines. Am J Physiol. 1991. 261:H1951–H1960.


16. West GA, Leppla DC, Simard JM. Effects of external pH on ionic currents in smooth muscle cells from the basilar artery of the guinea pig. Circ Res. 1992. 71:201–209.


17. Horie S, Yano S, Watanabe K. Intracellular alkalinization by NH4Cl increases cytosolic Ca2+ level and tension in the rat aortic smooth muscle. Life Sci. 1995. 56:1835–1843.
18. Aoyama Y, Ueda K, Setogawa A, Kawai Y. Effects of pH on contraction and Ca2+ mobilization in vascular smooth muscles of the rabbit basilar artery. Jpn J Physiol. 1999. 49:55–62.
19. Hamil OP, Marty A, Neher E, Sakmann B, Sigworth FJ. Improved patch-clamp techniques for high-resolution current recording from cells and cell-free membrane patches. Pflugers Arch. 1981. 391:85–100.
20. Horn R, Marty A. Muscarinic activation of ionic currents measured by a new whole-cell recording method. J Gen Physiol. 1988. 92:145–159.


21. Isenberg G, Klöckner U. Calcium tolerant ventricular myocytes delivered by pre-incubation in a "KB-medium". Pflugers Arch. 1982. 395:6–18.
22. Suh SH, Han JJ, Park SJ, Choi JY, Sim JH, Kim YC, Kim KW. Differentmechanisms for K+-induced relaxation in various artery. Korean J Physiol Pharmacol. 1998. 3:415–425.
23. So I, Kang TM, Kim KW. Characteristics of Ca currents in rabbit basilar arterial smooth muscle cells. Seoul J Med. 1994. 35:169–182.
24. Bolton TB. Mechanisms of action of transmitters and other substances on smooth muscle. Physiol Rev. 1979. 59:606–719.


25. Carl A, Lee HK, Sanders KM. Regulation of ion channels in smooth muscles by calcium. Am J Physiol. 1996. 271:C9–C34.


26. Kuriyama H, Kitamura K, Nabata H. Pharmacological and physiological significance of ion channels and factors that modulate them in vascular tissues. Pharmacol Rev. 1995. 47:387–573.
27. Nelson MT, Patlak JB, Worley JF, Standen NB. Calcium channels, potassium channels, and voltage dependence of arterial smooth muscle tone. Am J Physiol. 1990. 259:C3–C18.


28. Garland CJ. The role of membrane depolarization in the contractile response of rabbit basilar arery to 5-hydroxytryptamine. J Physiol (Lond). 1987. 392:333–348.
29. Karashima T, Kuriyama H. Electrical properties of smooth muscle cell membrane and neuromuscular transmission in the guinea-pig basilar artery. Br J Pharmacol. 1991. 74:495–504.


30. Sürprenant A, Neild TO, Holman ME. Membrane properties of rabbit basilar arteries and their responses to transmural stimulation. Pflugers Arch. 1987. 410:92–101.
31. Ishikawa T, Hume JR, Keef KD. Modulation of K and Ca2+ channels by histamine H1-receptor stimulation in rabbit coronary artery cells. J Physiol. 1993. 468:379–400.
32. Oike M, Kitamura K, Kuriyama H. Histamine H3-receptor activation augments voltage-dependent Ca2+ current via GTP hydrolysis in rabbitsaphenous artery. J Physiol. 1992. 448:133–152.
33. Takagi T, Tan EC, Shibata S. Characteristics of histamine receptors in human cerebral arteries. Neurol Med Chir (Tokyo). 1993. 33:675–681.


34. Hayabuchi Y, Nakaya Y, Matsuoka S, Kuroda Y. Effect of acidosis on Ca2+-activated K+ channels in cultured porcine coronary artery smooth muscle cells. Pflugers Arch. 1998. 436:509–514.
35. Shimamura K, Sekiguchi F, Sunano S. Tension oscillation in arteries and its abnormality in hypertensive animals. Clin Exp Pharmacol Physiol. 1999. 26:275–284.


36. Tostes RC, Storm DS, Chi DH, Webb RC. Intracellular calcium stores and oscillatory contractions in arteries from genetically hypertensive rats. Hypertens Res. 1996. 19:103–111.


37. Kang TM, So I, Kim KW. Caffeine- and histamine-induced oscillatons of K (Ca) current in single smooth muscle cells of rabbit cerebral artery. Pflugers Arch. 1995. 431:91–100.
38. Gurevicius J, Salem MR, Metwally AA, Silver JM, Crystal GJ. Contribution of nitric oxide to coronary vasodilation during hypercapnic acidosis. Am J Physiol. 1995. 268(1 Pt 2):H39–H47.


39. Cowan CL, Cohen RA. Two mechanisms mediate relaxation by bradykinin of pig coronary artery: NO-dependent and independent responses. Am J Physiol. 1991. 261:H830–H835.


41. Vanhoutte PM. Other endothelium-derived vasoactive factors. Circulation. 1993. 87:Suppl V. V9–V17.