Abstract
This study aimed to evaluate the antioxidant activity of Korean raspberry (Rubus coreanus Miq.) fruits, which are grown widely in the southwest area of Korea. Freeze-dried Rubus coreanus (RC) fruits and green tea (GT) were used to obtain water extracts (RCE and GTE, respectively). The total phenolic contents of the dried RC and GT were 126µmol CE (catechin equivalent)/g and 360µmol CE/g, respectively. The free radical scavenging activity of RCE and GTE was expressed as SC50 (50% scavenging capacity) and compared. The scavenging activity of RC toward DPPH, superoxide, and hydroxyl radicals was lower than that of GT. The inhibitory activity of RCE and GTE on AAPH-induced lipid peroxidation was also analyzed. In contrast with the free radical scavenging activities, the lipid peroxidation-inhibiting activity of RC was superior to that of GT. These results suggest that RCE contains effective scavengers of peroxyl radicals. In summary, RC contains less phenolics than does GT. Although RC has lower activity for scavenging DPPH, superoxide, and hydroxyl radicals than does GT, its activity to inhibit peroxyl radical-lipid peroxidation was higher. These results suggest that RC could be used as a valuable natural source of antioxidants.
The flowers, leaves, fruits, and roots of plants contain numerous antioxidants, such as carotenoids, thiols, vitamins, flavonoids, and phenolic compounds. It has been reported that fruits and vegetables contain many of these antioxidants and that ingestion of them prevents or decreases the death rate from cancer and cardiac disorder by reducing oxidative stress.1-5 Further health effects provided by their ingestion include decreased blood pressure, higher immunity, detoxified pollutants or contaminants, and suppressed inflammation.6,7 The chemical composition of antioxidants from plants mainly includes phenol compounds, such as flavonoids like anthocyanin.8
Flavonoids have various biological functions other than antioxidant activity, such as anti-cancer, antibacterial, anti-viral, and anti-inflammatory actions; enzyme inhibition; and alteration of the immune system.9-11
Based upon their antioxidant activity, flavonoids were recently shown to actively prevent and treat various diseases such as cancer, ischemic injury of the heart and brain, and AIDS.12-15 After mice were fed extracts of Physalis angulata flower and Rosa hybrida flower for 3 weeks, the activities of superoxide dismutase and catalase were increased and lipid peroxidation was inhibited in blood serum. Specifically, the concentration of HDL cholesterol was increased in the blood serum of mice fed extract of Rosa hybrida flower.16 Therefore, specific methods of how to analyze antioxidants and measure their activities in various plants have been studied.
Rubus coreanus Miq. (RC), a deciduous broadleaf shrub belonging to the family Rosaceae, is limited in growth to the far-east Asian countries (South Korea, China, and Japan).17 Until now, the biological function of constituents of Rubus, such as RC, were studied solely for their antioxidant and anti-inflammatory activities.18-21 In addition, anti-osteoporosis, anti-dementia, anti-stomach disorder, anti-rheumatism, and anti-naphylaxsis activities of RC have been reported.20,22-24 However, the use of RC as a functional health food is usually based on older oriental medical literature and therefore is not proved scientifically.
Rubus coreanus Miq. (RC), a deciduous broadleaf shrub belonging to the family Rosaceae, is limited in growth to the far-east Asian countries (South Korea, China, and Japan).17 Until now, the biological function of constituents of Rubus, such as RC, were studied solely for their antioxidant and anti-inflammatory activities.18-21 In addition, anti-osteoporosis, anti-dementia, anti-stomach disorder, anti-rheumatism, and anti-naphylaxsis activities of RC have been reported.20,22-24 However, the use of RC as a functional health food is usually based on older oriental medical literature and therefore is not proved scientifically.
Accordingly, this study aimed to investigate the antioxidant activity and reactive oxygen species (ROS)-scavenging activity of RC as compared with green tea (GT).
FCR (Folin-Ciocalteu Reagent), catechin, DPPH (2,2-diphenyl-1-picrylhydrazyl), ascorbate, EGCG ((-)-epigallocatechin gallate), PC (L-α-phosphatidylcholine), AAPH (3,2-azobis (2-amidinopropane) dihydrochloride), PMS (phenazine methosulfate), NBT (nitroblue tetrazolium), TBA (thiobarbituric acid), TCA (trichloroacetic acid), and MDA (malondialdehyde) were purchased from Sigma (St. Louis, MO, USA). CCA (coumarin-3-carboxylic acid) was purchased from Fluka (Buchs, Switzerland). High-grade GT was purchased at a store.
RC fruits were collected from Gochang, Korea. Aqueous extracts of RC and GT were prepared by the following method. RC fruits were frozen overnight at -80℃ and then dried by using a lyophilizer (Hanil Science Industrial Co., Incheon, Korea). Dried fruits (5 g) and dried green tea (5 g, Hankook Tea Co., Gwangju, Korea) were homogenized in distilled water (200 mL) for 5 min by use of a Polytron homogenizer (model T-25B, IKA Korea Co, Anyang, Korea). The homogenates were shaken for 20 min at 80℃ in a water bath and centrifuged at 20,000 rpm for 20 min at 4℃. Supernatants were individually collected and frozen overnight at -80℃, followed by drying with the lyophilizer. The weight of the lyophilized water extracts, RCE and GTE, were about 1.85 g and 2.00 g, respectively. RCE and GTE were dissolved in distilled water at a concentration of 10 mg/mL and stored at 4℃.
The phenol content of RCE and GTE was measured with FCR.25 Aliquots of diluted RCE (200µL) and GTE (200µL) were mixed with 10% FCR (1 mL) in test tubes. The mixture was left to react for 3 min at room temperature, followed by the addition of 7.5% Na2CO3 (0.8 mL) and incubation for 2 h in a shaking water bath. After incubation, the absorbance of the mixture was measured at 760 nm by use of a UV-spectrophotometer (model UV-1650PC, Shimadzu, Japan). The phenolic content was quantified from the standard curve plotted with authentic catechin and expressed as µM CE (catechin equivalent) or mmol CE/g (dry weight).
Liposomes were prepared by following the method of Motoyama et al.26 PC (20 mg) was dissolved in a mixture (2 mL) of chloroform and methanol (2:1). The PC solution was dried in a rotary evaporator (Eyela N-N Series, Tokyo Rikakikai Co., Tokyo, Japan) under nitrogen purging. The lipid membrane was stripped by shaking with 20 mL of phosphate buffer solution (50 mM potassium phosphate/0.1 M KCl, pH 7.4). Liposomes were made by ultrasonication (20 kHz) for 15 s at 4℃ under nitrogen purging. The amount of phospholipid in each liposome was evaluated by measuring the Pi (phosphorus) concentration according to the Ames method.27
The antioxidant activity of RCE and GTE was analyzed by measuring the scavenging of DPPH radicals.28 A mixture containing 250 µL of 0.004% DPPH dissolved in ethanol and diluted with distilled water was mixed with 20µL of prediluted CFE in a 96-well plate and incubated at 37℃ for 30 min. Scavenging of DPPH radicals by the antioxidant was analyzed by measuring the decrease in the absorbance at 517 nm with a microplate reader (model µQuant, Bio-Tek Instruments, USA). The DPPH-scavenging capacity of each sample was compared to ascorbic acid and EGCG, as positive controls. Antioxidant activity was expressed as the concentration of each sample that decreased the radicals by half (SC50, 50% scavenging capacity).
Superoxide radicals (O2-) generated by autooxidation of PMS were quantified by the NBT reduction method.29 The reaction mixture (total volume, 1.0 mL) containing 50 mM potassium phosphate (pH 7.4) buffer, 0.5 mM PMS, 0.15 mg Pi/ml PC liposomes, 0.1 mM NBT, and various concentrations of either EGCG, GTE, or CFE was incubated in a shaking water bath at 37℃ for 1 h. The supernatant obtained after centrifugation was analyzed for a decrease in absorbance at 560 nm with a spectrophotometer (model UV- 1650PC, Shimadzu, Japan).
Hydroxyl radicals (‧OH) generated by Fenton reaction of ascorbate and Cu (II) ions were quantified by 7-OHCCA (7-hydroxycoumarin-3-carboxylic acid) generation from CCA.30 The reaction mixture (total volume, 250µL) containing 20 mM potassium phosphate (pH 7.4) buffer, 500µM ascorbate, 50µM CuCl2, 0.1 mM CCA and various concentrations of either EGCG, GTE, or RCE was added to a microplate and incubated in a dark place at room temperature for 1 h. The reaction was stopped by the addition of 10 mM Tris base buffer (pH 9.0). The amount of generated 7-OHCCA was analyzed by a fluorescence microplate reader (Fluoroskan Ascent FL Type 374, Labsystems, Finland). The excitation wavelength was 380 nm and the emission wavelength was 460 nm.
Lipid peroxidation was measured by using the modified TBA assay method of Buege and Aust.31 Lipid peroxidation was induced by peroxyl radicals derived from AAPH, and inhibition mediated by EGCG, GTE, or RCE in PC liposomes was investigated. To induce lipid peroxidation, PC liposomes (0.3 mg/ mL) and 500µM AAPH were added to 50 mM potassium phosphate buffer (pH 7.4). This reaction mixture (total volume: 1.0 mL) was treated with EGCG, GTE, or RCE and then incubated with shaking for 2 h at 37℃. To stop the reaction after incubation, each reaction mixture was maintained in ice. Two milliliters of TBA mixture (0.375% (w/v) TBA, 15% (w/v) TCA, and 0.25 N HCl) was mixed with the reaction by vortexing, followed by heating for 15 min at 100℃. The heated reaction mixture was allowed to cool in tap water and was then centrifuged at 2,500 rpm for 10 min. Absorbance of the supernatant was read at 535 nm with a spectrophotometer (model UV- 1650PC, Shimadzu, Japan). The degree of LPO was expressed as the amount of MDA produced per unit amount of Pi in liposomes (nmol/µmol Pi) and was calculated by using the molecular extinction coefficient (EM = 1.56 × 105) of MDA.
About 1.85 g of RCE was prepared from 5 g of dried RC fruits and was dissolved in distilled water to a concentration of 10 mg/mL. The amount of phenol groups in the stock solution was quantified from the standard curve plotted previously with authentic catechin and was expressed as the concentration of CE phenol groups (Table 1). The phenol content of dried RC was 126µmol (36.5 mg) CE/g. The phenol content of RCE was 340µmol (98.6 mg) CE/g. Therefore, 1 mmol of CE phenol groups was contained in approximately 7.94 g of dried RC or about 2.94 g of RCE, and 1 g of CE phenol groups was contained in approximately 27.4 g of dried RC or about 10.1 g of RCE. The phenol content of dried GT and GTE was measured by the same methods and were found to be 360µmol CE/g and 890µmol CE/g, respectively. An analysis of the phenol group content represented as GAE (gallic acid equivalents) from a gallic acid standard curve found the phenol content of RC to be 33.4 mg GAE/g and that of GT to be 95.4 mg GAE/g (data not shown). These results show that the content of phenol groups was less in RC than in GT, producing a ratio of about 1/2.9~1/2.6.
The scavenging of DPPH radicals by RCE was compared with that of GTE, as well as some well-known antioxidants such as ascorbic acid and EGCG (Fig. 1). Antioxidant activity was expressed as the concentration of antioxidant that scavenged freeradicals by 50% (SC50). The SC50 of ascorbic acid, EGCG, GTE, and RCE was 30.16µM (5.31µg/mL), 6.52µM (2.99µg/mL), 15.93µM CE (44.29µg dry GT/mL), and 17.68µM CE (140.4µg dry RC/mL), respectively. Therefore, the relative DPPH-scavenging activities were in the order of ascorbic acid<RCE<GTE<EGCG. The DPPH-scavenging activity of RCE was especially lower than that of GTE by about 0.9-fold (Table 2). The SC50 of GT and of RC was higher than that of ascorbic acid and of EGCG per dry weight/unit volume. This may be because ascorbic acid and EGCG are single compounds, whereas GT and RC are mixed compounds. Furthermore, the SC50 of RC was 3-fold higher than that of GT (Table 2).
Superoxide radicals (O2-) produced by the autooxidation of PMS were scavenged by EGCG, GTE, and RCE. Their scavenging activities were analyzed and compared by measuring the formazan produced in the reduction of NBT (Fig. 2). The SC50 was 29.17µM (13.37µg/mL) for EGCG, 59.33µM CE (164.81µg dry GT/mL) for GTE, and 103.06µM CE (818.30µg dry RC/ml) for RCE. The superoxide radical-scavenging activity of RCE was lower than that of EGCG and GTE (Table 2).
Hydroxyl radical-scavenging activities of EGCG, GTE, and RCE were compared with one another (Fig. 3). Radicals were produced by a metal-catalyzed oxidation system composed of ascorbic acid and Cu (II). The Sc50 of EGCG, GTE, and RCE was 29.92µM (13.72µg/mL), 39.34µM CE (109.34µg dry GT/mL), and 35.39µM CE (280.87µg dry RC/mL), respectively. As expected, the superoxide radical-scavenging activity of RCE was lower than that of EGCG and GTE (Table 2).
Inhibition of lipid peroxidation by EGCG, GTE, or RCE was compared (Fig. 4). Lipid peroxidation was induced with peroxyl radicals derived from AAPH and was measured by the TBA assay. The SC50 of EGCG, GTE, and RCE was 0.34µM (0.16µg/mL), 0.70µM CE (1.94µg dry GT/mL), and 0.52µM CE (1.70µg dry RC/mL), respectively. Furthermore, the SC50 values of the lipid peroxidation-inhibition activity in dry weight per unit volume were compared (Table 2); that of RCE was lower than that of EGCG, but higher than that of GTE.
Polyphenol compounds have various physiological functions such as antioxidant activity. Thousands of these compounds have been identified in plants, and many studies aim to find new polyphenol compounds from plants and elucidate their functions.
This study investigated the antioxidant activity of RCE compared with GTE, which is a well-known antioxidant source. The phenol content of RC (126µmol CE/g) was only about one third that of GT (360µmol CE/g). Other reports have detailed the phenol content of some Rubus species fruits from the USA. Wang and Lin18 reported the phenol contents of water extracts of some berries (that is, blackberry, black raspberry, red raspberry, and strawberry) to be 4.00~21.66 mg GAE/g. When the phenol content is expressed as GAE, that of RC was 33.4 mg GAE/g and that of GT was 95.4 mg GAE/g. These results suggest that the phenol content of RC was higher than that of a similar species from the USA. Wada and Ou19 reported the phenol content of methanol extracts of various berries (evergreen blackberries, red raspberries, boysenberries, marion berries, and black raspberries) to be 30.94~37.65 mg GAE/g dry weight. These results show that methanol extract contains provably more phenol compounds than water extract. However, we used water extract in the present study because of the obvious relevance of evaluating fruits as a functional food. Wang and Lin18 also reported that phenol compounds in Rubus species are higher in the leaves (47.2~129.2 mg GAE/g) than in fruits. Therefore, further insights could be gained by extracting polyphenol compounds from leaves of RC.
The free radical-scavenging activities of RC, expressed as SC50, were compared with those of GT (Table 2). When antioxidant activities are expressed as dry weight per unit volume, a 2.5~5-fold higher amount of RC than of GT is required to attain a similar SC50 when scavenging DPPH, superoxide, or hydroxyl radicals. However, when antioxidant activities are expressed as the molarity of CE, nearly similar amounts of dried extracts of RC and GT were needed to scavenge DPPH (1.11-fold) and hydroxyl radical (0.9-fold), but much more dried extract of RC than of GT was needed to scavenge superoxide radical (1.74-fold). That is, RC and GT showed nearly similar activities in scavenging DPPH and hydroxyl radicals, but RC showed much less activity than GT in scavenging superoxide radical.
By the way, the lipid peroxidation inhibition by RC was slightly stronger than that by GT when expressed as both the molarity of CE and the dry weight per unit volume. This result means that RC might have better peroxyl radical-scavenging activity than GT because lipid peroxidation was induced by peroxyl radicals. It is quite possible that each antioxidant shows different scavenging activities to various radicals.
These results show that the antioxidant activity of the polyphenols of RC is almost equipotential to that of GT. However, RC itself has a relatively lower content of antioxidant compounds than does GT. Therefore, the amount of RC required may be 2.5~5 fold higher than that of GT in order to produce a similar level of scavenging activity. Last, although the antioxidant activity of Rubus species fruits is lower than that of GT, they have an advantage upon ingestion. A daily intake of GT should be limited because of high caffeine content. However, daily intake of Rubus species of fruits would have a much lower limit.
Figures and Tables
Fig. 1
Dpph Radical-scavenging Activity (A) And Sc50 (B) Of Gte And Rce. The Concentrations Of Gte And Rce Were Expressed As Catechin Equivalent (µm Ce). Each Value Is A Mean±s.D. (N=3). Sc50 Of Ascorbate, 30.16 Mm Ce; Sc50 Of Each Substance Was As Follows: Egcg, 6.52 Mm Ce; Gte, 15.93 Mm Ce; Rce, 17.68 Mm Ce. Egcg, (-)-epigallocatechin Gallate; Gte, Water Extract Of Green Tea; Rce, Te Water Extract Of Rubus Coreanus Fruits; Sc50, 50% Scavenging Capacity Of Total Radicals; Ce, Catechin Equivalent.
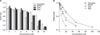
Fig. 2
Superoxide radical-scavenging activity (A) and Sc50 (B) of GTE and RCE. Superoxide radicals were generated from the reaction of 0.5 mM PMS and 0.15 mg Pi/mL PC liposomes. The concentrations of GTE and RCE were expressed as catechin equivalent (µM CE). Each value is a mean±S.D. (n=3). Sc50 of each substance was as follows: EGCG, 29.17 µM CE; GTE, 59.33 µM CE; RCE, 103.06 µM CE. Abbreviations: EGCG, (-)-Epigallocatechin gallate; GTE, water extract of green tea; RCE, water extract of Rubus coreanus fruits; Sc50, 50% scavenging capacity of total radicals; CE, catechin equivalent.
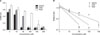
Fig. 3
Hydroxyl radical-scavenging activity (A) and Sc50 (B) of RCE and GTE. Hydroxyl radicals were generated by a mixed-function oxidation system containing 0.5 mM ascorbate and 50 µM Cu (II). Concentrations of GTE and RCE were expressed as catechin equivalent (µM CE). Each value is a mean±S.D. (n=3). Sc50 of each substance was as follows: EGCG, 29.92 µM CE; GTE, 39.34 µM CE; RCE, 35.39 µM CE. EGCG, (-)-Epigallocatechin gallate; GTE, water extract of green tea; RCE, water extract of Rubus coreanus fruits; Sc50, 50% scavenging capacity of total radicals; CE, catechin equivalent.
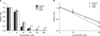
Fig. 4
Lipid peroxidation-inhibiting activity (A) and Sc50 (B) of RCE and GTE. Lipid peroxidation of PC liposomes was induced with 500 µM AAPH at 37℃ for 1 h. Concentrations of GTE and RCE were expressed as catechin equivalent (µM CE). Each value is a mean±S.D. (n=3). Sc50 of each substance was as follows: EGCG, 0.34µM CE; GTE, 0.70µM CE; RCE, 0.52µM CE. EGCG, (-)-Epigallocatechin gallate; GTE, water extract of green tea; RCE, water extract of Rubus coreanus fruits; Sc50, 50% scavenging capacity of total radicals; CE, catechin equivalent; MDA, malondialdehyde.
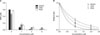
Acknowledgments
This work was supported by a research grant from The Research Institute of Medical Sciences, Chonnam National University (2008-CURIMS-DR006).
References
1. Ames BM, Shigenaga MK, Hagen TM. Oxidants, antioxidants, and the degenerative diseases of aging. Proc Natl Acad Sci USA. 1993. 90:7915–7922.


2. Dragsted LO, Strube M, Larsen JC. Cancer-protective factors in fruits and vegetables: biochemical and biological background. Pharmacol Toxicol. 1993. 72:Suppl 1. 116–135.


3. Cao G, Sofic E, Prior RL. Antioxidant capacity of tea and common vegetables. J Agric Food Chem. 1996. 44:3426–3431.


4. Velioglu YS, Mazza G, Gao L, Oomah BD. Antioxidant activity and total phenolics in selected fruits, vegetables, and grain products. J Agric Food Chem. 1998. 46:4113–4117.


5. Heber D. Vegetables, fruits and phytoestrogens in the prevention of diseases. J Postgrad Med. 2004. 50:145–149.
6. Ascherio A, Rimm EB, Giovannucci EL, Colditz GA, Rosner B, Willett WC, et al. A prospective study of nutritional factors and hypertension among US men. Circulation. 1992. 86:1475–1484.


7. Sacks FM, Kass EH. Low blood pressure in vegetarians: effects of specific foods and nutrients. Am J Clin Nutr. 1988. 48:3 Suppl. 795–800.


8. Cao G, Sofic E, Prior RL. Antioxidant and prooxidant behavior of flavonoids: structure-activity relationships. Free Radic Biol Med. 1997. 22:749–760.


9. Havsteen B. Flavonoids, a class of natural products of high pharmacological potency. Biochem Pharmacol. 1983. 32:1141–1148.


10. Middleton E Jr, Kandaswami C. Effects of flavonoids on immune and inflammatory cell functions. Biochem Pharmacol. 1992. 43:1167–1179.


11. Das DK. Naturally occurring flavonoids: structure, chemistry, and highperformance liquid chromatography methods for separation and characterization. Methods Enzymol. 1994. 234:410–420.
12. Haramaki N, Aggarwal S, Kawabata T, Droy-Lefaix MT, Packer L. Effects of natural antioxidant ginkgo biloba extract (EGB 761) on myocardial ischemia-reperfusion injury. Free Radic Biol Med. 1994. 16:789–794.


13. Rump AF, Schüssler M, Acar D, Cordes A, Theisohn M, Rösen R, et al. Functional and antiischemic effects of luteolin-7-glucoside in isolated rabbit hearts. Gen Pharmacol. 1994. 25:1137–1142.


14. Wu TW, Fung KP, Zeng LH, Wu J, Hempel A, Grey AA, et al. Molecular properties and myocardial salvage effects of morin hydrate. Biochem Pharmacol. 1995. 49:537–543.


15. Oyama Y, Chikahisa L, Ueha T, Kanemaru K, Noda K. Ginkgo biloba extract protects brain neurons against oxidative stress induced by hydrogen peroxide. Brain Res. 1996. 712:349–352.


16. Choi EM, Hwang JK. Effect of some medicinal plants on plasma antioxidant system and lipid levels in rats. Phytother Res. 2005. 19:382–386.


17. Choi HS, Kim MK, Park HS, Kim YS, Shin DH. Alcoholic fermentation of Bokbunja (Rubus coreanus Miq.) wine. Korean J Food Sci Technol. 2006. 38:543–547.
18. Wang SY, Lin HS. Antioxidant activity in fruits and leaves of blackberry, raspberry, and strawberry varies with cultivar and developmental stage. J Agric Food Chem. 2000. 48:140–146.


19. Wada L, Ou B. Antioxidant activity and phenolic content of Oregon caneberries. J Agric Food Chem. 2002. 50:3495–3500.


20. Choi J, Lee KT, Ha J, Yun SY, Ko CD, Jung HJ, et al. Antinociceptive and antiinflammatory effects of Niga-ichigoside F1 and 23-hydroxytormentic acid obtained from Rubus coreanus. Biol Pharm Bull. 2003. 26:1436–1441.


21. Park JH, Oh SM, Lim SS, Lee YS, Shin HK, Oh YS, et al. Induction of heme oxygenase-1 mediates the anti-inflammatory effects of the ethanol extract of Rubus coreanus in murine macrophages. Biochem Biophys Res Commun. 2006. 351:146–152.


22. Shin TY, Kim SH, Lee ES, Eom DO, Kim HM. Action of Rubus coreanus extract on systemic and local anaphylaxis. Phytother Res. 2002. 16:508–513.
23. Lee KH, Choi EM. Rubus coreanus Miq. extract promotes osteoblast differentiation and inhibits bone-resorbing mediators in MC3T3-E1 cells. Am J Chin Med. 2006. 34:643–654.


24. Nam JH, Jung HJ, Choi J, Lee KT, Park HJ. The anti-gastropathic and anti-rheumatic effect of niga-ichigoside F1 and 23-hydroxytormentic acid isolated from the unripe fruits of Rubus coreanus in a rat model. Biol Pharm Bull. 2006. 29:967–970.


25. Singleton VL, Orthofer R, Lamuela-Raventos RM. Analysis of total phenols and other oxidation substrates and antioxidants by means of Folin-Ciocalteu reagent. Methods Enzymol. 1999. 299:152–178.
26. Motoyama T, Miki M, Mino M, Takahashi M, Niki E. Synergistic inhibition of oxidation in dispersed phosphatidylcholine liposomes by a combination of vitamin E and cysteine. Arch Biochem Biophys. 1989. 270:655–661.


27. Ames BN. Assay of inorganic phosphate, total phosphate and phosphatases. Methods Enzymol. 1966. 8:115–118.
28. Rangkadilok N, Sitthimonchai S, Worasuttayangkurn L, Mahidol C, Ruchirawat M, Satayavivad J. Evaluation of free radical scavenging and antityrosinase activities of standardized longan fruit extract. Food Chem Toxicol. 2007. 45:328–336.


29. Lee MW, Ahn BW, Lee KY, Park BJ, Yang SY, Sull JE. Production of superoxide radical by reaction of phenazine methosulfate with erythrocyte membranes. Chonnam Med J. 1984. 21:389–393.
30. Asplund KU, Jansson PJ, Lindqvist C, Nordström T. Measurement of ascorbic acid (vitamin C) induced hydroxyl radical generation in household drinking water. Free Radic Res. 2002. 36:1271–1276.


31. Buege JA, Aust SD. Microsomal lipid peroxidation. Methods Enzymol. 1978. 52:302–310.