Abstract
Background and Objectives
This study aims to investigate the significance of changes in the expression 11β-hydroxysteroid dehydrogenase (11β-HSD) and glucocorticoid receptor (GR) for the development of Kawasaki disease (KD).
Subjects and Methods
Real-time polymerase chain reaction was performed to determine the mRNA expression levels of GR and 11β-HSD in peripheral blood monocytes, both in the acute phase of the disease and after treatment. Western blotting was performed to determine the protein expression levels of GR and 11β-HSD.
Results
The expression levels of GRβ, GRβ, and 11β-HSD1 mRNA in the acute phase were significantly higher than levels at baseline (p<0.01) and after treatment (p<0.05). The 11β-HSD2 mRNA levels were lower in the acute phase than in the normal group (p<0.01), and they were significantly higher after treatment than before (p<0.01). Western blot results were consistent with the real-time PCR results. The coronary artery lesion group exhibited significantly different 11β-HSD2 expression levels from that of the group with normal coronary arteries (p<0.01).
Kawasaki disease (KD) is a systemic vasculitis that occurs mainly in infants and children under five years old. The etiology of KD is still unknown, but it is standardly treated with shock therapy of 2 g/kg of intravenous gamma globulin. Approximately 5% of KD children still develop associated coronary artery diseases after treatment, and 8-38% of children exhibit initial intravenous immunoglobulin (IVIG) resistance.1) In recent years, a number of clinical studies have shown that the combination of glucocorticoids (GCs) and IVIG for KD treatment can reduce fever, shorten hospital stays, and improve coronary artery lesions (CAL).2)3)4)5)6)7) GCs are widely used to treat various types of immune vasculitis. At a cellular level, GC must combine with the glucocorticoid receptor (GR) to activate multiple downstream pathways, thus regulating multiple target genes.8) Additionally, the amount of GC that can bind to the GR is not only regulated by the in vivo concentration of GCs and corticosteroid-binding proteins, but also, and more importantly, by the intracellular pre-receptor metabolic enzyme, 11β-hydroxysteroid dehydrogenase (11β-HSD).9) In this study, expression levels of GR and 11β-HSD were measured in the acute phase of disease and after treatment in order to determine their significance in the development of KD and to provide a theoretical basis for the application of GCs in KD treatment.
Patients met the revised diagnostic criteria for KD (5th edition) proposed at the Seventh International Forum on KD.10) The patients had a disease duration of ≤10 days and did not receive any non-steroidal anti-inflammatory drugs or IVIG. The first day of fever was defined as the day of disease onset.
Patients with concomitant congenital heart disease, diabetes, or other inflammatory or autoimmune diseases were excluded.
Thirty KD children who were admitted and diagnosed between August 2013 and May 2014 at Xi'an Children's Hospital were selected for the study. They included 22 boys and eight girls, aged five months to six years with an average age of 1.6 years. The coronary artery normal (CAN) group included 16 patients, and the CAL group included 14 patients. Of these, 29 patients were diagnosed with complete KD, and one was diagnosed with incomplete KD. According to the standards established by cardiovascular and immune experts of the pediatrics branch of the Chinese Medical Association,11) 14 patients in this study exhibited coronary artery dilatation, nine exhibited slight dilation, four had moderate dilation, and one had a huge coronary artery tumor. All patients were administrated IVIG (2 g/kg) treatment within seven to ten days of disease onset. The control group included 30 healthy, age-matched children, including 20 boys and ten girls aged 2.0 to 5.3 years (3.94±0.97 years). None of the healthy children had fevers or any other medical history, and blood samples were obtained during a regular physical examination. There were no statistically significant differences in age and gender between the two groups. This study was conducted in accordance with the tenets of the Declaration of Helsinki and with approval from the Ethics Committee of Xi'an Children's Hospital. Written informed consent was obtained from the guardians of all participants.
Four milliliters of peripheral blood was collected from KD patients in a fasting state on the day following admission and five to seven days after treatment. Samples were anticoagulated using ethylene diamine tetraacetic acid (EDTA) and conventionally centrifuged to obtain monocytes using the stratified fluid density-gradient method. Samples were then divided into two parts (1 mL each) for messenger ribonucleic acid (mRNA) and protein extraction. Blood was only sampled once from the control group using the same methods.
Monocytes were isolated using TRIzol (TaKaRa, Dalian, China) according to the manufacturer's instructions. Total mRNA was extracted, reverse transcription was performed, and the cDNA was quantified. Real-time PCR was performed with actin as the internal control. Primer information is shown in Table 1.
Proteins were extracted from monocytes using conventional methods. All protein from the normal group were mixed for bicinchoninic acid quantification and western blotting. Monoclonal antibodies against 11β-HSD1, 11β-HSD2, and GRβ were purchased from Trask Lane (Danvers, MA, USA), and secondary antibodies were purchased from Zhongshan Co. (Guangzhou, China). Glyceraldehyde-3-phosphate dehydrogenase (GAPDH) was used as an internal reference.
Before treatment, the expression levels of GRα and GRβ mRNA in peripheral blood monocytes of the KD group were 8.76±0.92 and 3.78±0.43, respectively, and were significantly higher than those of the control group (1.00±0.06 and 1.00±0.05) (p<0.01). After IVIG treatment, the expression levels of GRα and GRβ mRNA were significantly reduced (p<0.05) (Fig. 1).
The expression of 11β-HSD1 in peripheral blood monocytes of the KD group was 7.13±0.79, which was significantly higher than that of the control group (1.00±0.07) (p<0.01). The expression of 11β-HSD2 was 0.32±0.05, which was significantly lower than that of the control group (1.00±0.06) (p<0.01). After IVIG treatment, the expression level of 11β-HSD1 was significantly lower than the level observed before treatment (p<0.05), while 11β-HSD2 expression was significantly higher after treatment (p<0.05) (Fig. 2).
Western blotting further confirmed the changes in GR and 11β-HSD expressions before and after IVIG treatment. They were consistent with the real-time PCR analysis results (Fig. 3).
Before treatment, the expression levels of GRα, GRβ, and 11β-HSD1 in peripheral blood monocytes of the CAL group were significantly higher than those of the control group (p<0.01). Expression of 11β-HSD2 was significantly lower in the CAL group than in the control group (p<0.01) (Fig. 4).
Synthetic glucocorticoids have significant anti-inflammatory effects. They inhibit the activity and proliferation of immune inflammatory cells, and they reduce the expression of endothelial cell adhesion molecules.12) Accordingly, they are used to treat a variety of vasculitides. GCs function by binding with the GR, followed by entry into the nucleus to promote or suppress the expression of target genes involved in anti-inflammatory processes. The GR belongs to the nuclear receptor superfamily and is divided into GRα and GRβ. GRα can bind directly to GC and is the main receptor that mediates the anti-inflammatory effects of GC in cells. Its density and affinity are positively correlated with the efficacy of GC. Because GRβ cannot form a complete ligand-binding region, it cannot directly combine with GC. However, GRβ can bind to GRα and form a heterodimer that lacks transcriptional activity but can bind to the GC response element. It can compete as a co-activator with GRα thus inhibiting the activation of GRα. Therefore, GRβ is considered an endogenous GRα antagonist, and the increased expression of GRβ is closely related to hormone tolerance.13)14) Therefore, GR mediates the biological effects of GC and plays an important role in inflammation and stress responses. The results of this study indicate that the expression levels of GRα and GRβ are significantly higher in the acute stage of KD than in the healthy control group. This difference was particularly apparent for GRα. After IVIG treatment, the expression levels of GRα and GRβ mRNA were significantly reduced, but they were still higher than those of the control group, indicating that receptor modulation is an important anti-inflammatory mechanism in KD children. GRα mediates the effects of GC, and the upregulation of GRα mRNA might increase the sensitivity and efficacy of GCs. Therefore, the application of exogenous GC might have anti-inflammatory effects in the early stage of KD.
In recent years, pre-receptor modulation of GCs has received increasing attention. 11β-HSD is the enzyme that regulates GC metabolism, and it includes two main subtypes, 11β-HSD1 and 11β-HSD2. 11β-HSD1 is an active nicotinamide adenine dinucleotide phosphate-dependent dehydrogenase and oxidoreductase that can catalyze the mutual interconversion of active cortisol and non-active cortisone. It is involved in oxidation-reduction in most cells, converting inactive cortisone to active hydrocortisone (cortisol), prior to binding the GR. Therefore, it works synergistically with GC. Generally, it is believed that 11β-HSD1 enhances the intracellular concentration and activity of GC, induces 11β-HSD1 expression, and enhances the protective effects of GC with regards to inflammatory injury of endothelial cells, while 11β-HSD2 exhibits the opposite effects.15)16) Studies have shown that the serum cortisol/cortisone ratio might reflect the activity of this enzymatic reaction. Sano et al.17) found that the serum cortisol and cortisone levels in KD children on admission and after the first dose of IVIG therapy were significantly higher than those in the disease recovery stage. This suggests that 11β-HSD1 activity in KD patients is upregulated and that this upregulation decreases in a time-dependent manner via the reduction of adrenal gland secretion and/or the reduction of 11β-HSD activity, which decreases endogenous cortisol levels. Earlier administration of exogenous GC could have anti-inflammatory effects. The results of this study confirmed that the expression of 11β-HSD1 mRNA was elevated in the acute phase of KD and that the expression of 11β-HSD2 mRNA was inhibited. After treatment, the expression of 11β-HSD1 mRNA was significantly reduced (p<0.05), while 11β-HSD2 mRNA was upregulated (p<0.05), suggesting that changes in 11β-HSD activity are a compensatory response. Inflammatory cytokines and cardiovascular injuries might activate 11β-HSD1, thus enhancing hydrocortisone activity.18) The downregulation of 11β-HSD2 mRNA indicates that the speed of GC inactivation was reduced, corresponding to an increase in its concentration in inflammatory tissue cells. These results indicate that in KD, changes in 11β-HSD are important for regulating the inflammatory response and that early intervention with exogenous GC might help control inflammation and reduce CAL.
The GR mediates the physiological and pharmacological effects of GC, and GC activity might correlate with activity of its receptors and pre-receptor, 11β-HSD. When GRα mRNA is upregulated, GR synthesis increases, and GC can more easily bind to its receptors and exert stronger biological effects, thus enhancing the physiological roles of GC. Intracellular 11β-HSD1 mRNA upregulation could enhance GC activity, and the anti-inflammatory effects of GC can therefore be regulated at the pre-receptor level. Thus the anti-inflammatory effects of GC can be enhanced. The results of this study demonstrate consistent upregulation of GRα mRNA and 11β-HSD1 mRNA in the acute phase of KD. Exogenous GC might upregulate intracellular 11β-HSD1, thus increasing the intracellular biological activity of GCs as well as enhancing the stress and anti-inflammatory abilities of the body. Studies have demonstrated that dexamethasone increases the in vitro activity of 11β-HSD1 and that the application of dexamethasone in early KD could promote the activation of 11β-HSD1 to inhibit acute inflammatory responses.19)20) Therefore, the application of low-dose exogenous GC in early KD might have anti-inflammatory effects.21) The results of this study also show that GRα expression was significantly higher in the CAL group than in the CAN group. This correlated with the presence of CALs, indicating that the expression levels of GRα might reflect the condition of KD patients. This further confirms that the clinical combination of GC and IVIG in refractory KD and severe KD is an effective and safe treatment.
KD is a common childhood vasculitis that is associated with the long-term complication of CALs. The standard therapies for KD include the combination of IVIG and aspirin, which effectively prevents the occurrence of CALs, but some young patients still develop CALs. In recent years, a number of clinical studies have shown that the conventional treatment regimen (GC+IVIG) could be used as an initial treatment strategy for KD to reduce the risk of coronary artery aneurysms. The incidence of CAD in children with KD did not differ between those who received simple hormone GC therapy and those receiving IVIG therapy.22)23) This study provided additional support for the use of GCs to treat acute KD. Because IVIG is highly expensive and is a blood product, it might be an economic burden for a large number of poor people in China. Accordingly, the combination of GC and aspirin could be an economical treatment for KD patients.
This study was limited by its small sample size and the indirect detection of changes in GC concentration.
Figures and Tables
Fig. 1
RT-PCR results of GR mRNA in peripheral blood monocytes in the KD group before and after treatment and in the control group. *p<0.01, **p<0.05. GR: glucocorticoid receptor, mRNA: messenger ribonucleic acid, KD: Kawasaki disease, IVIG: intravenous immunoglobulin.
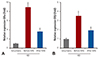
Fig. 2
Expression of 11β-HSD mRNA in peripheral blood monocytes of KD patients before and after treatment. *p<0.01, **p<0.05. 11β-HSD: 11β-hydroxysteroid dehydrogenase, mRNA: messenger ribonucleic acid, KD: Kawasaki disease, IVIG: intravenous immunoglobulin.
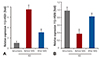
Fig. 3
Western blot results. *p<0.01. GR: glucocorticoid receptor, 11β-HSD: 11β-hydroxysteroid dehydrogenase, GAPDH: glyceraldehyde-3-phosphate dehydrogenase, IVIG: intravenous immunoglobulin.
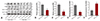
Fig. 4
Expression of 11β-HSD mRNA in monocytes of the CAL group. *p<0.01. and the CAN group. GR: glucocorticoid receptor, CAL: coronary artery lesion, 11β-HSD: 11β-hydroxysteroid dehydrogenase, CAN: coronary artery normal.
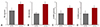
Table 1
Primer sequences of the 11β-HSD1, 11β-HSD2 and GR genes
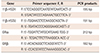
References
1. Weng KP, Ou SF, Lin CC, Hsieh KS. Recent advances in the treatment of Kawasaki disease. J Chin Med Assoc. 2011; 74:481–484.
2. Furukawa T, Kishiro M, Akimoto K, Nagata S, Shimizu T, Yamashiro Y. Effects of steroid pulse therapy on immunoglobulin-resistant Kawasaki disease. Arch Dis Child. 2008; 93:142–146.
3. Kobayashi T, Kobayashi T, Arekawa H. Prednisolone therapy for Kawasaki disease. Nihon Rinsho. 2014; 72:1623–1628.
4. Jibiki T, Kato I, Shiohama T, et al. Intravenous immune globulin plus corticosteroids in refractory Kawasaki disease. Pediatr Int. 2011; 53:729–735.
5. Shirley DA, Stephens I. Primary treatment of incomplete Kawasaki disease with infliximab and methylprednisolone in a patient with a contraindication to intravenous immune globulin. Pediatr Infect Dis J. 2010; 29:978–979.
6. Lim YJ, Jung JW. Clinical outcomes of initial dexamethasone treatment combined with a single high dose of intravenous immunoglobulin for primary treatment of Kawasaki disease. Yonsei Med J. 2014; 55:1260–1266.
7. Kobayashi T, Saji T, Otani T, et al. Efficacy of immunoglobulin plus prednisolone for prevention of coronary artery abnormalities in severe Kawasaki disease (RAISE study): a randomised, open-label, blinded-endpoints trial. Lancet. 2012; 379:1613–1620.
8. Hadoke PW, Iqbal J, Walker BR. Therapeutic manipulation of glucocorticoid metabolism in cardiovascular disease. Br J Pharmacol. 2009; 156:689–712.
9. Coutinho AE, Chapman KE. The anti-inflammatory and immunosuppressive effects of glucocorticoids, recent developments and mechanistic insights. Mol Cell Endocrinol. 2011; 335:2–13.
10. Ayusawa M, Sonobe T, Uemura S, et al. Revision of diagnostic guidelines for Kawasaki disease (the 5th revised edition). Pediatr Int. 2005; 47:232–234.
11. Subspecialty Group of Cardiology, the Society of Pediatrics, Chinese Medical Association. Clinical treatment recommendations of coronary artery lesions in Kawasaki disease. Chin J Pediatr. 2012; 50:746–749.
12. Walker BR. Glucocorticoids and cardiovascular disease. Eur J Endocrinol. 2007; 157:545–559.
13. Meduri GU, Tolley EA, Chrousos GP, Stentz F. Prolonged methylprednisolone treatment suppresses systemic inflammation in patients with unresolving acute respiratory distress syndrome: evidence for inadequate endogenous glucocorticoid secretion and inflammation-induced immune cell resistance to glucocorticoids. Am J Respir Crit Care Med. 2002; 165:983–991.
14. Kino T, Su YA, Chrousos GP. Human glucocorticoid receptor isoform beta: recent understanding of its potential implications in physiology and pathophysiology. Cell Mol Life Sci. 2009; 66:3435–3448.
15. Garbrecht MR, Schmidt TJ, Krozowski ZS, Snyder JM. 11Beta-hydroxysteroid dehydrogenase type 2 and the regulation of surfactant protein A bydexamethasone metabolites. Am J Physiol Endocrinol Metab. 2006; 290:E653–E660.
16. Holmes MC, Sangra M, French KL, et al. 11Beta-hydroxysteroid dehydrogenase type 2 protects the neonatal cerebellum from deleterious effects of glucocorticoid. Neuroscience. 2006; 137:865–873.
17. Sano S, Nakagawa Y, Iwashima S, et al. Dynamics of endogenous glucocorticoid secretion and its metabolism in Kawasaki disease. Steroids. 2010; 75:848–852.
18. Ignatova ID, Kostadinova RM, Goldring CE, Nawrocki AR, Frey FJ, Frey BM. Tumor necrosis factor-alpha upregulates 11beta-hydroxysteroid dehydrogenase type 1 expression by CCAAT/enhancer binding protein-beta in HepG2 cells. Am J Physiol Endocrinol Metab. 2009; 296:E367–E377.
19. Sai S, Esteves CL, Kelly V, et al. Glucocorticoid regulation of the promoter of 11beta-hydroxysteroid dehydrogenase type 1 is indirect and requires CCAAT/enhancer-binding protein-beta. Mol Endocrinol. 2008; 22:2049–2060.
20. Chapman KE, Gilmour JS, Coutinho AE, Savill JS, Seckl JR. 11Beta-hydroxysteroid dehydrogenase type 1--a role in inflammation? Mol Cell Endocrinol. 2006; 248:3–8.
21. D'Attilio L, Díaz A, Santucci N, et al. Levels of inflammatory cytokines, adrenal steroids, and mRNA for GRα, GRβ and 11βHSD1 in TB pleurisy. Tuberculosis (Edinb). 2013; 93:635–641.
22. Ogata S, Ogihara Y, Honda T, Kon S, Akiyama K, Ishii M. Corticosteroid pulse combination therapy for refractory Kawasaki disease: a randomized trial. Pediatrics. 2012; 129:e17–e23.
23. Chen S, Dong Y, Yin Y, Krucoff MW. Intravenous immunoglobulin plus corticosteroid to prevent coronary artery abnormalities in Kawasaki disease: a meta-analysis. Heart. 2013; 99:76–82.