Abstract
To examine the impact of lactate dehydrogenase (LDH) as an early marker of ventilator-induced lung injury (VILI) and the effect of prone position during the VILI, we ventilated 28 normal white rabbits (10 supine, 10 prone, 8 controls) for 6 hr or until PaO2/FIO2 ratio was <200 mmHg. We applied an identical injurious ventilatory pattern (peak inspiratory pressure of 35 cmH2O with a PEEP of 3 cmH2O, I:E ratio of 1:2, and FIO2 of 0.40) in the supine and prone group. VILI was assessed by oxygenation, gravimetric analysis and histologic grading. Serum levels of LDH progressively increased significantly during the VILI (supine and prone groups) as compared with controls. There was a significant negative correlation between oxygenation and LDH levels (r=-0.619, p<0.001). Wet weight/dry weight ratios (WW/DW) and histologic scores for dependent regions were significantly higher in the supine than the prone group. There were no differences in WW/DW and histologic scores for nondependent regions between the supine and prone group. These findings suggest that serum LDH levels might be an early marker of severity of lung injury. The prone position resulted in a less severe and more homogenous distribution of VILI.
Mechanical ventilation has been used to support acutely ill patients for several decades. However, the possibility that mechanical ventilation can actually worsen acute lung injury is now widely accepted (1). More recently, what is now known as ventilator-induced lung injury (VILI) has been initially documented experimentally (2) and has received much attention in the clinical fields (3, 4). VILI is mainly viewed as the result of excessive tidal volume, repeated opening and collapse of airways and inflammatory responses that an injurious ventilatory pattern may trigger. Broccard and colleagues (5) demonstrated that animals ventilated with high tidal volume and positive end-expiratory pressure (PEEP) resulted in less extensive histologic change in the prone position than in the supine position. A number of factors could contribute to beneficial effects of prone position to alter dorsal lung transpulmonary pressures, including the compressive effects of consolidated lung, direct transmission of the weight of abdominal content and heart (6).
Lung injury appears to predispose patients to the development of a systemic inflammatory response that culminates in multiple organ dysfunction syndrome and death (7). Several studies have suggested that inflammatory cells and mediators play an important role in the pathogenesis of VILI (8, 9). Supplementation (or blocking) of various inflammatory cytokines has been found to induce (or abrogate) lung injury (9). Behnia et al. (10) demonstrated that serum aspartate aminotransferase and LDH were significantly higher in the positive pressure ventilation with overdistension of the lungs compared with the control group.
The purpose of this study was 1) to examine serum levels of LDH in the supine and prone position during the VILI, 2) to determine whether the locations of VILI are influenced by body position, and 3) to evaluate whether prone position prevents the VILI.
Care of the animals, techniques, and procedures were approved by the Animal Care and Use Committee of Chonnam National University Hospital. New Zealand white rabbits weighing 3.21±0.09 kg were anesthetized with an intramuscular injection of a ketamine hydrochloride (30 mg/kg) and xylazine (0.3 mg/kg). An endotracheal tube (3.5 mm internal diameter) was inserted via a tracheostomy. Mechanical ventilation was initiated in the pressure-controlled mode (IV-110B, Sechrist infant ventilator, Sechrist industries, Anaheim, CA, U.S.A.), with a peak inspiratory pressure of 15 cmH2O, a PEEP of 3 cmH2O, a frequency of 25 breaths/min, an inspiration-to-expiration (I:E) ratio of 1:2, and an inspired oxygen fraction (FIO2) of 0.40. Anesthesia and paralysis were maintained throughout the experiment by continuous infusions of sodium pentobarbital (2-4 mg/kg/hr) and pancuronium bromide (0.03-0.07 mg/kg/hr).
The internal carotid artery was cannulated with a 20-gauge catheter (Custom Product, Abbott Ireland, Sligo, Republic of Ireland) to monitor systemic arterial pressure and heart rate. Arterial blood gas samples were analyzed at 37℃ (Rapidlab 865, Chiron diagnostics corporation, U.K.) and corrected for body temperature. Rectal temperature was monitored and maintained within the range of 37±1℃ using a radiant heating lamp.
After recording baseline hemodynamic and gas exchange, 28 rabbits were randomly assigned to one of three groups (10 supine, 10 prone, 8 control groups). We applied an identical injurious ventilatory pattern (peak inspiratory pressure of 35 cmH2O with a PEEP of 3 cmH2O, I:E ratio of 1:2, and FIO2 of 0.40) for 6 hr or until PaO2/FIO2 ratio was <200 mm Hg to compare the extent of VILI occurring in the prone and supine position. Control groups were ventilated with same baseline settings (peak inspiratory pressure of 15 cmH2O, PEEP of 3 cmH2O, I:E ratio of 1:2, and FIO2 of 0.40). Carbon dioxide was introduced into the inspiratory limb of the ventilator circuit, as necessary to maintain normocapnia (PaCO2, 35 to 45 mmHg) during the induction period. Arterial blood gases were measured at every 1 hr during the experiment. Blood samples for LDH were obtained every 2 hr and measured by enzyme-linked immunosorbent assay (Olympus, AU 5400, Japan). After completion of the protocol, the heart-lung block was then removed after exsanguinations of the heparinized animals.
Heparinization and exsanguinations were used to minimize the effect of residual blood on gravimetric indices. Left lung was physically sectioned into dorsal and ventral regions that were weighed separately to obtain wet weights (WW) and then desiccated in a vacuum oven at 60℃ to dryness for 4-5 days until a constant dry weight (DW).
The resected lungs were infused with 4% paraformaldehyde solution from a tracheostomy tube. Subsequently, the trachea was clamped and the lungs were placed in 4% buffered paraformaldehyde solution. The lungs were divided into dorsal and ventral regions along a coronal plane connecting the mid-height caudal and cranial portions of the lungs. Subdivision into caudal and cranial sectors was accomplished by sectioning the lungs (in the transverse plane) along upper and lower lobe fissures. To avoid sampling bias, tissue samples of 3 µL were excised (regardless of macroscopic appearance) from the mid-portion of each sector. The samples were sectioned and stained (hematoxylin and eosin). The sections were later characterized using a semiquantitative grading system by a pathologist who was blinded as to the experimental protocol and region of sampling. After viewing approximately ten fields per sector under low and high power, each section was assigned a numerical histologic score ranging from 1 to 4, based on the degree of neutrophilic infiltration, hemorrhage, and edema in the interstitial and alveolar spaces as follows (Fig. 1). 1 (normal), normal appearing lung; 2 (mild), mild congestion, interstitial edema, and interstitial neutrophilic infiltrate with occasional red blood cells and neutrophils in the alveolar spaces; 3 (moderate), moderate congestion and interstitial edema with neutrophils partially filling the alveolar spaces but without consolidation; 4 (severe), marked congestion and interstitial edema, with neutrophilic infiltrate nearly filling the alveolar spaces, or with frank lung consolidation. Atelectasis per se was disregarded and not scored as abnormality.
All values are reported as mean±SD, unless specified otherwise. Separate one-way analysis of variance (ANOVA) procedures were used to test for differences in oxygenation and serum levels of LDH. In each group, changes in measured variables over time were analyzed using a repeated-measures analysis of variance. A statistical package, SPSS 11.0 for Windows (SPSS Inc., Chicago, IL, U.S.A.), was used to analyze the data. A value of p<0.05 was considered significant.
There were no significant differences in oxygenation and systemic arterial pressure among the groups under baseline conditions. Systemic arterial pressure trended downward during the VILI in the prone as compared with supine group. However, there were no significant differences in systemic arterial pressure between supine and prone group. In the supine group, PaO2 decreased significantly after 2 hr as compared with baseline values (181.1±26.0 vs. 141.9±46.3 mm Hg; p<0.05). PaO2 decreased significantly after 3 hr as compared with baseline values in the prone group (182.0±19.2 vs. 137.1±47.2 mm Hg; p<0.05). Although PaO2 tended to be lower in the supine than prone group during the VILI, this change did not reach significance between two groups at end of experiment. PaO2 remained stable for 6 hr in the control group (Fig. 2).
Serum levels of LDH elevated progressively during the experiment in injurious ventilatory pattern (supine and prone position) when compared with the control groups (Fig. 3). There were no significant differences of serum levels of LDH between supine and prone position at the end experiment (supine: 679±423 U/L; prone: 435±174 U/L; p=0.51). In the control group, the trend toward elevated levels of LDH with time was not statistically significant when compared with baseline values. There was a significant negative correlation between oxygenation and LDH levels (r=-0.619, p<0.001) (Fig. 4).
The ratio of wet weight to dry weight (WW/DW) for dependent and nondependent regions was significantly greater in injurious ventilatory pattern (supine and prone) than the control group. The WW/DW for dependent regions was significantly higher in the supine than prone group. There were no differences in WW/DW for nondependent regions between supine and prone group. When dependent and nondependent regions were compared in the same animal, regional WW/DW was significantly greater in dependent in the supine group (dependent: 6.36±0.72; nondependent: 4.78±0.37; p<0.01). In the prone group, however, no significant differences was found in WW/DW between dependent and nonde pendent regions (dependent: 4.34±1.20; nondependent: 4.32±1.49; p=0.56) (Fig. 5).
Gross postmortem inspection of the lungs in the supine group revealed edema and hemorrhage that were most prominent in the dependent regions. These changes appeared much less intense in the prone position. Histologic scores for dependent and nondependent were significantly higher in injurious ventilatory pattern (supine and prone) as compared with the control group (Fig. 6). In the dependent regions, mean histologic scores were significantly higher in the supine than the prone group (supine: 3.06±0.36; prone: 2.20±0.27; p=0.04). In the nondependent regions, however, mean histologic scores did not differ in both groups (supine: 2.31±0.12; prone: 2.01±0.46; p=0.52). Histologic scores were greater in dependent than in nondependent regions for animals ventilated in the supine group (p=0.02). In the prone group, however, no significant differences was found in histologic scores between dependent and nondependent regions (p=0.44).
The main findings of this study were that in the VILI models 1) PaO2 tended to be lower in the supine than prone group during the VILI. However, this change did not reach significance between two groups at end of experiment. PaO2 remained stable for 6 hr in control group; 2) Serum levels of LDH progressive increased during the induction of VILI in the supine and prone position. There was a significant negative correlation between oxygenation and serum levels of LDH; 3) WW/DW and histologic scores for dependent and nondependent regions were significantly greater in the injurious ventilatory pattern (supine and prone group) than in the control group. Dependent regions with tissue damage and edema observed in the supine were attenuated by the prone group.
Despite advance in critical care, the mortality rate in patients with acute respiratory distress syndrome (ARDS) remains high at values exceeding 30-40%. Furthermore, most patients who die do so from multiple system organ failure rather than from hypoxia (7). One hypothesis that has recently been advanced to explain this observation is that mechanical ventilation per se may be not only exacerbation of underlying lung injury but may also lead to the development of a systemic inflammatory response syndrome (2, 8, 9) and multiple system organ failure (11, 12).
Two mechanisms are thought to contribute to the development of VILI: overdistension of the alveoli and shearing forces caused by the stress that occurs where collapsed areas of the lungs are reopened by positive pressure. Exactly how mechanical ventilation induces its deleterious effects is as of yet unclear. Studies in vitro and in vivo have found that both the pattern and the degree of stretch are important in determining cellular response (9, 12). Supplementation (or blocking) of various inflammatory cytokines has been found to induce (or abrogate) lung injury (13). Ranieri et al. (14) demonstrated that the concentrations of proinflammatory cytokines in both bronchoalveolar lavage (BAL) and plasma could indeed be decreased in patients ventilated with a lung-protective strategy. A report showed that serum aspartate aminotransferase and LDH were significantly higher in the positive pressure ventilation with overdistension of the lungs compared with the control group (10). These results suggested that serum LDH might be early markers of ventilator-induced lung injury in the rat model. Our results showed that serum levels of LDH elevated progressively during the experiment in the injurious ventilatory pattern, whereas serum levels of LDH remained stable for 6 hr in the control groups. There was a significant negative correlation between oxygenation and LDH levels.
Patients with ARDS are frequently kept in the supine position for days to weeks. However, many researchers suggest that the primary horizontal posture should be prone rather than the supine (15). The prone position has been proposed as a relatively simple maneuver to improve oxygenation (16), and an increased end-expiratory lung volume was originally suggested as the main mechanism for oxygenation improvement (17). Several studies found that the prone position causes a venous admixture reduction and a more even ventilation distribution, without affecting end-expiratory lung volume (18, 19). More recently, experimental reports demonstrated that the prone position may be reducing the VILI (5, 20). Broccard and colleagues (20) demonstrated that lung injury induced solely by mechanical forces, the prone position resulted in a less severe and more homogeneous distribution of VILI in the dog models. These observations are consistent with our results. In our VILI model, we observed a dependent predominance of tissue edema and histologic change in the supine position. Dependent and nondependent scores were similar in the prone position. However, the exact mechanism for the attenuated lung injury during prone ventilation remains speculative. In the present study we found that PaO2 tended to be lower in the supine than prone group during the VILI. However, this change did not reach significance between two groups at the end of experiment. PaO2 remained stable for 6 hr in the control group. Based on our results, we suggest that reduction in tidal volume is the more important therapeutic intervention for the significant reduction of VILI. Experimentally, use of high levels of PEEP (21), partial liquid ventilation (22), and proinflammatory cytokine neutralization (23) might be reducing VILI, but clinical proofs of efficacy are still lacking.
Despite its interesting results, this study was limited in several ways. In particular, our model did not evaluate long-term effects of prone position. Further investigation is needed to clarify the effects of mechanical ventilation on the lungs during periods of longer duration. Imai et al. (24) demonstrated that mechanical ventilation can lead to epithelial cell apoptosis in the kidney and small intestine, accompanied by biochemical evidence of organ dysfunction. Further experimental and clinical evidence will be required to explain VILI correlated with multiple organ dysfunction syndrome and examine other potential strategies for prevention the VILI.
Figures and Tables
Fig. 1
Representative examples of the histologic scores (HS). (A) HS 1: normal; (B) HS 2: mild; (C) HS 3: moderate; (D) HS 4: severe. Hematoxylin and eosin, magnification (×200).
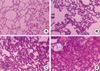
Fig. 2
Comparisons of oxygenation among the groups. PaO2 decreased significantly after 2 and 3 hr in the supine and prone groups as compared with baseline values, respectively. Data represent the mean±SD. *p<0.05 versus baseline values.
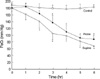
Fig. 3
Comparisons of lactate dehydrogenase among the groups. Serum levels of LDH elevated progressively during the VILI (supine and prone position) when compared with control groups. Data represent the mean±SD. *p<0.05 versus baseline values.
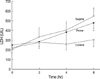
Fig. 4
Correlation between lactate dehydrogenase and oxygenation. There was a significant negative correlation between oxygenation and LDH levels.
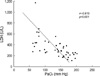
References
1. Parker JC, Hernandez LA, Peevy KJ. Mechanisms of ventilator-induced lung injury. Crit Care Med. 1993. 21:131–143.


2. Dreyfuss D, Saumon G. Ventilator-induced lung injury: lessons from experimental studies. Am J Respir Crit Care Med. 1998. 157:294–323.
3. Amato MB, Barbas CS, Medeiros DM, Magaldi RB, Schettino GP, Lorenzi-Filho G, Kairalla RA, Deheinzelin D, Munoz C, Oliveira R, Takagaki TY, Carvalho CR. Effect of a protective-ventilation strategy on mortality in the acute respiratory distress syndrome. N Engl J Med. 1998. 338:347–354.


4. Brochard L, Roudot-Thoraval F, Roupie E, Delclaux C, Chastre J, Fernandez-Mondejar E, Clementi E, Mancebo J, Factor P, Matamis D, Ranieri M, Blanch L, Rodi G, Mentec H, Dreyfuss D, Ferrer M, Brun-Buisson C, Tobin M, Lemaire F. The Multicenter Trial Group on Tidal Volume reduction in ARDS. Tidal volume reduction for prevention of ventilator-induced lung injury in acute respiratory distress syndrome. Am J Respir Crit Care Med. 1998. 158:1831–1838.
5. Broccard AF, Shapiro RS, Schmitz LL, Ravenscraft SA, Marini JJ. Influence of prone position on the extent and distribution of lung injury in a high tidal volume oleic acid model of acute respiratory distress syndrome. Crit Care Med. 1997. 25:16–27.


6. Pelosi P, D'Andrea L, Vitale G, Pesenti A, Gattinoni L. Vertical gradient of regional lung inflation in adult respiratory distress syndrome. Am J Respir Crit Care Med. 1994. 149:8–13.


7. Montgomery AB, Stager MA, Carrico CJ, Hudson LD. Causes of mortality in patients with the adult respiratory distress syndrome. Am Rev Respir Dis. 1985. 132:485–489.


8. Ghosh A, Greenberg ME. Calcium signaling in neurons: molecular mechanisms and cellular consequences. Science. 1995. 268:239–247.


10. Behnia R, Molteni A, Waters CM, Panos RJ, Ward WF, Schnaper HW, TS'Ao CH. Early markers of ventilator-induced lung injury in rats. Ann Clin Lab Sci. 1996. 26:437–450.
11. Slutsky AS, Tremblay LN. Multiple system organ failure. Is mechanical ventilation a contributing factor? Am J Respir Crit Care Med. 1998. 157:1721–1725.
12. Tremblay LN, Slutsky AS. Ventilator-induced injury: from barotrauma to biotrauma. Proc Assoc Am Physicians. 1998. 110:482–488.
13. Chernoff AE, Granowitz EV, Shapiro L, Vannier E, Lonnemann G, Angel JB, Kennedy JS, Rabson AR, Wolff SM, Dinarello CA. A randomized, controlled trial of IL-10 in humans. Inhibition of inflammatory cytokine production and immune responses. J Immunol. 1995. 154:5492–5499.
14. Ranieri VM, Suter PM, Tortorella C, De Tullio R, Dayer JM, Brienza A, Bruno F, Slutsky AS. Effect of mechanical ventilation on inflammatory mediators in patients with acute respiratory distress syndrome: a randomized controlled trial. JAMA. 1999. 282:54–61.


15. Messerole E, Peine P, Wittkopp S, Marini JJ, Albert RK. The pragmatics of prone positioning. Am J Respir Crit Care Med. 2002. 165:1359–1363.


16. Piehl MA, Brown RS. Use of extreme position changes in acute respiratory failure. Crit Care Med. 1976. 4:13–14.


17. Douglas WW, Rehder K, Beynen FM, Sessler AD, Marsh HM. Improved oxygenation in patients with acute respiratory failure: the prone position. Am Rev Respir Dis. 1977. 115:559–566.
18. Albert RK, Leasa D, Sanderson M, Robertson HT, Hlastala MP. The prone position improves arterial oxygenation and reduces shunt in oleic-acid-induced acute lung injury. Am Rev Respir Dis. 1987. 135:628–633.
19. Lamm WJ, Graham MM, Albert RK. Mechanism by which the prone position improves oxygenation in acute lung injury. Am J Respir Crit Care Med. 1994. 150:184–193.


20. Broccard A, Shapiro RS, Schmitz LL, Adams AB, Nahum A, Marini JJ. Prone positioning attenuates and redistributes ventilator-induced lung injury in dogs. Crit Care Med. 2000. 28:295–303.


21. Dreyfuss D, Soler P, Basset G, Saumon G. High inflation pressure pulmonary edema. Respective effects of high airway pressure, high tidal volume, and positive end-expiratory pressure. Am Rev Respir Dis. 1988. 137:1159–1164.


22. Dreyfuss D, Martin-Lefevre L, Saumon G. Hyperinflation-induced lung injury during alveolar flooding in rats: effect of perfluorocarbon instillation. Am J Respir Crit Care Med. 1999. 159:1752–1757.
23. Bone RC, Fisher CJ Jr, Clemmer TP, Slotman GJ, Metz CA. Early methylprednisolone treatment for septic syndrome and the adult respiratory distress syndrome. Chest. 1987. 92:1032–1036.


24. Imai Y, Parodo J, Kajikawa O, de Perrot M, Fischer S, Edwards V, Cutz E, Liu M, Keshavjee S, Martin TR, Marshall JC, Ranieri VM, Slutsky AS. Injurious mechanical ventilation and end-organ epithelial cell apoptosis and organ dysfunction in an experimental model of acute respiratory distress syndrome. JAMA. 2003. 289:2104–2112.

