Abstract
Background and Objectives
There are pathophysiologic similarities between calcification and atherosclerosis because both are the product of an active inflammatory process. The aim of this study was to examine the effects of statin treatment on calcification in bovine pericardial tissue valves.
Materials and Methods
Forty Sprague-Dawley rats were randomly divided into 4 groups according to hypercholesterolemia induction and statin intake (Group 1, n=10: normal diet without statin treatment, Group 2, n=10: normal diet with statin treatment, Group 3, n=10: high fat diet without statin treatment, Group 4, n=10: high fat diet with statin treatment). Serum lipid levels were measured just before the experiment and after 4 and 12 weeks. Bovine pericardial tissue valve cusps were surgically implanted in rat dorsal subcutis at 4 weeks. After the surgery, statin was administered daily to Groups 2 and 4. Serum interleukin-6 (IL-6) level was measured at 5 weeks. Cusps were explanted at 12 weeks and calcium levels were determined by atomic absorption spectroscopy.
Results
Mean IL-6 was significantly higher in Group 3 at 5 weeks (7.14, 2.03, 31.70, and 6.90 pg/dL for each group, respectively). Mean calcium level in Group 3 was significantly higher among groups but Group 4 was significantly lower compared to Group 3 and was similar to Group 1, 2 (1.86, 1.92, 2.55, and 1.80 mg/g for each group, respectively, p<0.01).
Since the first implants of heterologous tissue valve prostheses in the 1960s, improvements in tissue valve design and manufacturing have been implemented. Tissue valves have central flow and a high freedom from thrombus formation and usually do not need anticoagulation treatment. However, most tissue valves originate from biologic materials that undergo degenerative processes.1)2) A study of the causes of valve failure leading to reoperation reported that sterile degeneration or primary tissue failure accounted for 74% of bioprosthetic valve failures.3) Degeneration of biologic heart valve prostheses is believed to depend on the mechanical properties of the valve and on immunologic and calcification processes.4) Similarities between the mechanisms and risk factors for atherosclerosis, native valve calcification and postoperative tissue valve degeneration have recently been proposed.5)6) A chronic inflammatory change in the valve leaflet, including infiltration of macrophages and T lymphocytes and lipoprotein deposition in the areas of calcification, has prompted investigation as to whether medical therapy can be applied to slow the progression of valve failure.
This question has driven new research into prevention and treatment for postoperative valve tissue failure. A few studies have shown that the use of statins reduced calcific degeneration of native aortic valves.7)8)9) A few retrospective clinical series have also shown the potential relationship between statins and tissue prosthetic valves for preventing the progression of bioprosthetic valve degeneration.10)11) However, to our knowledge few studies have prospectively analyzed the effects of statin treatment on structural changes to biological tissue in prosthetic cardiac valve substitutes. Therefore, the aim of this study was to evaluate the effects of a specific statin therapy, namely rosuvastatin (Crestor®, HMG-CoA reductase inhibitors, rosuvastatin calcium, AstraZeneca Pharmaceuticals LP, Wilmington, DE, USA), on post-implant tissue changes to commonly used bovine pericardial tissue valve in a rat subdermal implantation model.
This animal study was approved by the Institutional Animal Care and Use Committee of Yonsei University Health System (publication no. 2014-0365, 2014).
Healthy 2 month old Sprague-Dawley rats (n=40) weighing 380.27±13.68 g were used in the present study. The animals were acclimatized for a week. Then, they were divided into four groups according to hypercholesterolemia induction and rosuvastatin intake (n=10): (1) Group 1, normal diet without statin treatment; (2) Group 2, normal diet with statin treatment; (3) Group 3, high cholesterol diet without statin treatment; (4) Group 4, high cholesterol diet with statin treatment. Group 1 and 2 were fed a normal diet for 12 weeks and Group 3 and 4 were fed a diet containing 1.25% cholesterol and 0.5% cholic acid (TD.02028, Harlan Korea Laboratories Co., Ltd, Seoul, Korea) for 12 weeks. All other conditions were kept the same for each group.
Non-fasting blood lipid profile (triglyceride, total cholesterol [Tchol], and low density lipoprotein [LDL] cholesterol) were measured just before the experiment and after 4 and 12 weeks for validation of proper hypercholesterolemia. For blood collection, the animals were anesthetized with 10 mg/kg of xylazine and 30 mg/kg of Zoletil® (Virbac Philippines Inc., Taguig, Philippines). Then, 1.3 mL of blood was aseptically obtained from the subclavian vein and 0.5mL of blood was placed into a heparin tube and another 0.8 mL of blood was placed into a serum separating tube. Plasma samples were obtained by centrifugation at 6000 rpm for 5 min at room temperature. TG, LDL cholesterol, and TC were measured by enzymatic colorimetric assay (Modular analytics, Roche, Germany). Serum samples were obtained by centrifugation at 14000 rpm for 10 min at 4℃ and stored at −70℃ in a deep freezer until further analysis. Serum Il-6 levels were measured at 5 and 12 weeks using enzyme-linked immunosorbent assay kits (Quantikine®, Rat IL-6 Immunoassay kit, R&D Systems, MN, USA).
Commercially available bovine pericardial bioprosthetic heart valves (BHVs; Carpentier-Edward PERIMOUNT Aortic valve®, Edwards Lifesciences LLC, Irvine, CA, USA) were used in this study. Each BHV was rinsed at least 15 minutes with 500 mL of sterile physiological saline solution and transferred to another rinse basin with sterile saline. Each leaflet was then incised along the frame of the BHV using a no.11 surgical blade. Then, a round shaped patch was made using a 4 mm skin biopsy punch (Fig. 1A).
At 4 weeks after hypercholesterolemia induction, the patch was surgically implanted in rat dorsal subcutis. Briefly, each animal was intraperitoneally injected with 10 mg/kg of xylazine, and 30 mg/kg of Zoletil® (Virbac Philippines Inc., Taguig, Philippines). After placing an animal in a ventral recumbent position, a dorsal midline skin incision was made. Then, four trimmed pericardial patches were implanted subcutaneously and fixed with 6-0 polypropylene sutures (Fig. 1B). The subcutaneous layer was sutured with a simple continuous pattern using 5-0 polyglyconate sutures, and the skin was closed with a simple interrupted pattern using 4-0 nylon. For three days after surgery, 20 mg/kg of gentamicin and 1 mg/kg of meloxicam were injected subcutaneously for infection prophylaxis and analgesia, respectively.
From the day after surgery, 20 mg/kg/day of rosuvastatin in 2 mL of normal saline was given to Groups 2 and 4 animals by oral gavage using an 18 gauge feeding tube with adequate restraint for 8 weeks. Body weight was measured every week to update drug dosage. Group 1 and 3 animals were given 2 mL of normal saline only.
Eight weeks after surgery, animals were euthanized by the CO2 inhalation method and implanted samples were collected. A Perkin Elmer Optima 8300 ICP-OES (Norwalk, CT, USA) was used for implant calcium analysis. For ICP-OES analysis, surrounding connective tissue from the implants was cleanly removed then frozen for 24 hours in a −80℃ deep freezer. After removing moisture for 24 hours using a freezing dryer, samples were weighed and then dissolved in 5 mL of aqua regia in a 100-mL beaker. After adding 5 mL of hydrogen peroxide (H2O2), the beaker was slowly heated on a hot plate for 6 hours at 70-80℃ (to dissolve the tissue) and for 5 h at 140-150℃; 5 mL of H2O2 were then added. After adjusting the volume up to 50 mL, the samples were analyzed by ICP-OES.
Samples were fixed in 10% neutralized buffered formalin (BBC Biochemical, WA, USA) and processed using standard methods then embedded in paraffin. The 4-µm-thick sections were stained with hematoxylin (Sigma-Aldrich, MO, USA) and eosin (BBC Biochemical, WA, USA) (H&E). The count of infiltrated cells within the implants was performed blindly by an objective pathologist. Eight pictures of each sample were obtained at x400 magnification and were used for the cell count.
To investigate the expression of BMP-2, and osteopontin (OPN), samples were stored at −70℃ in a deep freezer. Protein was extracted with 500 uL of PRO-PREP® protein extraction solution (Intron Biotechnology, Seongnam, Korea), followed by tissue homogenization using sharp scissors. After 30 minutes on ice, samples were centrifuged at 4℃, 14000 rpm for 10 minutes. Then, the supernatant containing the protein lysates was collected.
Quantification of protein content was performed with a Protein-assay Kit (Bio-rad, CA, USA). For immunoblotting, each 50 µg of total protein extract was mixed with Laemmli sample buffer and transferred to a Mini Protean® TGX™ precast gradient gel (Bio-rad, CA, USA) with DOKDO-MARK™ (Intron Biotechnology, Seoungnam, Korea) as a standard pre-stained protein marker. Gel electrophoresis was performed with a Mini Protean® system (Bio-rad, CA, USA) applying 100 V for 130 minutes. After electrophoresis, proteins were blotted to a methanol pre-activated PVDF membrane applying 230 mAh for 90 minutes using a Mini Trans-Blot® Cell (Bio-Rad, CA, USA). Blotted membranes were then blocked and incubated with anti-BMP-2 antibody (ab6285, 1:1000 dilution, Abcam, Cambridge, UK) and anti-OPN antibody (ab8448, 1:1000 dilution, Abcam, Cambridge, UK) in PBS-T with 5% skimmed milk. Anti-β-actin antibody was used as a loading control (ADI-905-733, 1:2000 dilution, Enzo Lifescience, NY, USA). After incubation with the primary antibodies, membranes were washed with PBS-T buffer three times and then incubated with secondary goat anti-mouse IgG-HRP (ab6789, 1:2000 dilution, Abcam, Cambridge, UK) for anti-BMP-2 antibody and anti-OPN antibody and goat anti-rabbit IgG-HRP (ADI-SAB-300-J, 1:2000 dilution, Enzo Lifescience, NY, USA) for anti-β-actin antibody. Proteins were detected using an enhanced chemiluminescence (ECL) detection reagent for immunoblot analysis (Daeill Lab Service, Seoul, Korea). Subsequently, quantitative analysis was performed with the pixel density analysis method using Image J software (National Institutes of Health, NY, USA). Relatively density was defined as follows: (specific band density/β-actin band density)×100.
All data are expressed as mean±standard deviation. Statistical analyses were performed using GraphPad Prism 5.0 software (GraphPad Software, Inc., San Diego, CA, USA). Normal data distribution was determined using the Shapiro-Wilk test. One-way ANOVA and post hoc Bonferroni tests were used to compare mean blood lipid and Il-6 levels, and the relative density of Western blotting level between groups. Kruskal-Wallis and Mann-Whitney tests were applied to assess overall/pair-wise group mean differences in calcium level and nucleated cell count between groups. A p value<0.05 was considered statistically significant.
Rosuvastatin significantly reduced blood Tchol levels in hypercholesterolemic animals (Table 1). Tchol levels measured just before the experiment were not significantly different between each group (Fig. 2A). However, Tchol levels at 4 weeks in Groups 3 and 4 were significantly higher than those in Groups 1 and 2 (p<0.001, Fig. 2C). In addition, Tchol level at 12 weeks in Group 4 was significantly lower than that in Group 3 (p<0.001, Fig. 2E). The effect of rosuvastatin on blood LDL cholesterol level was also similar. The LDL cholesterol levels at 4 weeks in Groups 3 and 4 were significantly higher than those in Groups 1 and 2 (p<0.001, Fig. 2D), but Group 4 was significantly lower than that of Group 3 at 12 weeks (p<0.001, Fig. 2F). Il-6 level at 5 weeks in Group 3 was significantly higher than Groups 1 (p=0.0012) and 2 (p=0.0012) (Fig. 3A). Il-6 level at 5 weeks in Group 4 was significantly lower than that of Group 3 (p=0.0282, Fig. 3A). There were no statistically significant differences between Groups 1, 2, and 4. The Il-6 level in Group 3 showed the highest average value at 12 weeks, but there were no statistically significant differences between the Groups (Fig. 3B). Rosuvastatin did not affect the animals' body weight change. All of the animals gained weight every week but there were no significant differences between groups (Table 1).
The calcium level was detected in all samples 12 weeks after implantation. The calcium level of Group 3 was significantly higher than Groups 1 (p=0.0246) and 2 (p=0.0240) (Fig. 3C). There was no significant difference in calcium level in the normal diet groups (Group 1 vs. Group 2) regardless of rosuvastatin administration (p=0.62, Fig. 3C). However, rosuvastatin treatment significantly decreased calcium levels in the hypercholesterolemic groups (p=0.0041, Fig. 3C).
Rosuvastatin significantly reduced cellular infiltration into bovine pericardial implants in hypercholesterolemic animals. Microscopic examination showed the implants had stacked eosinophilic collagen fibers (Fig. 4). Numerous cellular infiltrates were predominantly presented around the implants and some of them were also present within the fibers of the implants. Highest cellular infiltrates were observed in Group 3. In the normal diet groups, infiltrated cell counts for Group 2 were significantly lower than Group 1 (p=0.0014, Fig. 4E). Group 4 infiltrate cell count was significantly lower than Groups 1 (p<0.01) and 3 (p<0.001) (Fig. 4E). There was no statistical difference between Groups 2 and 4 (p=0.1641).
The relative band density of each group is presented in Table 1. Western blotting showed that BMP-2 and OPN expressions were significantly increased in hypercholesterolemic animals, Groups 3 and 4 (Fig. 5). However, rosuvastatin significantly suppressed BMP-2 (p<0.05) and OPN (p<0.01) expression in Group 4 compared to Group 3. There was no significant difference between the rosuvastatin and normal diet groups.
This study investigated the effect of rosuvastatin on bovine pericardial aortic tissue valve calcification in a rat subdermal implantation model. The principal findings of our study were that high cholesterol level may be a significant risk factor for bovine pericardial valve calcification. Statin treatment could significantly attenuate calcification of bovine pericardial valve tissue in a subcutaneous rat model with dual anti-calcification and anti-inflammation effects regardless of hypercholesterolemia.
Farivar et al.5) reported for the first time that increased serum cholesterol levels were associated with increased bioprosthetic valve calcification4. Furthermore, patients who had explanted valves showed higher serum cholesterol levels than age-, duration of survival-, and position matched patients with unexplanted valves (189 vs. 163 mg/dL, p<0.001). Finally, the odds ratio for valve explantation was 3.9-fold higher in patients whose serum cholesterol levels were greater than 200 mg/dL. Palta et al.10) also demonstrated that those with a serum cholesterol level greater than 200 mg/dL had a reduced native aortic valve area that was nearly twice that of those with a lower cholesterol level (p=0.04). However, Bellamy et al.12) recently reported that there was no correlation between the rate of aortic stenosis (AS) progression and cholesterol levels in 157 patients with AS. They insisted that progression of AS showed no trend for association with cholesterol level; however, statin treatment was associated with slower progression. Statin treatment induced a decreased degeneration of the aortic valve. In that study, the authors confirmed that AS progressed with a reduction in orifice size of approximately 0.1 cm2/year in patients who didn't receive statin therapy. Shavelle and coworkers13) reported that patients receiving statins had a reduced accumulation of aortic valve calcium, as assessed with electron beam computed tomography, although unfortunately serum cholesterol levels were not collected. However, all these reports were retrospectively reviewed using human cohort comparison studies and the object of the studies was human native aortic valves. In addition, experimental studies investigating the correlation between bioprosthetic calcification and blood lipid level are rare.
Recently, an experimental study using bovine pericardial bioprosthesis in a rat subdermal implantation model showed that atorvastatin treatment attenuated bioprosthesis calcification along with reducing the global extent of inflammatory infiltrates.14) They concluded that these effects could enhance structural preservation of bioprostheses and diminish calcific deposition. However, the correlation between the hypercholesterolemic condition and bioprosthetic calcification wasn't clear because they did not observe hypercholesterolemia. However, our experiment showed that hypercholesterolemia was clearly associated with bioprosthesis calcification. Moreover, our results indicated that statin treatment could prevent post-implant calcification and inflammatory infiltration even in animals under hypercholesterolemic conditions. However, calcification degree was not significantly different between Groups 1 and 2 in our study, as opposed to Lorusso et al.14)'s report. The major predictive factors for this discrepancy could be the differences in animal species and follow-up periods between the studies. Short-term rosuvastatin or long-term atorvastatin studies using the same species will help resolve these discrepancies.
The correlation of increased cholesterol levels with bioprosthetic valve calcification calls for mechanistic hypotheses.15) Eliminating lipids from tissue valves by means of ethanol extraction prevents in vivo calcification.16) It is possible that glutaraldehyde-fixed valves have the appropriate physical characteristics to serve as a nidus for crystallization. Another potential mechanism of calcification is the migration of cells into the valve matrix. Blood-borne progenitor cells that are capable of differentiating into various lineages might have the ability to deposit matrix, which can calcify. Additionally, oxidized LDL cholesterol, which interacts with T lymphocytes and calcium, has been demonstrated in degenerative aortic valve stenosis.17) In those studies, diverse strategies were employed to prevent tissue calcification, including inhibitors of hydroxyapatite formation, calcium diffusion inhibitors, peculiar material processing methods to reduce tissue material to calcification, and improved glutaraldehyde or non-glutaraldehyde tissue fixation.6) However, there have been few studies investigating post-implant treatment for degeneration or calcification inhibition. The hypothesis of pathophysiological similarity among atherosclerosis, bioprosthetic, and native valve degeneration has recently been suggested and calls for new research directions, particularly for novel treatment and prevention options.10)11)18) However, the role of hypercholesterolemia in cardiac valve degeneration remains unclear. Lorusso et al.14) reported a clear reduction in calcium deposition in pericardial tissue explanted from animals on statin therapy, either in terms of micro calcification or global calcium content. This effect, however, was not linked to a hypercholesterolemic state, indicating that calcific degeneration might also be induced in the presence of normal levels of cholesterol or may be induced by inflammatory or immunological-related changes elicited in the host body by the heterologous explant material. To clearly show the effect of statin treatment, we classified animals into 4 groups according to hypercholesterolemia and statin intake to observe the effect of statin treatment on both non-hyperlipidemia and hyperlipidemia. Our results showed a strong effect of statin treatment for preventing hypercholesterolemia and anti-inflammatory reactions that were due to inhibition of cellular infiltrates and IL-6 activation. Also, a marked reduction in calcium deposition was found in both statin treatment groups and BMP-2 and OPN expressions related to calcium metabolism. The pleiotropic effects of statin treatment have been recently highlighted19)20) and characterized as immune modulation, anti-inflammatory actions, cell proliferation inhibition, and a decrease in bone-matrix progenitor cells. In hyperlipidemic animal models, Rajamannan et al.21) showed that diet-induced atherosclerosis caused typical lesions to native aortic valves, whereas the administration of statins in this setting counteracted maladaptive valve change, particularly by reducing cellular proliferation and bone matrix production, but also by mitigating maladaptive immunological responses. Actually, BMP-2 stimulated osteoblastic differentiation. Mundy et al.22) recently used high-throughput screening accompanied by a BMP-2 gene promotor-driven reporter assay and found that statins stimulated the BMP-2 promoter. Statins also increased the number of mouse osteoblasts and amount of new bone formed, similar to that seen with recombinant BMP-2 itself. However, in our experiment, BMP-2 was analyzed in prosthetic valve tissue, not in rat blood stream. Statins can strongly inhibit inflammatory mediators and reactions such as IL-6. Consequently, this anti-inflammatory mechanism might downregulate BMP-2 in valve tissue. Osman et al. suggested that human valve ICs (interstitial cells) were capable of osteoblastic differentiation by potential mediators which could be pharmacologically targeted by statin treatment and statins could cause a downregulation in BMP-2 expression levels.23)
The limitations of this study are the small number of experiments and tissue implantation in a subcutaneous animal model which is different than the circulation model; therefore, our data should be interpreted with caution. The resected prosthetic valve cusp is also already injured by cutting, so its coating membrane continuity was broken before implantation. Thus, this experimental situation might induce cellular infiltration from the host system. This could result in explant valve inflammation and calcification when compared to un-resected valve cusps. Finally, the relationship between tissue valve calcification mitigation in animal model studies and clinical outcomes has not been established.
In conclusion, high cholesterol level may be a significant risk factor for bovine pericardial valve calcification. Statin treatment significantly attenuated calcification of bovine pericardial valve tissue in a subcutaneous rat model and showed dual anti-calcification and anti-inflammation effects regardless of hypercholesterolemia. This result suggests we should consider statin treatment to elongate bioprosthesis durability.
Acknowledgments
This study was supported by a research grant from Korean Society of Cardiology for 201403-04 and the authors report no relationships that could be construed as a conflict of interest.
References
1. Cohn LH, Collins JJ Jr, Rizzo RJ, Adams DH, Couper GS, Aranki SF. Twenty-year follow-up of the Hancock modified orifice porcine aortic valve. Ann Thorac Surg. 1998; 66(6 Suppl):S30–S34. PMID: 9930412.
2. Jamieson WR, Burr LH, Munro AI, Miyagishima RT. Carpentier-Edwards standard porcine bioprosthesis: a 21-year experience. Ann Thorac Surg. 1998; 66(6 Suppl):S40–S43. PMID: 9930414.
3. Schoen FJ. Cardiac valve prostheses: review of clinical status and contemporary biomaterials issues. J Biomed Mater Res. 1987; 21(A1 Suppl):91–117. PMID: 3553196.
4. Colli A, Gherli T, Mestres CA, Pomar JL. Degeneration of native and tissue prosthetic valve in aortic position: do statins play an effective role in prevention? Int J Cardiol. 2007; 116:144–152. PMID: 16828903.
5. Farivar RS, Cohn LH. Hypercholesterolemia is a risk factor for bioprosthetic valve calcification and explantation. J Thorac Cardiovasc Surg. 2003; 126:969–975. PMID: 14566234.
6. Schoen FJ, Levy RJ. Calcification of tissue heart valve substitutes: progress toward understanding and prevention. Ann Thorac Surg. 2005; 79:1072–1080. PMID: 15734452.
7. Novaro GM, Tiong IY, Pearce GL, Lauer MS, Sprecher DL, Griffin BP. Effect of hydroxymethylglutaryl coenzyme a reductase inhibitors on the progression of calcific aortic stenosis. Circulation. 2001; 104:2205–2209. PMID: 11684632.
8. Aronow WS, Ahn C, Kronzon I, Goldman ME. Association of coronary risk factors and use of statins with progression of mild valvular aortic stenosis in older persons. Am J Cardiol. 2001; 88:693–695. PMID: 11564402.
9. Achenbach S, Ropers D, Pohle K, et al. Influence of lipid-lowering therapy on the progression of coronary artery calcification: a prospective evaluation. Circulation. 2002; 106:1077–1082. PMID: 12196332.
10. Palta S, Pai AM, Gill KS, Pai RG. New insights into the progression of aortic stenosis: implications for secondary prevention. Circulation. 2000; 101:2497–2502. PMID: 10831524.
11. Black AE, Sinz MW, Hayes RN, Woolf TF. Metabolism and excretion studies in mouse after single and multiple oral doses of the 3-hydroxy-3-methylglutaryl-CoA reductase inhibitor atorvastatin. Drug Metab Dispos. 1998; 26:755–763. PMID: 9698289.
12. Bellamy MF, Pellikka PA, Klarich KW, Tajik AJ, Enriquez-Sarano M. Association of cholesterol levels, hydroxymethylglutaryl coenzyme-A reductase inhibitor treatment, and progression of aortic stenosis in the community. J Am Coll Cardiol. 2002; 40:1723–1730. PMID: 12446053.
13. Shavelle DM, Takasu J, Budoff MJ, Mao S, Zhao XQ, O'Brien KD. HMG CoA reductase inhibitor (statin) and aortic valve calcium. Lancet. 2002; 359:1125–1126. PMID: 11943265.
14. Lorusso R, Corradi D, Maestri R, et al. Atorvastatin attenuates post-implant tissue degeneration of cardiac prosthetic valve bovine pericardial tissue in a subcutaneous animal model. Int J Cardiol. 2010; 141:68–74. PMID: 19167110.
15. Demer LL. Cholesterol in vascular and valvular calcification. Circulation. 2001; 104:1881–1883. PMID: 11602487.
16. Vyavahare NR, Jones PL, Hirsch D, Schoen FJ, Levy RJ. Prevention of glutaraldehyde-fixed bioprosthetic heart valve calcification by alcohol pretreatment: further mechanistic studies. J Heart Valve Dis. 2000; 9:561–566. PMID: 10947050.
17. Olsson M, Thyberg J, Nilsson J. Presence of oxidized low density lipoprotein in nonrheumatic stenotic aortic valves. Arterioscler Thromb Vasc Biol. 1999; 19:1218–1222. PMID: 10323772.
18. Schmermund A, Möhlenkamp S, Erbel R. The latest on the calcium story. Am J Cardiol. 2002; 90(10C):12L–14L.
19. Mohler ER 3rd, Gannon F, Reynolds C, Zimmerman R, Keane MG, Kaplan FS. Bone formation and inflammation in cardiac valves. Circulation. 2001; 103:1522–1528. PMID: 11257079.
20. Yildirir A, Müderrisoglu H. Non-lipid effects of statins: emerging new indications. Curr Vasc Pharmacol. 2004; 2:309–318. PMID: 15320810.
21. Rajamannan NM, Subramaniam M, Springett M, et al. Atorvastatin inhibits hypercholesterolemia-induced cellular proliferation and bone matrix production in the rabbit aortic valve. Circulation. 2002; 105:2660–2665. PMID: 12045173.
22. Mundy G, Garrett R, Harris S, et al. Stimulation of bone formation in vitro and in rodents by statins. Science. 1999; 286:1946–1949. PMID: 10583956.
23. Osman L, Yacoub MH, Latif N, Amrani M, Chester AH. Role of human valve interstitial cells in valve calcification and their response to atorvastatin. Circulation. 2006; 114(1 Suppl):I547–I552. PMID: 16820635.
Fig. 1
Valve patch implantation model in rat. (A) Implant preparation. Round shaped implant (small box) was made using skin biopsy punch. (B) Gross appearance after patch implantation.
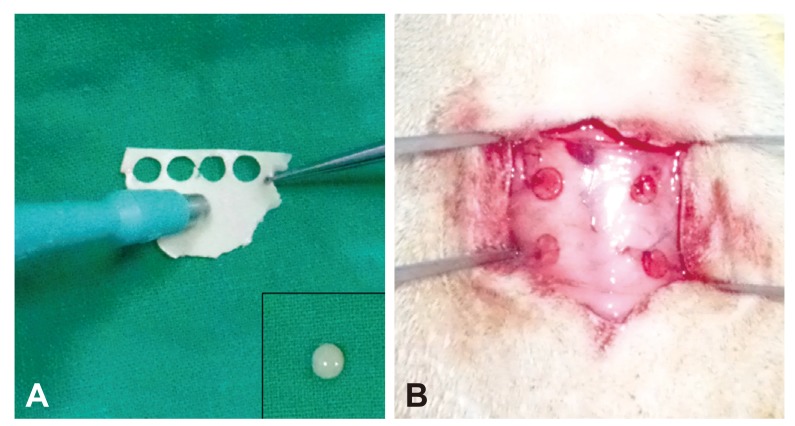
Fig. 2
Blood lipid profiles. Blood Tchol level and LDL level were significantly increased in the hypercholesterol diet intake group. In addition, rosuvastatin significantly lowered Tchol and LDL levels in hypercholesterolemic rats. (A) Blood Tchol level just before experiment, (B) blood LDL level just before experiment, (C) blood Tchol level at 4 weeks, (D) blood LDL level at 4 weeks, (E) blood Tchol level at 12 weeks, (F) blood LDL level at 12 weeks. ***p<0.001, ###p<0.001 when compared with other groups, †††p<0.001 when compared with Group 3. NS: not significant, Tchol: total cholesterol, LDL: low density lipoprotein cholesterol.
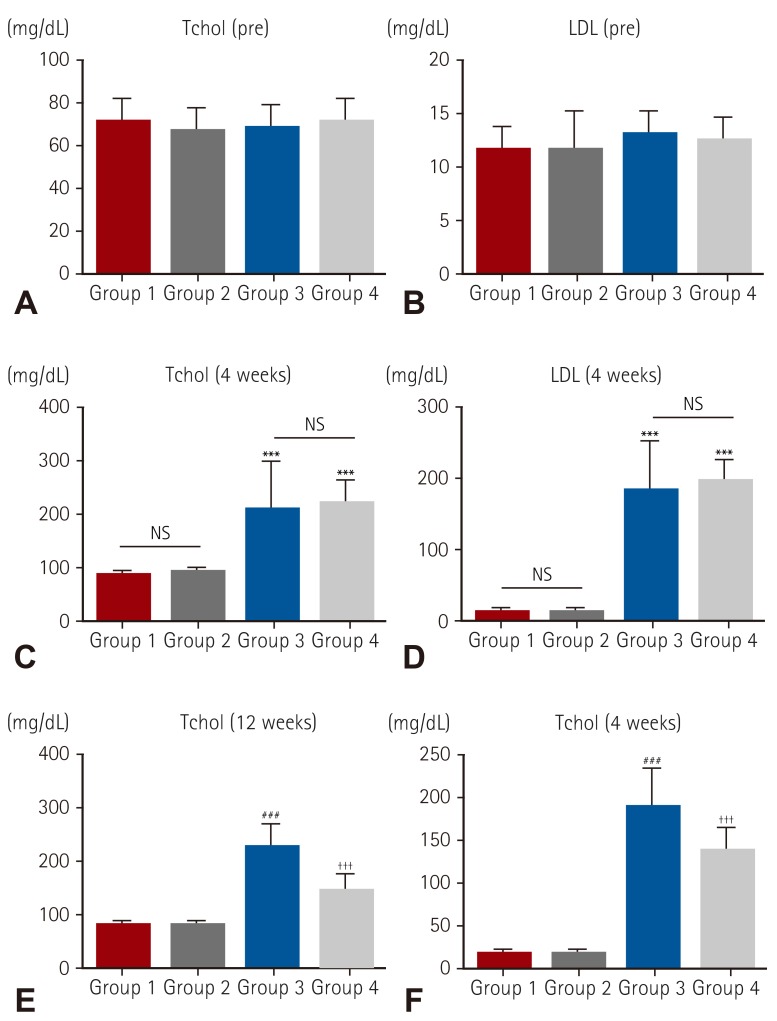
Fig. 3
Effect of rosuvastatin on blood Il-6 level and calcium level in the bovine pericardial implant. Rosuvastatin significantly lowered Il-6 level within 1 week after surgery. In addition, rosuvastatin significantly decreased calcium level in hypercholesterolemic animals. (A) Il-6 level at 5 weeks, (B) Il-6 level at 12 weeks, (C) calcium level at 12 weeks. *p<0.05 when compared with other groups, †p<0.05 when compared with group 3. Il-6: interleukin-6.
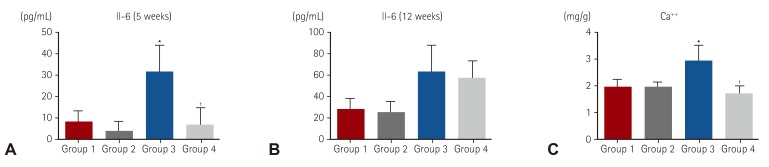
Fig. 4
Histopathological analysis. Cellular infiltration was significantly reduced in rosuvastatin-treated groups. (A) Microscopic image of Group 1, (B) microscopic image of Group 2, (C) microscopic image of Group 3, (D) microscopic image of Group 4, (E) graph of nucleated cell count results. (hematoxylin and eosin stain, x200 magnifi cation) *p<0.05 when compared with Group 1, **p<0.01 when compared with Group 1, †††p<0.001 when compared with Group 3.
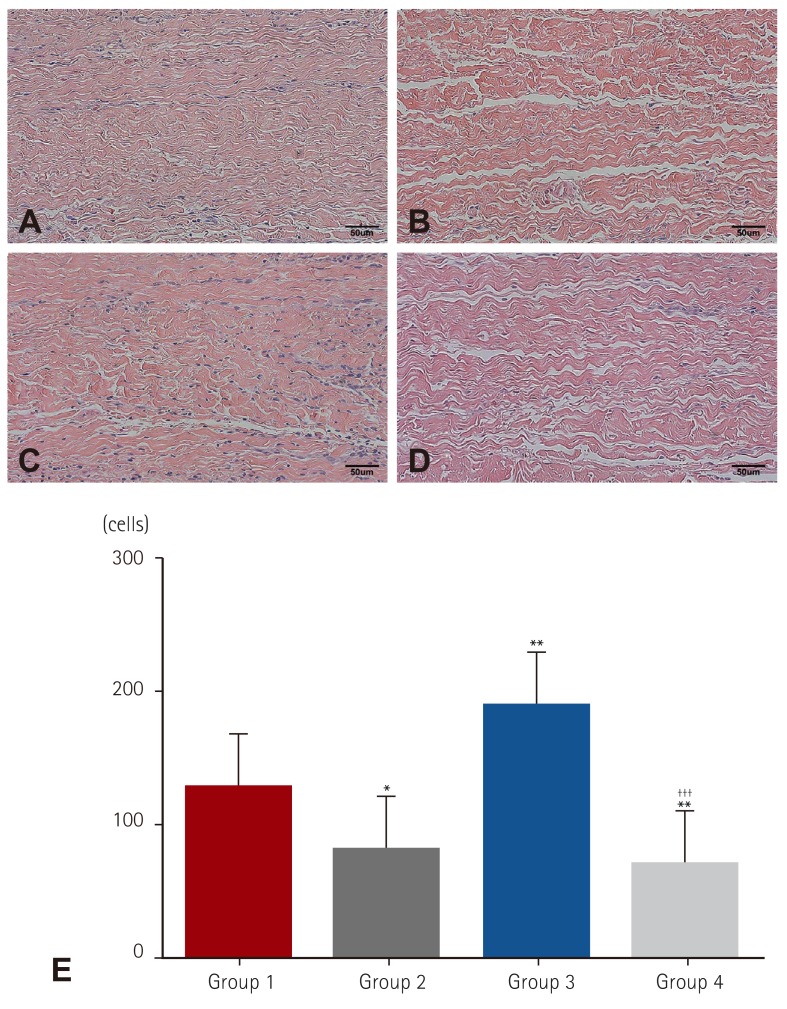
Fig. 5
Expression profiles involved in calcification metabolism. (A) Western blot analysis results. OPN and BMP-2 expressions were significantly lower in the rosuvastatin-treated group than in the normal saline-treated group with hypercholesterolemia. (B) Relative density of BMP-2, (C) relative density of OPN. **p<0.01 when compared with other groups, ††p<0.01 when compared with Group 3. †p<0.05 when compared with Group 3. OPN: osteopontin, BMP-2: bone morphogenic protein-2.

Table 1
Data summary for each experimental group
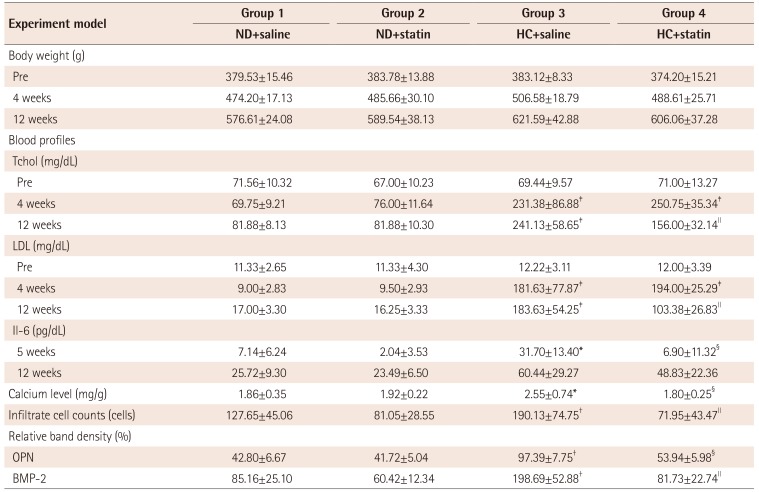
*p<0.05 compared with other groups, †p<0.01 compared with other groups, ‡p<0.001 compared with other groups, §p<0.05 compared with Group 3, ∥p<0.001 compared with Group 3. ND: normal diet, HC: hypercholesterolemic diet, Tchol: total cholesterol, LDL: low density lipoprotein cholesterol, Il-6: interleukin-6, OPN: osteopontin, BMP-2: bone morphogenic protein-2