Abstract
Background
Left ventricular (LV) torsion plays an important role in both LV systolic and diastolic function. Notwithstanding the fact that speckle tracking imaging echocardiography (STI) is a validated method to measure LV torsion, few data regarding the clinical significance of LV torsional parameters using STI on exercise capacity during exercise echocardiography were reported.
Methods
Fifty four participants completed the supine bicycle cardiopulmonary exercise echocardiography under a symptom-limited protocol. LV torsion was defined as the net difference between LV peak apical rotation, and basal rotation divided by LV diastolic longitudinal length. LV basal, and apical short-axis rotations at each stage were analyzed by STI.
Results
LV torsion measurement was feasible in 43/54 (80%) at peak exercise. The LV torsions were increased during exercise, and even until the recovery. Peak twisting, and untwisting velocities were significantly increased during exercise, but were decreased at recovery. As expected, baseline torsion was positively correlated with LV ejection fraction and baseline apical peak untwisting velocity has correlation with E/E' (r=0.50, p<0.01 and r=0.30, p<0.05, respectively). Interestingly, apical peak twisting velocity at peak exercise was significantly correlated with maximal O2 consumption and VO2 interval change (r=0.50, p<0.01 and r=0.33, p<0.05, respectively).
Conclusion
It was feasible to measure LV torsion by STI at every step during exercise echocardiography, although the feasibility was relatively low at peak exercise. LV torsional parameters during exercise showed significant relations with exercise capacity as well as LV systolic and diastolic functions.
The Left ventricular (LV) torsion is a force generated between a counterclockwise rotation of the ventricular apex, and a clockwise rotation of the base. It plays an important role in both LV systolic and diastolic function, and it is now widely accepted that LV torsion is sensitive to changes in both regional and global LV function.1)2)
Echocardiography offers a new way of assessing LV torsion for its non-invasiveness, and instant availability. Two-dimensional (2D) echocardiography using the papillary muscle as landmarks,1)4) Doppler Tissue Imaging (DTI),5) and Speckle Tracking Imaging (STI)6)7) are being competitively evaluated, to become a promising non-invasive tool for LV torsion assessment. 2D-echocardiography, however, does not meet the original idea of torsion, for it only measures one level, rather than whole LV length. Moreover, DTI shows angle dependency and low spatial resolution. STI, on the other hand, seems to be given the edge in this competition, as the development of 2D-STI has allowed LV rotation and torsion to be evaluated non-invasively.3)6-9)
LV torsion was calculated as a net difference between LV peak rotation angles obtained from basal (clockwise), and apical (counterclockwise) short-axis planes normalized by ventricular diastolic longitudinal length between the LV apex, and mitral plane.10) According to previous studies, the LV torsion estimated by STI was concordant with the data analyzed by tagged MRI, and also showed similar results with those evaluated by DTI.6)
LV systolic and diastolic function can be altered due to exercising activities. The former becomes augmented and the latter becomes attenuated.11)12) Exercise stress echocardiography is an established method for detecting inducible myocardial ischemia. Furthermore, supine bicycle cardiopulmonary exercise echocardiography is also an accepted method for evaluating regional cardiac function as well as measuring maximal exercise capacity.
On this basis above, it was hypothesized that the LV torsion measured by STI in supine bicycle cardiopulmonary exercise echocardiography would be very valuable in evaluating LV function, measuring exercise capacity, and detecting heart diseases as well. Thus, the aims of this study was to assess the feasibility of LV torsion measurement using STI during exercise, and to examine the clinical significance of LV torsional parameters on exercise capacity.
Participants designated for the study visited Gil hospital with various symptoms (chest pain, dyspnea etc.), all of which were proved by clinical examinations to have normally functioning hearts. Exclusion criteria included uncontrolled hypertension, lung disease, ischemic or valvular heart disease, and the use of cardiac medication. In addition, a transthoracic echocardiogram was performed to exclude the presence of LV hypertrophy, valvular heart disease, and global or regional wall-motion abnormalities. A total of 57 participants (27 women and the mean age at 45.0 years) were recruited in the study. The study was approved by the institutional review board of our hospital, and all participants provided an informed consent before the study was conducted.
All 57 participants completed the supine bicycle cardiopulmonary exercise echocardiography. The study was conducted using a symptom-limited protocol with a supine bicycle (Lode, Groningen, Netherland) at an initial workload of 50 W for 2 minutes, and an extra 25 W was loaded every 2 minutes to intensify the exercise until physical exhaustion. A 12-lead electrocardiogram, and blood pressure reading were recorded at baseline every 2 minutes thereafter. Heart rate (HR), and rhythm were monitored into the recovery period. In addition, O2 consumption was measured from resting time to recovery time by gas analyzer (Quark PFT-ergo, Cosmed, Rome, Italia). Standard 2-dimensional echocardiography was performed at baseline, during exercise, and recovery of the supine in the left lateral position of the patient. A commercially available ultrasound transducer and equipment (M3S probe, Vivid 7, GE Medical System, Horten, Norway) was used. The standard echocardiographic examination included measurements of LV systolic, diastolic dimensions, wall thicknesses, LV ejection fraction (LVEF), and pulsed wave Doppler examination of the mitral inflow.
For the assessment of LV twist, two LV short-axis planes were obtained from basal and apical levels at high frame rates (range 55-80 frames/s). In exercise echocardiography, maintaining high frame rates was difficult, since intense translation of heart during exercise limited to restrict a narrowing sector range, which induced high frame rates. Despite the efforts to generate high frame rates, 55-80 frames/s was the maximal frequency reached in this study.
One of the goals of the study was to make sure that the basal short-axis plane contained the mitral valve, and that the apical plane was LV cavity with no visible papillary muscles. In each short axis image, the LV cross section was made as circular as possible. At each plane, three consecutive cardiac cycles were acquired and digitally stored in a hard drive for offline analysis.
After obtaining standard echocardiographic imaging, a commercially available 2D strain software (EchoPac 7.0.0 for PC, GE medical system) was used to quantify basal and apical LV rotations. 'LV rotation' was used as a parameter to represent angle of movements at each level of LV in systole, and diastole, while 'twisting and untwisting velocity' represented the rate of rotation at each level of LV. Measuring LV rotation, LV twisting, and untwisting velocity was accomplished in the following processes.
One cardiac cycle with an appropriate image quality was selected for subsequent analysis. Aortic valve closure was determined by apical 3-chamber view, and the endocardial border of each short axis in the end-systolic frame were manually traced. A speckle tracking region of interest was automatically selected to approximate the entire myocardium, yet it frequently needed to be adjust manually. This was followed by an software algorithm which automatically segmented the LV short axis into 6 equidistant segments, and selected suitable speckles for tracking. Once these series of steps were completed, the software algorithm searched these speckles on a frame-by-frame basis using the sum of absolute difference algorithm. The software estimated LV rotation, LV twisting, and untwisting velocity profiles in each short-axis image, defining the ventricular centroid from the midmyocardial line on each frame. The LV rotation profile was, then, smoothed with cubic spline interpolation, from which LV twisting and untwisting velocity was estimated by differentiation. Averaged LV rotation, LV twisting, and untwisting velocity profile data of tracked mid-myocardium were used for the calculation of LV torsion.6)9)
Counterclockwise rotation, as viewed from the LV apex, was expressed as a positive value, whereas a clockwise rotation was as a negative value (Fig. 1 and 2). Data points of the basal and apical LV rotation, LV twisting and untwisting velocity and time (from the R wave to peak positive velocity and from the aortic valve closure to peak negative velocity) were exported to a spreadsheet program (SPSS 15.0, SPSS Inc, Chicago, Ill) to calculate LV torsion (Fig. 3 and 4). LV torsion was calculated as the net difference between LV peak rotation angles obtained from basal (clockwise) and apical (counterclockwise) short-axis planes divided by ventricular diastolic longitudinal length between the LV apex and the mitral plane.
Data were expressed as mean±SD or percentages. Pearson's correlation analysis was used to reveal relations between two continuous variables. ANOVA analysis and paired-samples T test were used to compare means of groups. All statistical analyses were performed with software (SPSS 15.0, SPSS Inc, Chicago, Ill) and a p value less than .05 was considered statistically significant.
A positive correlations between torsion at baseline and LVEF (r=0.50, p=0.001) was discovered in the study (Fig. 5A). On the other hand, positive correlations between the absolute value of basal rotation at baseline and LVEF (r=0.47, p=0.001) and between basal peak twisting velocity at baseline and LVEF (r=0.46, p=0.001) were also observed.
Apical peak untwisting velocity at baseline showed positive correlation with E/E' (r=0.30, p<0.05), as well (Fig. 5B).
LV rotation, torsion, peak twisting velocity and peak untwisting velocity at basal and apical level were shown in Table 3. The torsions were increased at exercise and even recovery (Fig. 6A). The change of torsion from baseline to peak exercise (Δtor) was 19.0±45.7%, which turned out to be statistically insignificant (p=0.3). However, the change of torsion from baseline to recovery was 27.1±54.6% and statistically significant (p=0.012).
Both peak twisting and untwisting velocity at basal and apical level was significantly increased as the workload of exercise was increased in contrast to the torsion or rotation, which both decreased at recovery (Fig. 6B and C).
The exercise time positively correlate with torsion at peak exercise (r=0.33, p<0.05) and also was correlated with Δtor (r=0.47, p=0.002). Maximal O2 consumption (MVO2, mL/min/kg) correlated with apical peak twisting velocity at peak exercise (r=0.5, p<0.001) (Fig. 7). The maximal O2 consumption, however, had no significant relation to torsion at any stage (all p>.05) while VO2 change from 75 W exercise to peak exercise (ΔVO2) had correlation with torsion at recovery (r=0.29, p<0.05). In addition, ΔVO2 had significant correlation with Δtor (r=0.37, p=0.02), with apical peak twisting velocity at peak exercise (r=0.33, p=0.02), and with apical rotation at peak exercise (r=0.34, p<0.05).
The data of 54 patients were analyzed out of a total of 57 patients, as the data of 3 patients were omitted in the process of data transmission. LV basal rotation measurements by STI were possible in 52/54 (96%) at baseline, 51/54 (94%) at 75 W exercise, 44/54 (81%) at peak exercise and 53/54 (98%) at recovery. LV apical rotations were measured in 53/54 (98%) at baseline, 52/54 (96%) at 75 W exercise, 50/54 (93%) at peak exercise and 53/54 (98%) at recovery. The major cause of exclusion was poor quality of images. So, LV torsion computation were feasible in 51/54 (94%) at baseline, 49/54 (90%) at 75 W exercise, 43/54 (80%) at peak exercise and 52/54 (96%) at recovery (Fig. 8). The relatively low feasibility at peak exercise was a result of the poor quality of images, especially of basal rotation.
Inter-observer variability for LV torsion measurements were obtained by two independent observers, and the intra-class correlation coefficients were 0.91 at baseline, 0.95 at 75 W exercise, 0.71 at peak exercise and 0.95 at recovery. For intra-observer variability of LV torsion, the intra-class correlation coefficients were 0.94 at baseline, 0.79 at 75 W exercise, 0.82 at peak exercise and 0.95 at recovery.
According to many earlier reports, left ventricular torsion originates from rotations of base, and apex located on the opposite of the heart, and is well known for its important role for both LV systolic and diastolic function. Among the methods to measure LV torsion, STI using 2D ultrasound is now widely accepted as reliable tool for its non-invasiveness, and angle independency. In this study, measurements of LV torsion using STI on all stages of exercise echocardiography were performed. In addition, to discover the effect of exercise on LV torsion or function, a supine bicycle cardiopulmonary exercise echocardiography was used.
Measurements of LV torsion were possible in most patients, but the torsion on peak exercise was obtained in relatively low feasibility, inter/intra-observer variability. The causes were thought to be increased heart rate during exercise, which was led to a shorter length of cardiac cycle, and lower frame rate than baseline, or the recovery phase. This led to poor quality of images on echocardiography, which made speckle tracking more difficult. For this reason, the chances of failure to measure basal rotations particularly increased as the extent of exercise was intensified.10) It was also observed that the speckle tracking was not perfect, because of assessing only longitudinal contraction, being affected from cardiac translation, and measuring by insufficient frame rate. To apply this method to the clinical the field, the development of a more sophisticated program with delicate and trained echocardiographic techniques are necessary.
With augmented exercise intensity, the basal rotations irregularly increased, and the apical rotations were continually increased, whereas all of these results showed no significant difference (all p>0.05). The torsion computed at basal and apical rotations showed increasing tendency as the extent of exercise were augmented and this result was consistent with earlier reports.2)4)12) Unexpectedly, however, the rotation, and torsion at recovery were also continually increased. The significance of this discovery is that it has never been reported. This may have resulted from measurements undergone too early before full recovery, although the measurement of echocardiography was attempted, as the heart rate decreased back to the baseline level. In addition, the better quality of images at recovery could make it easy to track apical rotation.
Peak twisting and untwisting velocity at basal and apical level were significantly increased during exercise. However, these velocities were decreased at recovery in contrast to rotation or torsion. The cause of this phenomenon was mainly due to relatively increased length of cardiac cycle at recovery phase. This made peak twisting and untwisting velocity decreased despite larger torsion at recovery than even that at peak exercise.
As it has been reported in most previous studies, LV ejection fraction showed a positive correlation between torsion at baseline, and apical rotation at baseline, while it showed a negative correlation with basal rotation at baseline and with basal peak twisting velocity at baseline and exercise. These results indicate that apical rotation plays a central role in determining LV systolic function.5)10)
Besides, there was a correlation between E/E', and apical peak untwisting velocity at baseline. With this notion in mind, it may be suggested that the untwisting velocity may be a promising parameter of diastolic function.13-16)
Exercise time is an indication of exercise capacity, as it is, by definition, the duration of peak exercise until exhaustion. Exercise time showed correlation with torsion at peak exercise and Δtor. Both MVO2 and ΔVO2 had positive correlation with apical peak twisting velocity at peak exercise. Especially, ΔVO2 showed positive correlation with apical rotation at peak exercise and Δtor. These results suggest that torsion and twisting velocity can represent exercise capacity.
Study Limitations. The measurements of LV torsion using STI has been recently validated by previous studies, although it has as yet a few limitations. First, the tracking is affected by frame rate and quality of images. In this study, it was very hard to gather a suitable quality of echocardiographic images with high frame rate during exercise. Due to this reason, clinical feasibility of LV torsion at peak exercise, inter- and intra-observer variability was relatively decreased, and this may suggest that STI would be recommended at suboptimal phase of exercise, for example at 75 W. However, it is acceptable that feasibility of LV torsion at peak exercise was about 80% and inter- and intra-observer variability were over 0.7. Second, STI using 2D echocardiography does not reflect the longitudinal contraction, and this problem indicates that the tracking is not able to be performed at the same level of LV during a cardiac cycle: it cannot present the real three dimensional cardiac movements. Finally, it was impossible to compare parameters at baseline with that after exercise because the LV functional parameters during exercise were not recorded.
In this study, LV torsion, twisting and untwisting velocitities were measured for the first time by STI through stepwise analysis. The measurement of LV torsion during exercise was feasible, although the feasibility at peak exercise was relatively low. As expected, baseline LV torsional parameters showed positive relations to LV systolic, and diastolic function. Apical peak twist ing velocity, in particular, showed a strong relevance to maximal O2 consumption at peak exercise.
Figures and Tables
Fig. 1
Basal rotation during exercise and recovery phase. The white arrowheads represent each peak values. At baseline (A), 75 W exercise (B), peak exercise (C) and recovery (D), each basal rotation represents negative value (clockwise rotation) and increases during exercise and even till the recovery phase.
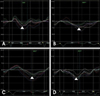
Fig. 2
Apical rotation during exercise and recovery phase. The white arrowheads represent each peak values. At baseline (A), 75 W exercise (B), peak exercise (C) and recovery (D), each apical rotation represents positive value (counterclockwise rotation) and increases during exercise and even till the recovery phase.
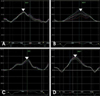
Fig. 3
Basal peak twisting and untwisting velocities during exercise and recovery phase. The white arrowheads represent each peak twisting velocities and yellow arrowheads represent each peak untwisting velocities. At baseline (A), 75 W exercise (B), peak exercise (C) and recovery (D), both twisting and untwisting velocity were increased during exercise and decreased at recovery phase.
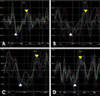
Fig. 4
Apical peak twisting and untwisting velocity during exercise and recovery phase. The white arrowheads represent each peak twisting velocities and yellow arrowheads represent each peak untwisting velocities. At baseline (A), 75 W exercise (B), peak exercise (C) and recovery (D), both twisting and untwisting velocity were increased during exercise and decreased at recovery phase.
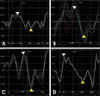
Fig. 5
The correlation between torsional parameters at baseline and left ventricular functional parameters. There were positive correlations between torsion at baseline and left ventricular ejection fraction (r=0.50, p=0.001) (A). Apical peak untwisting velocity at baseline showed positive correlation with E/E' (r=0.30, p<0.05) (B).
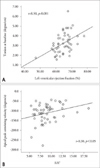
Fig. 6
LV torsion (A), apical peak twisting velocity (B) and apical peak untwisting velocity (C) during exercise echocardiography. LV torsion increases during exercise and recovery. However, apical peak twisting velocity increases during exercise and decreases at recovery phase and also absolute value of apical peak untwisting velocity increases during exercise and decreases at recovery phase.
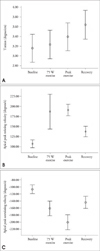
Fig. 7
The correlation between maximal O2 consumption and apical peak twisting velocity at peak exercise. Maximal O2 consumption (MVO2, mL/min/kg) correlated with apical peak twisting velocity at peak exercise (r=0.5, p<0.001).
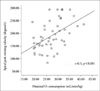
Fig. 8
The Feasibility of left ventricular torsion. Measurements of left ventricular torsion were feasible in 51/54 (94%) at baseline, 49/54 (90%) at 75 W exercise, 43/54 (80%) at peak exercise and 52/54 (96%) at recovery, respectively.
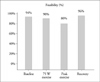
Table 2
Echocardiographic parameters
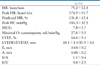
Values are expressed as means±standard deviation. E: peak transmitral E wave velocity, A: peak transmitral A wave velocity, E': peak mitral annular E velocity. HR: heart rate, BP: blood pressure, METs: metabolic equivalents, LVEF: left ventricular ejection fraction, LVEDD: left ventricular end diastolic dimension, LVESD: left ventricular end systolic dimension
Acknowledgement
The present study was supported by grants from the Korean Society of Echocardiography (07-05). We thank Seok In Lee, BS, Eon Ok Shim, RDCS, Kyeong Soon Kim, RDCS, Ok Ryun Kim, RDCS, Mi Jeong Ryu, RN, for his or her excellent technical assistances.
References
1. Rothfeld JM, LeWinter MM, Tischler MD. Left ventricular systolic torsion and early diastolic filling by echocardiography in normal humans. Am J Cardiol. 1998. 81:1465–1469.


2. Notomi Y, Maureen G, Martin-Miklovic MG, Oryszak SJ, Shiota T, Deserranno D, Popovic ZB, Garcia MJ, Greenberg NL, Thomas JD. Enhanced ventricular untwisting during exercise a mechanistic manifestation of elastic recoil described by Doppler tissue imaging. Circulation. 2006. 113:2524–2533.
3. Takeuchi M, Nishikage T, Nakai H, Kokumai M, Otani S, Lang RM. The assessment of left ventricular twistin anterior wall myocardial infarction using two-dimensional speckle tracking imaging. J Am Soc Echocardiogr. 2007. 20:36–44.


4. Tischler M, Niggel J. Left ventricular systolic torsion and exercise in normal hearts. J Am Soc Echocardiogr. 2003. 16:670–674.


5. Notomi Y, Setser RM, Shiota T, Martin-Miklovic MG, Weaver JA, Popović ZB, Yamada H, Greenberg NL, White RD, Thomas JD. Assessment of left ventricular torsional deformation by Doppler tissue imaging validation study with tagged magnetic resonance imaging. Circulation. 2005. 111:1141–1147.


6. Notomi Y, Lysyansky P, Setser RM, Shiota T, Popovic ZB, Martin-Miklovic MG, Weaver JA, Oryszak SJ, Greenberg NL, White RD, Thomas JD. Measurement of ventricular torsion by two-dimensional ultrasound speckle tracking imaging. J Am Coll Cardiol. 2005. 45:2034–2041.


7. Helle-Valle T, Crosby J, Edvardsen T, Lyseggen E, Amundsen BH, Smith HJ, Rosen BD, Lima JAC, Torp H, Ihlen H, Smiseth OA. New noninvasive method for assessment of left ventricular rotation speckle tracking echocardiography. Circulation. 2005. 112:3149–3156.


8. Nakai H, Takeuchi M, Nishikage T, Kokumai M, Otani S, Lang RM. Effect of aging on twist-displacement loop by 2-dimensional speckle tracking imaging. J Am Soc Echocardiogr. 2006. 19:880–885.


9. Takeuchi M, Nakai H, Kokumai M, Nishikage T, Otani S, Lang RM. Age-related changes in left ventricular twist assessed by two-dimensional speckle-tracking imaging. J Am Soc Echocardiogr. 2006. 19:1077–1084.


10. Kim HK, Sohn DW, Lee SE, Choi SY, Park JS, Kim YJ, Oh BH, Park YB, Choi YS. Assessment of left ventricular rotation and torsion with twodimensional speckle tracking echocardiography. J Am Soc Echocardiogr. 2007. 20:45–53.


11. Reuss CS, Moreno CA, Appleton CP, Lester SJ. Doppler tissue imaging during supine and upright exercise in healthy adults. J Am Soc Echocardiogr. 2005. 18:1343–1348.


12. Thomas JD, Picard MH, Yoerger DM, Wood MJ. Myocardial adaptation to short-term high-intensity exercise in highly trained athletes. J Am Soc Echocardiogr. 2006. 19:1280–1285.


13. Yun KL, Niczyporuk MA, Daughters GT, Ingels NB, Stinson EB, Alderman EL, Hansen DE, Miller DC. Alterations in left ventricular diastolic twist mechanics during acute human cardiac allograft rejection. Circulation. 1991. 83:962–973.


14. Davis KL, Mehlhorn U, Schertel ER, Geissler HJ, Trevas D, Laine GA, Allen SJ. Variation in tau, the time constant for isovolumic relaxation, along the left ventricular base-to-apex axis. Basic Res Cardiol. 1999. 94:41–48.


15. Ommen SR, Nishimura RA, Appleton CP, Miller FA, Oh JK, Redfield MM, Tajik AJ. Clinical utility of Doppler echocardiography and tissue Doppler imaging in the estimation of left ventricular filling pressures: a comparative simultaneous Doppler-catheterization study. Circulation. 2000. 102:1788–1794.


16. D'Souza KA, Mooney DJ, Russell AE, MacIsaac AI, Aylward PE, Prior DL. Abnormal septal motion affects early diastolic velocities at the septal and lateral mitral annulus, and impacts on estimation of the pulmonary capillary wedge pressure. J Am Soc Echocardiogr. 2005. 18:445–453.