Abstract
Intimal hyperplasia is defined as the abnormal migration and proliferation of vascular smooth muscle cells (VSMCs) with deposition of extracellular matrix. However, the cell cycle regulatory mechanisms of injury-induced VSMC proliferation are largely unknown. To examine the expression kinetics of cell cycle regulatory factors which is known to be worked positively or negatively, we used rat balloon injury model. Marked induction of proliferating cell nuclear antigen (PCNA), G1/S cyclin-dependent kinase (cdk2), and its regulatory subunit (cyclin E) occurred between 1 and 3 days after balloon arterial injury, and this was sustained for up to 7 days and then declined. However, the induction of the negative regulators, p21 and p27, occurred between 3 and 5 days of injury, peaked after 7 and 14 days and was then sustained. VSMC proliferation after balloon catheter injury of the rat iliac artery is associated with coordinated expression of positive (cdk2, cyclin E and PCNA) and negative (p21, p27) regulators. Cell cycle regulators such as cdk2, cyclin E, p21, p27 may be suitable targets for the control of intimal hyperplasia.
Vascular proliferative disease such as primary atherosclerosis, postangioplasty restenosis, and neointimal formation after vein graft have eluded successful pharmacotherapy (1). The activation of vascular smooth muscle cells (VSMCs) is associated not only with entry into the cell cycle, but also with enhanced cell migration, the expression of chemoattractants and adhesion molecules, and extracellular matrix modulation. Platelets, inflammatory cells, damaged vascular endothelial cells (2) and activated VSMCs all secrete growth factors and cytokines that trigger signaling pathways (3-6). Redundant signal transduction processes are likely to prevent the successful targeting of one or two factors. At homeostasis, VSMCs are postmitotic and express markers of the differentiated phenotype. However, mature VSMCs can be undergone phenotypic modulation in response to several environmental stimuli. Pathological VSMC proliferation is thought to play a central role during atherosclerosis and restenosis (7-9). Therefore understanding the fundamental basis of VSMC proliferation is obviously of interest. Previous studies which used several models of angioplasty have demonstrated a rapid VSMC proliferative response of in the media, followed by a second peak of proliferation in the neointima, which then declined to basal levels 2-4 weeks after angioplasty (10, 11). Although the contribution of several cell cycle control genes and growth stimulatory genes to VSMC growth has been well established (12-14), the kinetics of expression of endogenous cell cycle regulatory factors in response to vascular injury remains largely unknown.
Cellular proliferation is controlled by multiple holoenzymes, which include a catalytic cyclin-dependent protein kinase (cdk) and a cyclin regulatory subunit (15-17). Functional cdk/cyclin holenzymes phosphorylate target protein substrates that facilitate progression through the cell cycle. In addition, cdk activity is negatively regulated by cdk interaction with specific cdk inhibitory proteins that cause cell cycle arrest in the G1 phase when overexpressed in transformed and nontransformed cell lines (18, 19). In the early G1 phase, early D-type cyclins and early cdk's accumulate, and p27 binds to and inhibits G1-phase cyclin/cdk complexes. In addition to p27, the early phases of the cell cycles are also regulated by p21, another cell cycle inhibitor. Cyclin E might also be essential for G1-S transition in mammalian cells, in contrast with the three D cyclins, whose lineage specific expression suggest their dispensability in various cell types. The functional inhibition cdk2 also prevents mammalian cells from entering the S phase (20, 21). In the present study, we examined the regulation of cell cycle control proteins after vascular injury. Our findings demonstrate the temporarily and spatially coordinated induction of cdk2, cyclin E, PCNA, p21, and p27 in balloon-injured rat iliac arteries.
The following antibodies were used in this study: (1) rabbit polyclonal antibodies sc-481 (anti-cyclin E), sc-163 (anti-cdk 2), and sc-56 (PCNA), and (2) goat polyclonal antibodies sc-528-G (anti-p27), and sc-397-G (anti-p21), (Santa Crutz Biotechnology, Santa Crutz, CA, U.S.A.)
Acute endothelial injury was performed by denuding the left common iliac artery. Male Sprague-Dawley rats (Korea Experimental Animal Center, Cheongwon) weighing 500 g were anesthetized with sodium pentobarbital (intraperitoneal injection, 45 mg/kg of body weight). The bifurcation of the left common iliac artery was exposed through a midline incision and the distal abdominal aorta, and the right common iliac artery was temporarily occluded. A 2F embolectomy catheter (Baxter Edwards Health Corp., Korea) was introduced into the aorta and advanced to the left iliac artery. The balloon was inflated with saline and drawn toward the arteriotomy site three times to produce a de-endothelializing injury. After the catheter was withdrawn, the arteriotomy was repaired with 8-0 Prolene and blood flow was restored to the iliac artery. The right uninjured iliac artery was used as a tissue control. At the indicated times (6 hr, 1, 3, 5, 7, 14, and 28 day; n=7) after injury, rats were euthanized with sodium pentobarbital, and both injured and uninjured common iliac arteries were perfused with saline and dissected free of the surrounding tissues. For immunohistochemistry, tissue specimens were fixed by immersion in 100% methanol overnight and then embedded in paraffin. Arteries to be used for the preparation of cell extracts were quickly frozen in liquid nitrogen and stored at -80℃ until required.
Rat iliac arteries were fixed by immersion in 100% methanol overnight, embedded in paraffin, and cut into 5 µm sections for immunohistochemistry which was performed using a streptavidin-peroxidase detection system according to the manufacturer's recommendation (Signet Laboratories Inc. Dedham, MA, U.S.A.). Specimens were incubated overnight at 4℃ with anti-cyclin E (1:100 dilution), anti-cdk2 (1:250), anti-p27 (1:250), anti-p21 (1:250), and PCNA (1:50). The biotinylated secondary antibodies were added for 30 min and this was followed by addition of the streptavidin-biotin peroxidase reagent (DAKO) for an additional 30 min. After washing with phosphate buffered saline, antibodies were detected using the ABC method and developed with 3,3'-diaminobenzidine (DAKO). The slides were counter-stained with hematoxylin and examined under a microscope.
Whole cell extracts from rat iliac arteries and cultured cells were prepared in ice-cold lysis buffer, and arterial extracts were prepared using a homogenizer (Tissumizer Mark, OH, U.S.A.). Lysates were centrifuged at 4℃ for 10 min at maximum speed, and the supernatant was stored at -80℃ in small aliquots. The following dilutions of primary antibodies were used for Western blot analysis: anti-cyclin E (1:250), anti cdk2 (1:500), anti-p21 (1:500), anti-p27 (1:500), and PCNA (1:100). Proteins were fractionated on 12% SDS-polyacrylamide gels, and then transferred onto nitrocellulose membrane for and electrophoresed for 2 hr at 60 V. The membranes were preincubated in Tris buffered saline (TBS) including 10% defatted milk powder for 1 hr at room temperature, adequately diluted antibodies were then added, and the membranes were incubated for 1 hr. After washing with TBS-0.1% Tween 20, membranes were further incubated with alkaline-phosphatase-conjugated secondary antibodies for 30 min washed with TBS-0.1% Tween 20 and TBSS, and the reaction was visualized with nitroblue tetrazolium/S-bromo-4-chloro-3-indoyl-1-phosphate.
Three days after intimal injury, microscopic examination showed medial thickening versus the control (Fig. 1). One week after endothelial injury, mild intimal hyperplasia resulting from the fresh accumulation of proliferating smooth muscle cells and connective tissue was observed. Two weeks after injury, marked intimal hyperplasia was observed, and persisted for four weeks.
Positive regulators of cellular proliferation induced after angioplasty included cdk2 and its cognate, cyclin E (Fig. 2). After cyclin E accumulation within 1 day of injury, cdk2 was upregulated at 3 days. Western blot analysis at earlier times revealed the induction of cyclin E within 6 hr, which preceded the upregulation of cdk2. The expressions of these proteins was sustained for 5 days and then declined 28 days after balloon injury. Of note, the temporal expressional pattern of cdk2 and of its cyclin subunits correlated with the expression of PCNA, a marker of cell growth. On the other hand, we tried to show the expression pattern of cell cycle regulatory inhibitors simultaneously. The expression of p21 was minimally observed at baseline and elevated after 3 days, reached a peak at 7 days and then decreased. Expression of p27 was low in early times (6 hr, 1 day, and 3 days) and elevated after 5 days, and reached a maximum at 14 days and then decreased.
To further characterize the kinetics of expression of cell cycle regulatory proteins after balloon injury, we performed immunohistochemical analysis in control and injured rat iliac arteries. Cdk2 expression was found to be upregulated in medial VSMCs 72 hr after injury. After intimal hyperplasia had formed, cdk2 was detected in intimal lesions, but at lower levels in the media. At 2 weeks, cdk2 expression was largely confined to the luminal surface of prominent intimal lesions (Fig. 3). As shown in Fig. 4, cyclin E upregulation was also noted in media 24 hr after balloon injury and increased thereafter. The expression of cyclin E was detected initially in emerging intimal lesions and was then predominantly seen in the luminal surface of the intima at 2 weeks. In p21, p27 as cell cycle regulatory inhibitors, their expressions were detected in medial smooth muscle cells at 5 days and was strongly observed after 1 week and sustained at 4 weeks (Fig. 5, 6). To investigate whether the expressions of cdk2 and cyclin E are spatially correlated with VSMC proliferation following balloon injury, we examined the expression of PCNA by immunohistochemistry. PCNA expression was undetectable in uninjured arteries and its expression was upregulated 72 hr after injury. Later, the expression of PCNA was detected throughout the emerging neointima and subsequently was limited to the luminal surface (Fig. 7). Taken together, these results indicate that VSMC proliferation is correlated with the spatially coordinated expressions of cdk2 and cyclin E after injury of rat iliac artery.
To elucidate the molecular mechanisms underlying the proliferative response of vascular smooth muscle cells (VSMCs) to arterial injury, we analyzed the kinetics of expressions of cell cycle regulatory factors in balloon-injured rat iliac arteries. Intimal hyperplasia in this model of vascular remodeling results primarily from the excessive proliferation of VSMCs, which also undergo dedifferentiation and migration (22, 23). The emergence of VSMCs from quiescence involves a transition from G0 to G1 and the S phases of the cell cycle. These cell cycle phases are coordinated by the expression and activation of regulatory proteins including holoenzymes which are formed from a complex of cyclins and cyclin dependent kinase. Three D type cyclins and cyclin E are key regulators of G1 progression in mammalian cells. Previously high levels of cyclin dependent kinase inhibitors (CKI) declines on induction of cell cycle entry in mitogen deprived (G0) cells (19). In addition to p27, the early phases of the cell cycle are also believed to be regulated by a 21-kDa CKI protein, p21, which is thought to act as a cyclin-cdk assembly and a regulatory factor. Binding of a single p21 molecule may be required for enzymatic cdk activation, whereas complexes bound by multiple p21 subunits are rendered inactive. The level of p21 protein are low in G0-phase cells, are upregulated in the G1 phase, which is believed to provide a counterbalance to the increased accumulation and activity of cyclin/cdk complexes. Importantly, cyclin E might also be essential for the G1-S transition in contrast to D cyclins, whose lineage specific expression connotes the dispensability of at least certain of its members in various cell types (24). Therefore, we focused on the kinetics of cdk2/cyclin E expression in proliferating VSMCs after balloon injury. Western blot analysis revealed a rapid induction and activation of cdk2 cyclin E between 1 and 3 days after injury.
The expressions of these proteins correlated with PCNA expression was sustained up to 10 days after injury and then declined to reach base line levels at 28 days. Immunohistochemical analyses 72 hr after injury revealed cdk2 and cyclin E expressions in medial VSMCs. Subsequently, cdk2 and cyclin E expressions were detected in VSMCs within the emerging intimal lesions and reduced within the media. Later, when a prominent hyperplastic response was present, the expressions of cdk2 and cyclin E were largely confined to the luminal surface of the intima. The expressions of cdk2 and cyclin E were spatially correlated with VSMC proliferation following injury, which was suggested by PCNA immunohistochemistry because it displayed essentially the same expression patterns. These findings are consistent with the results of previous [3H] thymidine and 5-bromodeoxyuridine pulse-labeling experiments in rats (25), which demonstrated peak VSMC proliferation in the media of balloon-injured carotid arteries between 33 and 48 hr that then rapidly declined to baseline levels. Proliferative activity was noted throughout the newly formed intimal lesion, but was later limited to the luminal surface. Protein levels of the CKI's such as p21, and p27 are also variable in vivo. In our studies, p21, and p27 protein were minimally expressed in uninjured arteries and were increased 3 days after injury, peaking at 7, and 14 days respectively. These levels were subsequently found to be mildly decreased at 28 days. Notably, high levels of p27 were correlated with the downregulation of cdk2 at 2 weeks after injury, which was coincident with the termination of VSMC proliferation.
This study demonstrates a striking temporal and spatial correlation between the kinetics of VSMC proliferation and the kinetics of cdk2, cyclin E, p21, and p27 expression. These cell cycle regulators may be suitable targets for the control of intimal hyperplasia after injury, especially p27, because its induction may contribute to the reestablishment of the functional state of VSMCs after injury.
Figures and Tables
Fig. 1
Microscopic findings: photomicrograph of cross section of the injured rat arteries (H&E): Normal iliac artery (control group) with single layer of endothelium in intima and prominent intima was seen from 3 days, marked thickened in 7 days, and sustained thereafter. I, indicate intima and M, media. Black arrows point to the internal elastic lamina. Magnification ×200.

Fig. 2
Sequential change in expression of cell cycle regulators (cdk 2, cyclin E, PCNA, p21, p27) by Western blot analysis after balloon catheter injury of rat iliac artery. Marked induction of cdk2, cyclin E, PCNA occurs between 1 and 3 days after balloon arterial injury, and is peaked in 5 or 7 days and then declined. But induction of negative regulators, p21 and p27 occurs between 3 and 5 days after injury, and is peaked 7 or 14 days and then sustained.

Fig. 3
Immunohistochemical staining of cdk2 after balloon catheter injury of rat iliac artery. Note that cdk2 expression in the medial cells is upregulated within 3 days (C) and then declined thereafter (D, G). At later time points, cdk2 expression is detected throughout emerging intimal lesion (D-E). White arrows point to the internal elastic lamina. Magnification ×200.
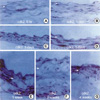
Fig. 4
Immunohistochemical staining of cdk2 after balloon catheter injury of rat iliac artery. Cyclin E expression in the media is noted early at 3 days and 5 days (C, D) and then declined thereafter (E, F). At later time points, cyclin E expression is noticed throughout emerging intimal lesion (D-E). White arrows point to the internal elastic lamina. Magnification ×200.

Fig. 5
Immunohistochemical staining of p21 after balloon catheter injury of rat iliac artery. Note that p21 expression in the medial cells is upregulated at 5 days (D) and then sustained thereafter (E, F). At later time points, cdk2 expression is detected throughout emerging intimal lesion (E-F). White arrows point to the internal elastic lamina. Magnification ×200.

Fig. 6
Immunohistochemical staining of p27 after balloon catheter injury of rat iliac artery. Note that p27 expression in the medial cells is upregulated at 3 days and 5 days (C, D) and then sustained thereafter (E, G). At later time points, p27 expression is detected throughout emerging intimal lesion (D, G). White arrows point to the internal elastic lamina. Magnification ×200.

Fig. 7
Immunohistochemical staining of PCNA after balloon catheter injury of rat iliac artery. PCNA expression is noted in the medial at 3 days (C) and then sustained at 5 and 7 days (D, G) and then declined (F, G). PCNA expression is detected throughout emerging intimal lesion (D-E). White arrows point to the internal elastic lamina. Magnification ×200.
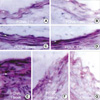
References
1. Herman JP, Herman WR, Vos J, Serruys PW. Pharmacological approaches to the prevention of restenosis following angioplasty: the search for the Holy Grail? Drugs. 1993. 46:18–52.
3. Walker LN, Bowne-Pope DF, Ross R, Reidy MA. Production of platelet derived growth factor-like molecules by cultured arterial smooth muscle cells accompanies proliferating after arterial injury. Proc Natl Acad Sci USA. 1986. 83:7311–7315.
4. Warner SJ, Libby P. Human vascular smooth muscle cells: target for and source of tumor necrosis factor. J Immunol. 1989. 142:100–109.
5. Gibbons GH, Pratt RE, Dzau VJ. Vascular smooth muscle cell hypertrophy vs. hyperplasia: autocrine transforming growth factor-beta 1 expression determines growth response to angiotensin II. J Clin Invest. 1992. 90:456–461.


6. Gospodarowicz D, Ferrara N, Haaparanta T, Neufeld G. Basic fibroblast growth factor: expression in cultured bovine vascular smooth muscle cells. Eur J Cell Biol. 1988. 46:144–151.
7. Owens GK. Regualtion of differentiation of vascular smooth muscle cells. Physiol Rev. 1995. 75:487–517.
8. Fuster V, Badimon L, Badimon JJ, Chesebro JH. The pathogenesis of coronary artery disease and the acute coronary syndromes. N Engl J Med. 1992. 326:242–250.


9. Ross R. The pathogenesis of atherosclerosis: a perspective for the 1990s. Nature. 1993. 362:801–809.


10. Clowes AW, Reidy MA, Clowes MM. Kinetics of cellular proliferation after arterial injury: Smooth muscle growth in the absence of endothelium. Lab Invest. 1983. 49:327–333.
11. Geary RL, Williams JK, Golden D, Brown DG, Benjamin ME, Adams MR. Time course of cellular proliferation, intimal hyperplasia, and remodeling following angioplasty in monkeys with established atherosclerosis. Arterioscler Thromb Vasc Biol. 1996. 16:34–43.


12. Lindner V, Reidy MA. Proliferation of smooth muscle cells after vascular injury is inhibited by an antibody against basic fibroblast growth factor. Proc Natl Acad Sci USA. 1991. 88:3739–3743.


13. Benett MR, Anglin S, McEwan JR, Jagoe R, newby AC, Evan GI. Inhibition of vascular smooth muscle cell proliferation in vitro and in vivo by c-myc antisense oligodeoxynucleotides. J Clin Invest. 1994. 93:820–828.
14. Nabel EG, Yang Z, Liptay S, San H, Gordon D, Haundenschild CC, Nabel GJ. Recombinant platelet-derived growth factor-B gene expression in porcine arteries induce intimal hyperplasia in vivo. J Clin Invest. 1993. 91:1822–1829.
17. Su TT, Stumpff J. Promiscuity rules? The dispensability of cyclin E and Cdk2. Sci STKE. 2004. 2004:11.


19. Sherr CJ, Roberts JM. Inhibitors of mammalian G1 cyclin dependent kinases. Genes Dev. 1995. 9:1149–1163.
20. Pagano M, Pepperkok R, Lukas J, Baldin V, Ansorge W, Bartek J, Draetta G. Regulation of the cell cycle the cdk2 protein kinase in cultured human fibroblasts. J Cell Biol. 1993. 121:101–111.
21. Tsai L, Lees E, Faha B, harlow E, Riabowol K. The cdk2 kinase is required for the G1-to-S transition in mammalian cells. Oncogene. 1993. 8:1593–1602.
22. Wengrovitz M, Selassie LG, Gifford RR, Thiele BL. Cyclosporine inhibits the development of medial thickening after experimental arterial injury. J Vasc Surg. 1990. 12:1–7.


23. Gabeler EE, van Hillegersberg R, Statius van Eps RG, Sluiter W, Mulder P, van Urk H. A comparison of balloon injury models of endovascular lesions in rat arteries. BMC Cardiovasc Disord. 2002. 2:16.


25. Belknap JK, Grieshaber NA, Schwartz PE, Orton EC, Reidy MA, Majack RA. Tropoelastin gene expression in individual vascular smooth muscle cells: relationship to DNA synthesis during vascular development and after arterial injury. Circ Res. 1996. 78:388–394.