Abstract
Over the past decade, stem cell therapy has been extensively studied for clinical application for heart diseases. Among various stem cells, adipose tissue-derived stem cell (ADSC) is still an attractive stem cell resource due to its abundance and easy accessibility. In vitro studies showed the multipotent differentiation potentials of ADSC, even differentiation into cardiomyocytes. Many pre-clinical animal studies have also demonstrated promising therapeutic results of ADSC. Furthermore, there were several clinical trials showing the positive results in acute myocardial infarction using ADSC. The present article covers the brief introduction, the suggested therapeutic mechanisms, application methods including cell dose and delivery, and human clinical trials of ADSC for myocardial regeneration.
Cellular loss of cardiomyocytes in myocardium mostly through myocardial ischemia or infarction, so-called ischemic cardiomyopathy, causes functional limitation of the remaining heart and finally contributes to heart failure. Ischemic cardiomyopathy caused more than seven million annual deaths worldwide, and patients suffering from this condition lead a very deprived life.1) Although many therapeutic options including revascularization, medication, and even cardiac resynchronization have been adopted for the treatment of patients with ischemic cardiomyopathy, current treatment options are still far from the myocardial regeneration. Therefore, strategies including cell therapy and specific implantation of cells which have potential to induce myocardial regeneration, have been of particular interest. Recently, the international federation for adipose therapeutics and science stated that adipose-tissue derived stem cells (ADSCs) could be one of the ideal biological cellular resources for regenerative medicine applications.2)
During the last several decades, ADSCs have been studied as potential cellular resources for tissue regeneration. ADSCs mean ‘stem cells’ derived from adipose tissue. ADSCs have been known to have potential to differentiate in vitro into adipogenic, osteogenic, chondrogenic, and myogenic cells as well as endothelial cells.3)4)5)6)7)8)9)10) Many studies have identified ADSCs as heterogeneous mesenchymal cells (except adipocytes) derived from adipose tissue.11) ‘Stromal vascular fraction’ have also been used to indicate ADSC. Previous studies showed some discrepancies probably due to different isolation procedures and culturing methods as well as the absence of the standard purifying methods for homogenous cell population. Some cell surface markers were reported to vary at different passage numbers.12) Thus, each ADSC population might need to be assessed for their suitability before clinical applications are ensured. Many recent studies have suggested therapeutic potential of ADSC as cell therapy for many different diseases including myocardial infarction. In this review, we focused on clinical application of ADSC for myocardial regeneration.
In the 1960s, Rodbell13) firstly found the method to isolate cells from rat adipose tissue. In the 2000s, Zuk et al.4) modified and developed ADSC isolation method from human adipose tissue and suggested that ADSC as a promising source for adult multipotent stem cells.14) Recently, Nicoletti et al.15) also developed a new ADSC isolation protocol using enzymatic digestion. D'Andrea et al.16) reported a successful long-term (4 months) and large-scaled ADSC culture. However, it is still necessary to establish a standardized ADSC isolation protocol, which can warrant homogenous ADSC population and reproducible results for clinical application. Although ADSCs have tendency to differentiate into adipocytes,17) they have potential to differentiate into other lineage cells including osteoblasts,18)19) chondrocytes,20) endothelial cells,21)22) and myogenic cells23)
in vitro, which are comparable to bone marrow derived mesenchymal stem cells (BMSCs).21)24) Similar to BMSCs, ADSCs secrete many different kinds of growth factors and cytokines which could contribute in stimulating angiogenesis as well as myocardial regeneration.25)
In the perspectives of cell therapy, ADSCs have several advantages. ADSC can be easily isolated from the adipose tissues which are obtained in relatively large amounts by liposuction. Its efficiency was known to be up to 100 times as compared to BMSCs.26)27)28) Thus, immediate autologous implantation of ADSCs without further in vitro cell culture could be feasible. Detailed comparisons of cellular characteristics between ADSC and BMSC are summarized in Table 1.29)30)31)32)
The mechanisms underlying the effects of ADSCs for myocardial regeneration have not been fully elucidated (Fig. 1). Previously, ADSCs have been reported to have direct differentiation potential into cardiomyocytes in vitro.24) Bai et al.33) demonstrated human ADSC-derived cardiomyocytes expressing connexin 43 and troponin I in mouse acute myocardial infarction (AMI) model, which suggested in vivo cardiomyocyte differentiation of ADSC through fusion-independent mechanism. However, most of the previous in vivo studies on ADSC implantation suggested that only a small fraction of implanted ADSCs contributed into the possible in vivo cardiomyocyte differentiation.34)35) Cell-fusion and mitochondria transfer might also contribute to ADSC-derived cardiomyocytes.36) On the other hand, Yang et al.37) failed to detect ADSC-derived cardiomyocytes although ADSC-derived vascular cells were measured up to 9% of the enhanced angiogenesis. Other study also reported no specific findings of transdifferentiation of ADSC.38)
Predominant suggested mechanisms of ADSCs for myocardial regeneration were their paracrine effects.39)40) ADSCs have been known for secreting many different kinds of proangiogenic, anti-apoptotic and immunomodulatory cytokines and growth factors in the implanted myocardium after ischemic injury.37)41) Productions of various paracrine factors are thought to be enhanced even under hypoxic stress.42) These factors rescue the endangered cardiomyocytes, promote neoangiogenesis and prevent exaggeration of inflammatory response. Finally, ADSCs could reduce scar formation and prevent the adverse cardiac remodeling after ischemic injury.41) In addition, ADSCs have been reported to promote recruitment of other progenitor and stem cells. Kondo et al.43) demonstrated SDF-1 expression in mouse ADSC, and reported that implantation of ADSC into the ischemic hindlimb increased recruitment of circulating endothelial progenitor cells through SDF-1 dependent mechanism.
There have been several studies on comparative analysis between ADSC and other cellular resources. When compared with BMSCs, ADSCs showed similar proangiogenic and immunomodulatory potential. Hao et al.44) demonstrated similar recovery capacity of limb perfusion between ADSCs and BMSCs in a rabbit hindlimb ischemic model. Both cell types also similarly suppressed lymphocyte proliferation and activation elicited by mitogen.45) Recently, several studies have reported that human ADSCs revealed higher capacity to reduce the infarct area in rat AMI models as compared to BMSCs.46)47) Rasmussen et al.46) reported that ADSC implantation showed a superior improvement in left ventricular (LV) systolic function as compared to BMSC implantation. Paul et al.47) also demonstrated superior improvement in cardiac function and less scar formation, which were driven by the immunomodulatory effect of implanted ADSCs. Thus, it is proposed that the unique immune tolerance property of ADSC in addition to its angiogenic property could be the important cellular mechanism for tissue regeneration in allogenic or transgenic transplantation models.
Because most of the ADSCs were rapidly washed out or phagocytosed after implantation, it has been hypothesized that larger number of ADSC implantation might induce more engraftment of the residual ADSCs, which could enhance their therapeutic potential in vivo. However, there have been very few studies regarding neither an optimal implantation dose of ADSCs nor their possible dose-response relationship.48) Naaijkens et al.49) extensively reviewed previous studies regarding the implantation cell number and its functional capacity. In small animal models, 5/13 (38%) studies involving less than 1 million of implanted ADSCs showed a significant infarct size reduction as compared to 12/15 (80%) studies involving more than 1 million of implanted ADSCs. For LV systolic function, 10/17 (59%) studies involving less than 1 million of implanted ADSC showed a significant increase in left ventricular ejection fraction (LVEF) as compared to 12/15 (80%) studies with more than 1 million ADSC implantations. In contrast, studies on the immune-deficient animal model showed less pronounced dose dependencies with respect to increase in blood vessels; whereas in studies involving immune-deficient animal model, significant reduction in infarct size was found in 3 out of 4 (75%) studies using less than 1 million of implanted ADSC as compared with 5 out of 5 (100%) studies using more than 1 million ADSC. The researchers also compared the efficacy of less than 50 million or more ADSC implantation with studies conducted in large animal models by implanting more ADSCs. Similar to the small animal models, studies using larger number of ADSC implantation showed positive results more frequently. Thus, it has been suggested that larger number of ADSC implantation could be beneficial in reducing infarct size and in inducing myocardial recovery. Considering the rapid cellular wash-out in vivo, the optimal delivery method, which is as important as the delivered total ADSC number, to enhance the in vivo engraftment of the implanted ADSC should also be explored.
The delivery method of stem cell implantation is an important determinant for effective cell therapy. Until now, three main different delivery routes of intramyocardial, intravenous, and intracoronary injections have been widely used. Compared to intramyocardial and intracoronary injections, intravenous injection has been contemplated to deliver smaller number of stem cells into the injured myocardium due to the pulmonary first pass effect.50) Nonetheless, intravenous injection of ADSC showed beneficial effects on reducing infarct size and even enhancing cardiac function and blood vessel formation in several animal studies.51)52) Therefore, several human clinical trials have adopted intravenous injection with respect to advantageous and less invasive procedure.
Intracoronary administration of ADSCs has also been considered for its selective delivery into the target myocardium. Firstly, intracoronary administration of ADSC showed similar improvement in myocardial perfusion and LV systolic function as compared to BMSC in porcine AMI model.53) In six large animal studies of ADSC implantation reviewed by Naaijkens et al.,49) 3/4 (75%) studies involving intramuscular injection decreased myocardial infarct size significantly as compared to 1/3 (33%) studies involving intracoronary injection. For LV systolic function, 2/4 (50%) studies involving intramuscular injection increased LVEF significantly as compared to 2/3 (67%) studies relating intracoronary injection. For angiogenesis, 2/4 (50%) studies involving intramuscular injection increased blood vessels significantly as compared to 3/3 (100%) studies dealing with intracoronary injection.54)55)56)57)58) Although there are limited numbers of studies, previous studies suggested that intracoronary injection could be comparable to intramuscular injection for myocardial recovery. Notably, Hong et al.59) compared the cell retention rates of ADSCs between intracoronary and retrograde coronary venous delivery methods in porcine AMI model. Intracoronary injections showed the potential risk of coronary microcirculatory obstructions and early redistribution within 24 hours of injection of ADSCs into pulmonary circulation.
Numerous studies employing small animals have adopted intramyocardial injections. However, intramyocardial injections still show the limitation of cellular engraftment in the infarcted myocardium. Many attempts have been proposed to overcome this obstacle like, a few novel hydrogels have been introduced as potential delivery methods for ADSCs. Wang et al.60) developed chitosan-based hydrogel and implantation of ADSCs mixed with chitosan hydrogel significantly improved LV systolic function as well as cellular engraftment. Notably, chitosan-induced cardiomyocyte differentiation of ADSC both in vitro and in vivo has been demonstrated in their study. Similarly, ADSCs embedded platelet-rich fibrin scaffold revealed further improvement in LV systolic function and LV remodeling as compared to direct implantation of ADSC in rat AMI model.61) Recently, cell sheet technology was introduced to improve cell engraftment after stem cell implantation. Ishii et al.62) developed multilayered ADSC sheet by magnetite tissue engineering technology and transplanted multilayered ADSC sheet epicardially in mouse AMI model. ADSC sheet significantly improved LV systolic function as well as neovascularization in infarct area. Yeh et al.63) developed vascular endothelial growth factor-overexpressing ADSC sheets and applied them in rabbit AMI model with demonstrable near normalized functional recovery of the infarcted myocardium by the epicardial implantation of such sheets. Moreover, application of ADSC sheet improved LV systolic function in a porcine chronic myocardial infarction model.64)
To date, there have been several human clinical trials of ADSCs for ischemic heart diseases (Table 2). 65) Houtgraaf et al.66) firstly reported the safety and efficacy of ADSC in patients with AMI (APOLLO trial; randomized, double-blind placebo-controlled, phase I/IIa study). The study involved infusion of 20 million ADSCs, freshly isolated by liposuction, via infarct related coronary artery into the injured myocardium within 24 hours after primary percutaneous coronary intervention. At 6 months follow-up, cardiac magnetic resonance imaging (MRI) and MIBI-SPECT showed a trend towards the improved LVEF as compared to the placebo group, accompanied by a significant reduction in the perfusion defect and infarct size. Moreover, there were no adverse events during the procedure. The ADVANCE trial, another clinical trial regarding intracoronary injection of ADSCs for patients with AMI, was performed and now final results are awaited.
Perin et al.67) reported the PRECISE trial which was the randomized, placebo-controlled, double-blind trial of transendocardial injection of ADSCs in patients with refractory ischemic cardiomyopathy. Transendocardial injection of ADSCs preserved LV mass and the regional wall motion determined by cardiac MRI and improved myocardial perfusion determined by single-photon emission CT as well as exercise capacity. Other ongoing human clinical trials of ADSCs for ischemic heart diseases include the ATHENA I and II trial (Cytori Therapeutics; intramyocardial injection),68) the MyStromalCell tria (Rigshospitalet, Denmark; allogenic ADSC; intramyocardial injection),69)70) the NCT01502501 trial (Ageless Regenerative Institute; intramyocardial/intravenous injection), the NCT01502514 trial (Ageless Regenerative Institute; intramyocardial/intravenous injection), the NCT01974128 trial (Ageless Regenerative Institute; intramyocardial/intravenous injection), and the NCT01709279 trial (Kanazawa University; intracoronary injection).
During the last several decades, cell therapy using adult stem cells has emerged as a future therapeutics especially for ischemic heart diseases. Among them, ADSCs are very attractive cellular resource for their relatively easy accessibility in large amount, potent differentiation properties, and powerful paracrine effects. Previous animal studies have demonstrated promising results of ADSC implantation for myocardial recovery in ischemic heart diseases, and recent studies focused on the improvement of its efficacy by enhancing their therapeutic potential through genetic modification and cellular priming, in addition to development of novel delivery methods using hydrogels and cell sheet technology. Human clinical trials also support the safety and therapeutic efficacy of ADSCs for myocardial regeneration. Many clinical trials are still ongoing to step forward in clinical research and application.
Acknowledgments
This research was supported by a grant of the Korea Health Technology R&D Project through the Korea Health Industry Development Institute (KHIDI), funded by the Ministry of Health & Welfare (Grant number: HI14C0209), and by a grant of the Basic Science Research Program through the National Research Foundation of Korea (NRF) funded by the Ministry of Science, ICT & Future Planning (No. NRF-2014R1A2A1A11051998), Republic of Korea.
References
1. Moran AE, Forouzanfar MH, Roth GA, et al. Temporal trends in ischemic heart disease mortality in 21 world regions, 1980 to 2010: the Global Burden of Disease 2010 study. Circulation. 2014; 129:1483–1492. PMID: 24573352.
2. Bourin P, Bunnell BA, Casteilla L, et al. Stromal cells from the adipose tissue-derived stromal vascular fraction and culture expanded adipose tissue-derived stromal/stem cells: a joint statement of the International Federation for Adipose Therapeutics and Science (IFATS) and the International Society for Cellular Therapy (ISCT). Cytotherapy. 2013; 15:641–648. PMID: 23570660.
3. Hong SJ, Traktuev DO, March KL. Therapeutic potential of adipose-derived stem cells in vascular growth and tissue repair. Curr Opin Organ Transplant. 2010; 15:86–91. PMID: 19949335.
4. Zuk PA, Zhu M, Mizuno H, et al. Multilineage cells from human adipose tissue: implications for cell-based therapies. Tissue Eng. 2001; 7:211–228. PMID: 11304456.
5. Halvorsen YD, Franklin D, Bond AL, et al. Extracellular matrix mineralization and osteoblast gene expression by human adipose tissue-derived stromal cells. Tissue Eng. 2001; 7:729–741. PMID: 11749730.
6. Erickson GR, Gimble JM, Franklin DM, Rice HE, Awad H, Guilak F. Chondrogenic potential of adipose tissue-derived stromal cells in vitro and in vivo. Biochem Biophys Res Commun. 2002; 290:763–769. PMID: 11785965.
7. Gimble J, Guilak F. Adipose-derived adult stem cells: isolation, characterization, and differentiation potential. Cytotherapy. 2003; 5:362–369. PMID: 14578098.
8. De Francesco F, Tirino V, Desiderio V, et al. Human CD34/CD90 ASCs are capable of growing as sphere clusters, producing high levels of VEGF and forming capillaries. PLoS One. 2009; 4:e6537. PMID: 19657392.
9. Kim JH, Lim IR, Joo HJ, et al. Sphere formation of adipose stem cell engineered by poly-2-hydroxyethyl methacrylate induces in vitro angiogenesis through fibroblast growth factor 2. Biochem Biophys Res Commun. 2015; 468:372–379. PMID: 26498525.
10. Hong SJ, Rogers PI, Kihlken J, et al. Intravenous xenogeneic transplantation of human adipose-derived stem cells improves left ventricular function and microvascular integrity in swine myocardial infarction model. Catheter Cardiovasc Interv. 2015; 86:E38–E48. PMID: 24905889.
11. Dominici M, Le Blanc K, Mueller I, et al. Minimal criteria for defining multipotent mesenchymal stromal cells. The International Society for Cellular Therapy position statement. Cytotherapy. 2006; 8:315–317. PMID: 16923606.
12. Maumus M, Peyrafitte JA, D’Angelo R, et al. Native human adipose stromal cells: localization, morphology and phenotype. Int J Obes (Lond). 2011; 35:1141–1153. PMID: 21266947.
13. Rodbell M. Localization of lipoprotein lipase in fat cells of rat adipose tissue. J Biol Chem. 1964; 239:753–755. PMID: 14154450.
14. Zuk PA, Zhu M, Ashjian P, et al. Human adipose tissue is a source of multipotent stem cells. Mol Biol Cell. 2002; 13:4279–4295. PMID: 12475952.
15. Nicoletti GF, De Francesco F, D’Andrea F, Ferraro GA. Methods and procedures in adipose stem cells: state of the art and perspective for translation medicine. J Cell Physiol. 2015; 230:489–495. PMID: 25294367.
16. D'Andrea F, De Francesco F, Ferraro GA, et al. Large-scale production of human adipose tissue from stem cells: a new tool for regenerative medicine and tissue banking. Tissue Eng Part C Methods. 2008; 14:233–242. PMID: 18781836.
17. Yu G, Floyd ZE, Wu X, et al. Adipogenic differentiation of adipose-derived stem cells. Methods Mol Biol. 2011; 702:193–200. PMID: 21082403.
18. Kroeze RJ, Knippenberg M, Helder MN. Osteogenic differentiation strategies for adipose-derived mesenchymal stem cells. Methods Mol Biol. 2011; 702:233–248. PMID: 21082406.
19. Cheng SL, Yang JW, Rifas L, Zhang SF, Avioli LV. Differentiation of human bone marrow osteogenic stromal cells in vitro: induction of the osteoblast phenotype by dexamethasone. Endocrinology. 1994; 134:277–286. PMID: 8275945.
20. Huang JI, Zuk PA, Jones NF, et al. Chondrogenic potential of multipotential cells from human adipose tissue. Plast Reconstr Surg. 2004; 113:585–594. PMID: 14758221.
21. Planat-Benard V, Silvestre JS, Cousin B, et al. Plasticity of human adipose lineage cells toward endothelial cells: physiological and therapeutic perspectives. Circulation. 2004; 109:656–663. PMID: 14734516.
22. Janeczek Portalska K, Leferink A, Groen N, et al. Endothelial differentiation of mesenchymal stromal cells. PLoS One. 2012; 7:e46842. PMID: 23056481.
23. Mizuno H, Zuk PA, Zhu M, Lorenz HP, Benhaim P, Hedrick MH. Myogenic differentiation by human processed lipoaspirate cells. Plast Reconstr Surg. 2002; 109:199–209. discussion 210-1. PMID: 11786812.
24. Planat-Benard V, Menard C, Andre M, et al. Spontaneous cardiomyocyte differentiation from adipose tissue stroma cells. Circ Res. 2004; 94:223–229. PMID: 14656930.
25. Rehman J, Traktuev D, Li J, et al. Secretion of angiogenic and antiapoptotic factors by human adipose stromal cells. Circulation. 2004; 109:1292–1298. PMID: 14993122.
26. Katz AJ, Tholpady A, Tholpady SS, Shang H, Ogle RC. Cell surface and transcriptional characterization of human adipose-derived adherent stromal (hADAS) cells. Stem Cells. 2005; 23:412–423. PMID: 15749936.
27. Nakagami H, Morishita R, Maeda K, Kikuchi Y, Ogihara T, Kaneda Y. Adipose tissue-derived stromal cells as a novel option for regenerative cell therapy. J Atheroscler Thromb. 2006; 13:77–81. PMID: 16733294.
28. Varma MJ, Breuls RG, Schouten TE, et al. Phenotypical and functional characterization of freshly isolated adipose tissue-derived stem cells. Stem Cells Dev. 2007; 16:91–104. PMID: 17348807.
29. Li CY, Wu XY, Tong JB, et al. Comparative analysis of human mesenchymal stem cells from bone marrow and adipose tissue under xeno-free conditions for cell therapy. Stem Cell Res Ther. 2015; 6:55. PMID: 25884704.
30. Jin HJ, Bae YK, Kim M, et al. Comparative analysis of human mesenchymal stem cells from bone marrow, adipose tissue, and umbilical cord blood as sources of cell therapy. Int J Mol Sci. 2013; 14:17986–18001. PMID: 24005862.
31. Kern S, Eichler H, Stoeve J, Kluter H, Bieback K. Comparative analysis of mesenchymal stem cells from bone marrow, umbilical cord blood, or adipose tissue. Stem Cells. 2006; 24:1294–1301. PMID: 16410387.
32. Ullah I, Subbarao RB, Rho GJ. Human mesenchymal stem cells - current trends and future prospective. Biosci Rep. 2015; 35:pii: e00191.
33. Bai X, Yan Y, Song YH, et al. Both cultured and freshly isolated adipose tissue-derived stem cells enhance cardiac function after acute myocardial infarction. Eur Heart J. 2010; 31:489–501. PMID: 20037143.
34. Chang JC, Lee PC, Lin YC, Lee KW, Hsu SH. Primary adipose-derived stem cells enriched by growth factor treatment improves cell adaptability toward cardiovascular differentiation in a rodent model of acute myocardial infarction. J Stem Cells. 2011; 6:21–37. PMID: 22997843.
35. Wang L, Deng J, Tian W, et al. Adipose-derived stem cells are an effective cell candidate for treatment of heart failure: an MR imaging study of rat hearts. Am J Physiol Heart Circ Physiol. 2009; 297:H1020–H1031. PMID: 19574490.
36. Acquistapace A, Bru T, Lesault PF, et al. Human mesenchymal stem cells reprogram adult cardiomyocytes toward a progenitor-like state through partial cell fusion and mitochondria transfer. Stem Cells. 2011; 29:812–824. PMID: 21433223.
37. Yang D, Wang W, Li L, et al. The relative contribution of paracine effect versus direct differentiation on adipose-derived stem cell transplantation mediated cardiac repair. PLoS One. 2013; 8:e59020. PMID: 23527076.
38. Mazo M, Planat-Benard V, Abizanda G, et al. Transplantation of adipose derived stromal cells is associated with functional improvement in a rat model of chronic myocardial infarction. Eur J Heart Fail. 2008; 10:454–462. PMID: 18436478.
39. Madonna R, Geng YJ, De Caterina R. Adipose tissue-derived stem cells: characterization and potential for cardiovascular repair. Arterioscler Thromb Vasc Biol. 2009; 29:1723–1729. PMID: 19628786.
40. Meliga E, Strem BM, Duckers HJ, Serruys PW. Adipose-derived cells. Cell Transplant. 2007; 16:963–970. PMID: 18293895.
41. Chen L, Qin F, Ge M, Shu Q, Xu J. Application of adipose-derived stem cells in heart disease. J Cardiovasc Transl Res. 2014; 7:651–663. PMID: 25205213.
42. Gnecchi M, Zhang Z, Ni A, Dzau VJ. Paracrine mechanisms in adult stem cell signaling and therapy. Circ Res. 2008; 103:1204–1219. PMID: 19028920.
43. Kondo K, Shintani S, Shibata R, et al. Implantation of adipose-derived regenerative cells enhances ischemia-induced angiogenesis. Arterioscler Thromb Vasc Biol. 2009; 29:61–66. PMID: 18974384.
44. Hao C, Shintani S, Shimizu Y, et al. Therapeutic angiogenesis by autologous adipose-derived regenerative cells: comparison with bone marrow mononuclear cells. Am J Physiol Heart Circ Physiol. 2014; 307:H869–H879. PMID: 25063790.
45. Puissant B, Barreau C, Bourin P, et al. Immunomodulatory effect of human adipose tissue-derived adult stem cells: comparison with bone marrow mesenchymal stem cells. Br J Haematol. 2005; 129:118–129. PMID: 15801964.
46. Rasmussen JG, Frøbert O, Holst-Hansen C, et al. Comparison of human adipose-derived stem cells and bone marrow-derived stem cells in a myocardial infarction model. Cell Transplant. 2014; 23:195–206. PMID: 23211469.
47. Paul A, Srivastava S, Chen G, Shum-Tim D, Prakash S. Functional assessment of adipose stem cells for xenotransplantation using myocardial infarction immunocompetent models: comparison with bone marrow stem cells. Cell Biochem Biophys. 2013; 67:263–273. PMID: 22205499.
48. Hong SJ, Kihlken J, Choi SC, March KL, Lim DS. Intramyocardial transplantation of human adipose-derived stromal cell and endothelial progenitor cell mixture was not superior to individual cell type transplantation in improving left ventricular function in rats with myocardial infarction. Int J Cardiol. 2013; 164:205–211. PMID: 21794931.
49. Naaijkens BA, van Dijk A, Kamp O, Krijnen PA, Niessen HW, Juffermans LJ. Therapeutic application of adipose derived stem cells in acute myocardial infarction: lessons from animal models. Stem Cell Rev. 2014; 10:389–398. PMID: 24577790.
50. Fischer UM, Harting MT, Jimenez F, et al. Pulmonary passage is a major obstacle for intravenous stem cell delivery: the pulmonary first-pass effect. Stem Cells Dev. 2009; 18:683–692. PMID: 19099374.
51. Zhu XY, Zhang XZ, Xu L, Zhong XY, Ding Q, Chen YX. Transplantation of adipose-derived stem cells overexpressing hHGF into cardiac tissue. Biochem Biophys Res Commun. 2009; 379:1084–1090. PMID: 19146824.
52. van Dijk A, Naaijkens BA, Jurgens WJ, et al. Reduction of infarct size by intravenous injection of uncultured adipose derived stromal cells in a rat model is dependent on the time point of application. Stem Cell Res. 2011; 7:219–229. PMID: 21907165.
53. Valina C, Pinkernell K, Song YH, et al. Intracoronary administration of autologous adipose tissue-derived stem cells improves left ventricular function, perfusion, and remodelling after acute myocardial infarction. Eur Heart J. 2007; 28:2667–2677. PMID: 17933755.
54. Yang JJ, Yang X, Liu ZQ, et al. Transplantation of adipose tissue-derived stem cells overexpressing heme oxygenase-1 improves functions and remodeling of infarcted myocardium in rabbits. Tohoku J Exp Med. 2012; 226:231–241. PMID: 22450704.
55. Alt E, Pinkernell K, Scharlau M, et al. Effect of freshly isolated autologous tissue resident stromal cells on cardiac function and perfusion following acute myocardial infarction. Int J Cardiol. 2010; 144:26–35. PMID: 19443059.
56. De Siena R, Balducci L, Blasi A, et al. Omentum-derived stromal cells improve myocardial regeneration in pig post-infarcted heart through a potent paracrine mechanism. Exp Cell Res. 2010; 316:1804–1815. PMID: 20156437.
57. Rigol M, Solanes N, Farre J, et al. Effects of adipose tissue-derived stem cell therapy after myocardial infarction: impact of the route of administration. J Card Fail. 2010; 16:357–366. PMID: 20350704.
58. Mazo M, Hernández S, Gavira JJ, et al. Treatment of reperfused ischemia with adipose-derived stem cells in a preclinical Swine model of myocardial infarction. Cell Transplant. 2012; 21:2723–2733. PMID: 22524986.
59. Hong SJ, Hou D, Brinton TJ, et al. Intracoronary and retrograde coronary venous myocardial delivery of adipose-derived stem cells in swine infarction lead to transient myocardial trapping with predominant pulmonary redistribution. Catheter Cardiovasc Interv. 2014; 83:E17–E25. PMID: 22972685.
60. Wang H, Shi J, Wang Y, et al. Promotion of cardiac differentiation of brown adipose derived stem cells by chitosan hydrogel for repair after myocardial infarction. Biomaterials. 2014; 35:3986–3998. PMID: 24508080.
61. Sun CK, Zhen YY, Leu S, et al. Direct implantation versus platelet-rich fibrin-embedded adipose-derived mesenchymal stem cells in treating rat acute myocardial infarction. Int J Cardiol. 2014; 173:410–423. PMID: 24685001.
62. Ishii M, Shibata R, Shimizu Y, et al. Multilayered adipose-derived regenerative cell sheets created by a novel magnetite tissue engineering method for myocardial infarction. Int J Cardiol. 2014; 175:545–553. PMID: 25023793.
63. Yeh TS, Fang YH, Lu CH, et al. Baculovirus-transduced, VEGF-expressing adipose-derived stem cell sheet for the treatment of myocardium infarction. Biomaterials. 2014; 35:174–184. PMID: 24120047.
64. Ishida O, Hagino I, Nagaya N, et al. Adipose-derived stem cell sheet transplantation therapy in a porcine model of chronic heart failure. Transl Res. 2015; 165:631–639. PMID: 25613060.
65. Pavo N, Charwat S, Nyolczas N, et al. Cell therapy for human ischemic heart diseases: critical review and summary of the clinical experiences. J Mol Cell Cardiol. 2014; 75:12–24. PMID: 24998410.
66. Houtgraaf JH, den Dekker WK, van Dalen BM, et al. First experience in humans using adipose tissue-derived regenerative cells in the treatment of patients with ST-segment elevation myocardial infarction. J Am Coll Cardiol. 2012; 59:539–540. PMID: 22281257.
67. Perin EC, Sanz-Ruiz R, Sanchez PL, et al. Adipose-derived regenerative cells in patients with ischemic cardiomyopathy: The PRECISE Trial. Am Heart J. 2014; 168:88–95.e2. PMID: 24952864.
68. Henry TD, Pepine C, Lambert C, et al. The Athena Trials: Autologous Adipose-Derived Regenerative Cells (ADRCs) for Refractory Chronic Myocardial Ischemia with Left Ventricular Dysfunction. Catheter Cardiovasc Interv. 2017; 89:169–177. PMID: 27148802.
69. Qayyum AA, Haack-Sørensen M, Mathiasen AB, Jørgensen E, Ekblond A, Kastrup J. Adipose-derived mesenchymal stromal cells for chronic myocardial ischemia (MyStromalCell Trial): study design. Regen Med. 2012; 7:421–428. PMID: 22594332.
70. Follin B, Tratwal J, Haack-Sørensen M, Elberg JJ, Kastrup J, Ekblond A. Identical effects of VEGF and serum-deprivation on phenotype and function of adipose-derived stromal cells from healthy donors and patients with ischemic heart disease. J Transl Med. 2013; 11:219. PMID: 24047149.
Fig. 1
Effects of ADSCs on myocardial regeneration. ADSCs can be harvested readily, safely, and abundantly by liposuction. They can be cultured in vitro and differentiated into cardiomyocytes and other mesenchymal cells through direct transdifferentiation or cell-fusion. When transplanted into the infarcted myocardium by IC infusion or IM injection, ADSCs might contribute towards improvement in cardiac function. The suggested mechanisms include their paracrine effects modulating inflammatory response and inducing neoangiogenesis under hypoxic stress. ADSCs: adipose tissue-derived stem cells, IC: intracoronary, IM: intramuscular.
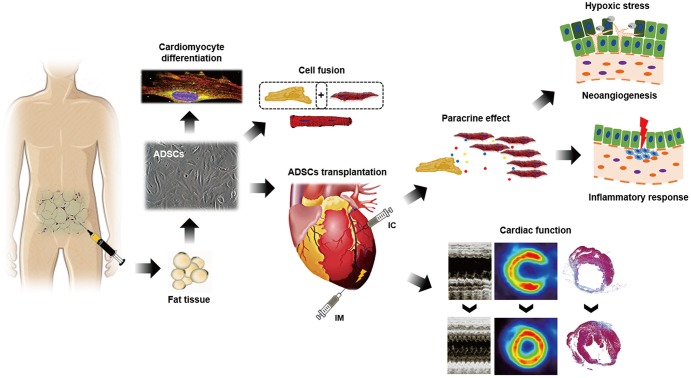
Table 1
Comparison of cellular characteristics between BMSC and ADSC
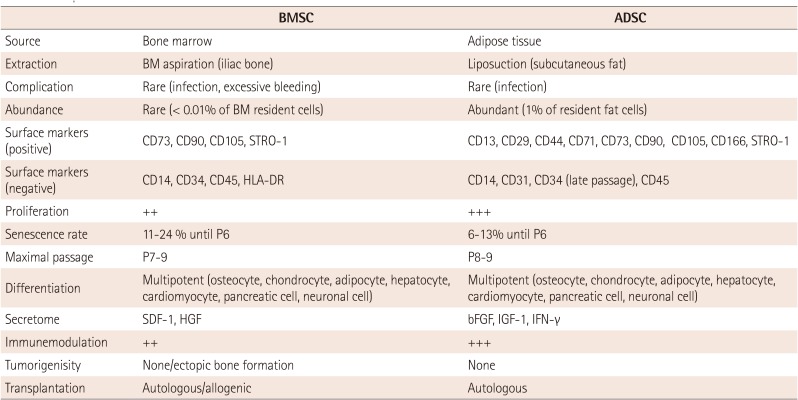
BMSC: bone marrow-mesenchymal stem cell, ADSC: adipose tissue-derived stem cell, BM: bone marrow, STRO-1: stromal cell surface marker 1, HLA-DR: human leukocyte antigen-antigen D related, HGF: hepatocyte growth factor, bFGF: basic fibroblast growth factor, IGF-1: insulin-like gowth factor 1, IFN-γ: interferon gamma
Table 2
Ongoing human clinical trials using ADSCs for the transplantation of cardiovascular diseases
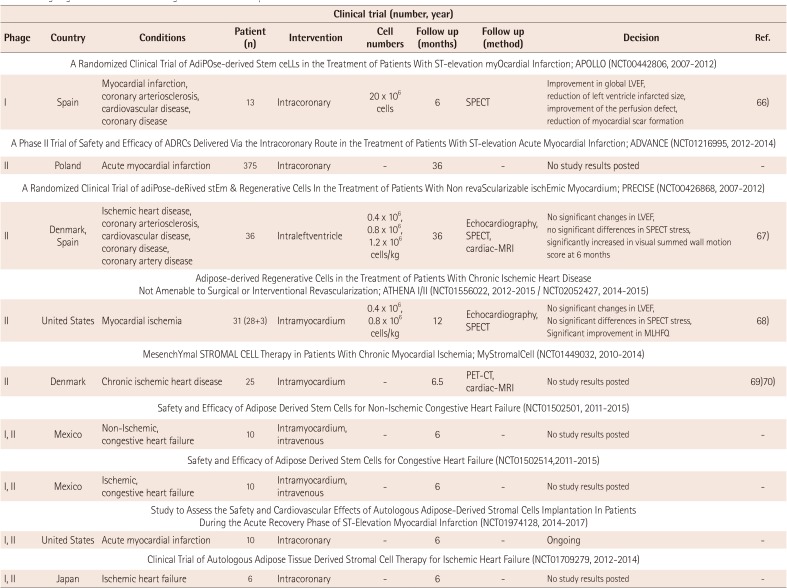
Clinical trial (number, year) | |||||||||
---|---|---|---|---|---|---|---|---|---|
Phage | Country | Conditions | Patient (n) | Intervention | Cell numbers | Follow up (months) | Follow up (method) | Decision | Ref. |
A Randomized Clinical Trial of AdiPOse-derived Stem ceLLs in the Treatment of Patients With ST-elevation myOcardial Infarction; APOLLO (NCT00442806, 2007-2012) | |||||||||
I | Spain | Myocardial infarction, coronary arteriosclerosis, cardiovascular disease, coronary disease | 13 | Intracoronary | 20 × 106 cells | 6 | SPECT | Improvement in global LVEF, reduction of left ventricle infarcted size, improvement of the perfusion defect, reduction of myocardial scar formation | 66) |
A Phase II Trial of Safety and Efficacy of ADRCs Delivered Via the Intracoronary Route in the Treatment of Patients With ST-elevation Acute Myocardial Infarction; ADVANCE (NCT01216995, 2012-2014) | |||||||||
II | Poland | Acute myocardial infarction | 375 | Intracoronary | - | 36 | - | No study results posted | - |
A Randomized Clinical Trial of adiPose-deRived stEm & Regenerative Cells In the Treatment of Patients With Non revaScularizable ischEmic Myocardium; PRECISE (NCT00426868, 2007-2012) | |||||||||
II | Denmark, Spain | Ischemic heart disease, coronary arteriosclerosis, cardiovascular disease, coronary disease, coronary artery disease | 36 | Intraleftventricle | 0.4 × 106, 0.8 × 106, 1.2 × 106 cells/kg | 36 | Echocardiography, SPECT, cardiac-MRI | No significant changes in LVEF, no significant differences in SPECT stress, significantly increased in visual summed wall motion score at 6 months | 67) |
Adipose-derived Regenerative Cells in the Treatment of Patients With Chronic Ischemic Heart Disease Not Amenable to Surgical or Interventional Revascularization; ATHENA I/II (NCT01556022, 2012-2015 / NCT02052427, 2014-2015) |
|||||||||
II | United States | Myocardial ischemia | 31 (28+3) | Intramyocardium | 0.4 × 106, 0.8 × 106 cells/kg | 12 | Echocardiography, SPECT | No significant changes in LVEF, No significant differences in SPECT stress, Significant improvement in MLHFQ | 68) |
MesenchYmal STROMAL CELL Therapy in Patients With Chronic Myocardial Ischemia; MyStromalCell (NCT01449032, 2010-2014) | |||||||||
II | Denmark | Chronic ischemic heart disease | 25 | Intramyocardium | - | 6.5 | PET-CT, cardiac-MRI | No study results posted | 69)70) |
Safety and Efficacy of Adipose Derived Stem Cells for Non-Ischemic Congestive Heart Failure (NCT01502501, 2011-2015) | |||||||||
I, II | Mexico | Non-Ischemic, congestive heart failure | 10 | Intramyocardium, intravenous | - | 6 | - | No study results posted | - |
Safety and Efficacy of Adipose Derived Stem Cells for Congestive Heart Failure (NCT01502514,2011-2015) | |||||||||
I, II | Mexico | Ischemic, congestive heart failure | 10 | Intramyocardium, intravenous | - | 6 | - | No study results posted | - |
Study to Assess the Safety and Cardiovascular Effects of Autologous Adipose-Derived Stromal Cells Implantation In Patients During the Acute Recovery Phase of ST-Elevation Myocardial Infarction (NCT01974128, 2014-2017) | |||||||||
I, II | United States | Acute myocardial infarction | 10 | Intracoronary | - | 6 | - | Ongoing | - |
Clinical Trial of Autologous Adipose Tissue Derived Stromal Cell Therapy for Ischemic Heart Failure (NCT01709279, 2012-2014) | |||||||||
I, II | Japan | Ischemic heart failure | 6 | Intracoronary | - | 6 | - | No study results posted | - |