Abstract
Background and Objectives
Artemisinin and dihydroartemisinin are drugs used to treat malaria. These drugs suppress inflammatory reactions. The aim of this study was to examine the anti-intima hyperplasia effect of a novel drug-eluting stent with artemisinin or dihydroartemisinin in a porcine coronary restenosis model.
Materials and Methods
Pigs were randomized into four groups; in the first, the coronary arteries (20 pigs, a total of 40 coronary arteries, with 10 coronary arteries in each group) was implanted with bare metal stents (BMS, n=10); the second group was given polymer-coated stents (PCS, n=10); the third group was treated with artemisinin-eluting stents (AES, n=10); and the fourth group was given dihydroartemisinin-eluting stents (DAES, n=10). Histopathologic analysis was performed 28 days after stenting.
Results
The injury and fibrin scores among the four groups were not significantly different. However, the internal elastic lamina, lumen area, and neointima area were significantly different. Moreover, the percent area of stenosis (46.2±18.66% in BMS vs. 89.4±10.92% in PCS vs. 83.3±17.07% in AES vs. 36.7±11.20% in DAES, p<0.0001) and inflammation score (1.0 [range: 1.0-1.0] vs. 3.0 [range: 2.25-3.0] vs. 3.0 [range: 1.0-3.0] vs. 2.0 [range: 1.75-3.0] in BMS, PCS, AES, and DAES, respectively; p<0.001) were markedly decreased in the DAES group compared to the PCS group.
The bare metal stent (BMS) was developed to overcome the adverse effects of balloon angioplasty (BA). Compared with BA, the use of BMS decreased the restenosis rates by attenuating arterial contraction and recoiling. However, the restenosis rate remains relatively high at 10-20% and is related to neointimal hyperplasia at the stented lesion.1)2)3)
First generation drug-eluting stents (DESs), such as paclitaxel- or sirolimus-eluting stents, were introduced to reduce the relatively high rate of restenosis associated with BMS. The rate of target lesion revascularization associated with DES was 50-70% less than that of BMS in clinical trials.4)5)
Taxol is isolated from the Pacific yew (Taxus brevifolia), which is a small to medium evergreen plant. A manufacturing company rebranded taxol as paclitaxel. Paclitaxel is used as an anti-neointimal agent for preventing coronary stent restenosis. Thus, the first DES used a plant-based substance.
Artemisinin is extracted from sweet wormwood (Artemisia annua). Artemisinin and dihydroartemisinin, which are semi-synthetic derivatives, are the standard treatment for Plasmodium falciparum malaria. Artemisinin and dihydroartemisinin possess anti-inflammatory effects.
In this study, we investigated the inhibitory effects of artemisinin and dihydroartemisinin, which are naturally occurring artemisinin analogs, on vascular smooth muscle cell proliferation, inflammation, and fibrosis in a porcine coronary restenosis model. Our study may encourage the development of a coronary stent coating with a drug derived from a natural source.
Preparation of artemisinin-eluting, dihydroartemisinin-eluting, and polymer-coated stents
The BMS (Chonnam National University Hospital stent) used in this study was made by laser-cut processing in a cobalt–chromium alloy tube (L605 Co-Cr alloy; Vascotube GmbH, Birkenfeld, Germany) and electropolished to a strut thickness of <70 µm.6)7) An ultrasonic spray method was used to apply coatings to the prepared BMS (3×16 mm). The required amount of poly-l-lactide (PLA) and artemisinin or dihydroartemisinin was dissolved in 5 mL of tetrahydrofuran (THF). Then, the drug solution was dissolved in the polymer solution. The stents to be coated were cleansed with ethanol and distilled water and then vacuum-dried for 24 h. The sprayed liquid consisted of the polymer/drug solution dissolved in THF and diluted to 2% by weight. Coating application required a flow rate of 50 µL/min. The polymer-coated stent (PCS) was coated with only PLA, without any drug. The stents were placed on a mandrel attached to a rotating shaft, coated, and vacuum-dried for 24 h. The surface morphologies of the artemisinin-eluting stent (AES), dihydroartemisinin-eluting stent (DAES), and PCS were then examined.
We used a scratch method under scanning electron microscopy (SNE-1500M; SEC Co., Ltd., Suwon, Korea) and reflection spectrometry (F40; Filmetrics Inc., San Diego, CA, USA) in the wavelength range of 400-850 nm to measure the thickness of the coating layer on each stent. The optical index of refraction was assumed to be n=1.50 of the PLA measurement. To determine the total amount of artemisinin and dihydroartemisinin on the BMS, the coated stent was sonicated in 5 mL of acetonitrile (ACN) for 1 h to dissolve the coated layer with the drug and then analyzed with an ultraviolet spectrophotometer (UV; UV-1800, Shimadzu, Japan) at 241.5 nm. In vitro drug release was measured using a simple shaking method with UV.8)
All stents were expanded to a 3.0-mm diameter. The stents were immersed in 5 mL of phosphate buffered saline (PBS) in colored vials and subjected to 100 rpm shaking at 37℃. The stent was taken out at each designated time point, and the PBS was replaced with fresh solution at specified times. The drugs remaining on the stents were dissolved in ACN and measured with the UV–visible spectrophotometer at 241.5 nm.
The study animals were Yorkshire × Landrace F1 crossbred castrated male swine. Their average age was 7-9 weeks. The procedure for coronary stenting was conducted as described previously.9) The stent was deployed by inflating the balloon (3×20 mm), and the resulting stent-to-coronary artery ratio was 1.3:1. The diameter of the implanted coronary stent (stent-to-artery ratio) was adjusted with reference to the 7 F guiding catheter diameter (2.31 mm). The stented pigs underwent follow-up angiography after four weeks. The pigs were anesthetized on the day of the follow-up with zolazepam and tiletamine 2.5 mg/kg (Zoletil50®, Virbac, Caros, France), xylazine 3 mg/kg (Rompun®, Bayer AG, Leverkusen, Germany), and azaperone 6 mg/kg (Stresnil®, Janssen-Cilag, Neuss, Germany). The pigs were sacrificed with 20 mL of potassium chloride via intracoronary injection under deep anesthesia after follow-up angiography.
The pigs were randomly divided into four groups: BMS (3.0×16 mm, n=10), PCS (3.0×16 mm, n=10), AES (3.0×16 mm, n=10), and DAES (3.0×16 mm, n=10).
A total of 20 pigs (40 coronary arteries) were used in this study. BMS, PCS, AES, or DAES were implanted in the left anterior descending artery and the left circumflex artery of each pig in a randomized manner.
Histopathological evaluation of each artery was performed by an experienced cardiovascular pathologist. The specimens were embedded, and ~3-5 µm thick sections were obtained at ~1 mm intervals and stained with hematoxylin and eosin (H&E) and Carstairs' stain for histological analysis. The histopathological sections were measured using a calibrated microscope, digital video imaging system, and microcomputer program (Visus 2000 Visual Image Analysis System; IMT Tech, San Diego, CA, USA). Borders were manually traced to define the lumen area, the area circumscribed by the internal elastic lamina, and the innermost border of the external elastic lamina (i.e., external elastic lamina area). Morphometric analysis was used to calculate the neointimal area of a given vessel as the measured internal elastic lamina area minus the lumen area. Measurements were made on five cross-sections from the proximal and distal ends and the three midpoints of each stented segment. Histopathological stenosis was calculated as 100× (1– [lesion lumen area/lesion internal elastic lamina area]).10) The harvested stent specimens were stored in formaldehyde solution. A 1.5 mL Eppendorf tube was filled with clay that was formed into a V shape to hold the stent during contrast agent staining. The stents were taken from the solution and placed vertically in the V-shaped opening in the clay. Each stent was fixed in the clay so that it would not move inside the tube. The contrast agent used was omnihexol. One milliliter of the contrast agent was placed in a 5 mL syringe and injected through the opening at the center of the stent. The stent was incubated with the contrast agent overnight and subjected to microcomputed tomography imaging.11) All results were interpreted by two independent pathologists in a blinded fashion.
Arterial injury at each stent strut site was determined by the anatomic structures penetrated by each stent strut. A numeric value was assigned, as previously described by Schwartz et al.10): 0=no injury; 1=break in the internal elastic membrane; 2=perforation of the media; and 3=perforation of the external elastic membrane to the adventitia. The average injury score for each segment was calculated by dividing the sum of the injury scores by the total number of stent struts in the examined section.
The inflammation score for each individual stent strut was graded as follows: 0=no inflammatory cells surrounding the stent strut; 1=light, noncircumferential lymphohistiocytic infiltrate surrounding the strut; 2=localized, moderate-to-dense cellular aggregate surrounding the stent strut noncircumferentially; and 3=circumferential dense lymphohistiocytic cell infiltration of the stent strut. The inflammation score for each cross-section was calculated by dividing the sum of the individual inflammation scores by the total number of stent struts in the examined section.12) Ordinal data for fibrin were collected for each stent section using a scale of 0-3, as previously reported.13)
This animal study was approved by the Ethics Committee of Chonnam National University Medical School and Chonnam National University Hospital (CNU IACUC-H-2013-26) and conformed to the Guide for the Care and Use of Laboratory Animals published by the US National Institutes of Health (NIH Publication No. 85-23, revised 1996).
Statistical analysis was performed with the aid of commercially available software (SPSS version 15; SPSS Inc., Chicago, IL, USA). The data are presented as mean±standard deviation. An unpaired Student's t test was used to compare each stent group. Analysis of variance (ANOVA) was used for comparisons between the four stent groups. Ordinal measurements such as injury score, fibrin score, and inflammation score were analyzed using the Kruskal-Wallis test. Non-parametric results are presented as median and interquartile range. A value of p<0.05 was considered statistically significant.
Roughness and defects of the polymer-coated surface of the stents could affect stent thrombosis and drug release.14)15)
Our findings using the optical microscope (Fig. 1) and SEM (Fig. 2) showed that the coated surfaces were uniform and smooth. Neither bridge nor webbing was observed in any of the stents. The coating thickness using RS was 5-8 µm.
The in vitro elution of artemisinin and dihydroartemisinin on the BMS is shown in Fig. 3. Artemisinin and dihydroartemisinin were released for more than 30 days.
A total of 40 stents (10 AES, 10 DAES, 10 PCS, and 10 BMS) were placed in the left anterior descending and left circumflex arteries of 20 pigs (Table 1). The overall mortality rate was 0% in this study. There was no significant difference in the stent-to-artery ratio among the three stent groups.
All stented coronary arteries showed ISR pattern II (intra-stent) on follow-up coronary angiographic analysis.16)
Injury score (1.0 [range: 1.0-1.0] vs. 1.0 [range: 1.0-2.0] vs. 1.0 [range: 1.0-1.0] vs. 1.0 [range: 1.0-2.0] for BMS, PCS, AES and DAES, respectively; p=NS) and fibrin score (1.0 [range: 1.0-1.0] vs. 1.0 [range: 1.0-2.0] vs. 1.0 [range: 1.0-1.0] vs. 1.0 [range: 1.0-2.0] for BMS, PCS, AES, and DAES, respectively; p=NS) were not significantly different among the four groups. However, internal elastic lamina (4.1±0.82 mm2 vs. 4.9±0.69 mm2 vs. 4.8±0.64 mm2 vs. 4.2±0.41 mm2 for BMS, PCS, AES, and DAES, respectively; p<0.0001), lumen area (1.7±1.27 mm2 vs. 2.2±1.12 mm2 vs. 0.8±0.84 mm2 vs. 2.7±0.56 mm2 for BMS, PCS, AES, and DAES, respectively; p<0.0001), neointimal area (2.0±0.79 mm2 vs. 4.3±0.63 mm2 vs. 4.0±0.92 mm2 vs. 1.6±0.47 mm2 for BMS, PCS, AES, and DAES, respectively; p<0.0001), percent area of stenosis (46.2±18.66% vs. 89.4±10.92% vs. 83.3±17.07% vs. 36.7±11.20% for BMS, PCS, AES, and DAES, respectively; p<0.0001) (Fig. 4), and inflammation score (1.0 [range: 1.0-1.0] vs. 3.0 [range: 2.25-3.0] vs. 3.0 [range: 1.0-3.0] vs. 2.0 [range: 1.75-3.0] for BMS, PCS, AES, and DAES, respectively; p<0.001) (Fig. 4) were significantly different among the four groups (Fig. 5).
The occlusion rate (percent area of stenosis) of stented arteries assessed using micro-CT was significantly lower in the DAES group than in the other groups (55.6±16.84% vs. 86.3±20.64% vs. 87.4±10.44% vs. 41.6±8.96% in the BMS, PCS, AES, and DAES groups, respectively; p<0.0001) (Fig. 6).
This study was conducted to estimate the effect of DES with artemisinin or dihydroartemisinin compared with that of PCS without any drug and BMS in a porcine coronary restenosis model. Our study showed that dihydroartemisinin had suppressive effects on vascular smooth muscle cells (VSMCs) and inflammation.
Paclitaxel, which was previously called taxol, is isolated from the pacific yew (Taxus brevifolia). Paclitaxel blocks progression of the cell-division cycle by stabilizing microtubule formation. Paclitaxel is used as a drug to treat lung, pancreatic, ovarian, breast, and other cancers. Paclitaxel is used as a coronary and peripheral stent coating for preventing restenosis within stented vessels because paclitaxel suppresses VSMC migration and proliferation by inhibiting platelet-derived growth factor in VSMCs.17) The rates of restenosis and target vessel revascularization were significantly lower with paclitaxel-eluting stents than with BMSs in previous clinical research.18)19)
Artemisinin, also known as qinghaosu, is isolated from the Chinese herb Artemisia annua, similar to the isolation of paclitaxel. Artemisinin and dihydroartemisinin (dihydroqinghaosu, arteminol, or DHA) are used to treat Plasmodium falciparum malaria.
In previous studies, artemisinin, as a reactive oxygen species (ROS) scavenger, significantly inhibited inflammation, migration, and proliferation of tumor necrosis factor (TNF)-α-stimulated VSMCs through cell-cycle arrest, the ROS-mediated NF-κB signal pathway, suppression of ERK1/2 phosphorylation and MMP9 expression, and stimulation of the apoptotic pathway. Therefore, artemisinin inhibits neointimal proliferation after vessel injury.20)21)22)
Dihydroartemisinin is an active metabolite of other artemisinin derivatives (artesunate, artemether, etc.).
Dihydroartemisinin attenuates fibrosis through a decrease in transforming growth factor-beta 1, TNF-α, alpha smooth muscle actin, and NF-κB expression and inhibition of inflammatory cell reactions and collagen deposition.23) Moreover, dihydroartemisinin significantly inhibits the inflammatory response by decreasing the release of TNF-α, interleukin (IL)-6, and the inflammatory mediator nitric oxide by suppression of the inducible nitric oxide synthase (iNOS) protein.24)
The anti-inflammatory and anti-neointimal effect of artemisinin was insufficient to suppress vascular injury after polymer-based coronary stent implantation in this experiment. However, dihydroartemisinin has demonstrated a stronger effect than artemisinin. One explanation for this phenomenon is that dihydroartemisinin is the active metabolite.
This study has several limitations. First, the stents were implanted in normal porcine coronary arteries without atherosclerosis and calcification, which is different from human patients with preexisting atherosclerosis, endothelial dysfunction, plaque, and calcification. Second, we did not analyze the degree of re-endothelialization of the injured stented artery. We focused on estimating the inhibition of neointimal proliferation. Third, a longer-term follow-up experiment done after 6 months was not conducted using minipigs, which would be useful in examining drug efficacy on delayed arterial healing, inflammatory reactions, and late-stage stent thrombosis.
Our group will attempt to assess the clinical applications of DAES after a long-term follow-up experiment using minipigs.
In conclusion, DAES showed a suppressive effect on neointima and the inflammatory response at 1 month compared with AES, PCS, and BMS in a porcine coronary restenosis model. Therefore, dihydroartemisinin can be a useful drug that is derived from a natural product for coronary stent coating to inhibit neointimal proliferation and inflammatory reactions after stenting.
Figures and Tables
Fig. 1
Optical microscopy was used to assess uniformity of stent surface. (A) Surface morphology of PCS, (B) AES, and (C) DAES by optical microscopy (×10). PCS: polymer-coated stent, AES: artemisinin-eluting stent, DAES: dihydroartemisinin-eluting stent.
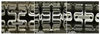
Fig. 2
The SEM pictures indicate uniform coating on stent surface without webbing and bridge. SEM images (magnification, ×50 and ×100) of (A, B) PCS, (C, D) AES, and (E, F) DAES. SEM: scanning electron microscopy, PCS: polymer-coated stent, AES: artemisinin-eluting stent, DAES: dihydroartemisinin-eluting stent.
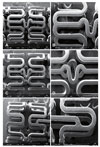
Fig. 3
In vitro release kinetics of artemisinin (dashed line) and dihydroartemisinin (filled line) from the stent over time. AES: artemisinineluting stent, DAES: dihydroartemisinin-eluting stent.
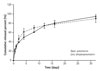
Fig. 4
Representative images of hematoxylin and eosin staining at 4 weeks after stenting. Specimens show implanted BMS (A, ×20), PCS (C, ×20), AES (E, ×20) and DAES (G, ×20). Carstairs' fibrin stain (magnification, ×20) for fibrin infiltration in implanted BMS (B, ×20), PCS (D, ×20), AES (F, ×20), and DAES (H, ×20). BMS: bare metal stent, PCS: polymer-coated stent, AES: Artemisinin-eluting stent, DAES: dihydroartemisinin-eluting stent.
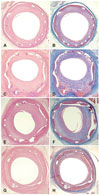
Fig. 5
DAES showed superior neointima and inflammation suppressive effect compare to PCS in graph F and G. (A) Injury score, (B) fibrin score, (C) internal elastic lamina, (D) lumen area, (E) neointimal area, (F) percent area of stenosis, and (G) inflammation score of BMS, PCS, AES, and DAES. (A), (B), and (G) are expressed as median (interquartile range). NS: not statistically significant, BMS: bare metal stent, PCS: polymer-coated stent, AES: artemisinin-eluting stent, DAES: dihydroartemisinin-eluting stent.
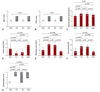
Fig. 6
Micro-CT analysis of in-stent restenosis of BMS, PCS, AES, and DAES. CT: computed tomography, BMS: bare metal stent, PCS: polymer-coated stent, AES: artemisinin-eluting stent, DAES: dihydroartemisinin-eluting stent, OR: occiusion rate.
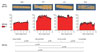
Acknowledgments
This study was supported by a grant from the Korean Society of Cardiology in 2013 (201303-07).
References
1. Fischman DL, Leon MB, Baim DS, et al. A randomized comparison of coronary-stent placement and balloon angioplasty in the treatment of coronary artery disease. Stent Restenosis Study Investigators. N Engl J Med. 1994; 331:496–501.
2. Serruys PW, de Jaegere P, Kiemeneij F, et al. A comparison of balloon-expandable-stent implantation with balloon angioplasty in patients with coronary artery disease. Benestent Study Group. N Engl J Med. 1994; 331:489–495.
3. Cutlip DE, Chauhan MS, Baim DS, et al. Clinical restenosis after coronary stenting: perspectives from multicenter clinical trials. J Am Coll Cardiol. 2002; 40:2082–2089.
4. Stettler C, Wandel S, Allemann S, et al. Outcomes associated with drug-eluting and bare-metal stents: a collaborative network meta-analysis. Lancet. 2007; 370:937–948.
5. Stone GW, Moses JW, Ellis SG, et al. Safety and efficacy of sirolimus- and paclitaxel-eluting coronary stents. N Engl J Med. 2007; 356:998–1008.
6. Bae IH, Lim KS, Park JK, et al. Mechanical behavior and in vivo properties of newly designed bare metal stent for enhanced flexibility. J Ind Eng Chem. 2015; 21:1295–1300.
7. Lim KS, Bae IH, Kim JH, et al. Mechanical and histopathological comparison between commercialized and newly designed coronary bare metal stents in a porcine coronary restenosis model. Chonnam Med J. 2013; 49:7–13.
8. Ma X, Oyamada S, Gao F, et al. Paclitaxel/sirolimus combination coated drug-eluting stent: in vitro and in vivo drug release studies. J Pharm Biomed Anal. 2011; 54:807–811.
9. Lim KS, Park JK, Jeong MH, et al. Biodegradable polymer-based sirolimus coating stent in a porcine coronary restenosis model. Clin Exp Thromb Hemost. 2014; 1:59–65.
10. Schwartz RS, Huber KC, Murphy JG, et al. Restenosis and the proportional neointimal response to coronary artery injury: results in a porcine model. J Am Coll Cardiol. 1992; 19:267–274.
11. Che HL, Bae IH, Lim KS, et al. Suppression of post-angioplasty restenosis with an Akt1 siRNA-embedded coronary stent in a rabbit model. Biomaterials. 2012; 33:8548–8556.
12. Schwartz RS, Edelman E, Virmani R, et al. Drug-eluting stents in preclinical studies: updated consensus recommendations for preclinical evaluation. Circ Cardiovasc Interv. 2008; 1:143–153.
13. Suzuki T, Kopia G, Hayashi S, et al. Stent-based delivery of sirolimus reduces neointimal formation in a porcine coronary model. Circulation. 2001; 104:1188–1193.
14. Lim KS, Park JK, Jeong MH, et al. Comparison of sirolimus loaded PLGA-PEG co-polymer coronary stent and bare metal stent in a porcine coronary restenosis model. Macromol Res. 2014; 22:639–646.
15. Kang SN, Kim SE, Choi J, et al. Comparison of phytoncide with sirolimus as a novel drug candidate for drug-eluting stent. Biomaterials. 2015; 44:1–10.
16. Mehran R, Dangas G, Abizaid AS, et al. Angiographic patterns of instent restenosis: classification and implications for long-term outcome. Circulation. 1999; 100:1872–1878.
17. Sollott SJ, Cheng L, Pauly RR, et al. Taxol inhibits neointimal smooth muscle cell accumulation after angioplasty in the rat. J Clin Invest. 1995; 95:1869–1876.
18. Stone GW, Lansky AJ, Pocock SJ, et al. Paclitaxel-eluting stents versus bare-metal stents in acute myocardial infarction. N Engl J Med. 2009; 360:1946–1959.
19. De Luca G, Dirksen MT, Kelbaek H, et al. Paclitaxel-eluting versus bare metal stents in primary PCI: a pooled patient-level meta-analysis of randomized trials. J Thromb Thrombolysis. 2015; 39:101–112.
20. Cao Q, Jiang Y, Shi J, et al. Artemisinin inhibits tumour necrosis factor-alpha-induced vascular smooth muscle cell proliferation in vitro and attenuates balloon injury-induced neointima formation in rats. Clin Exp Pharmacol Physiol. 2015; 42:502–509.
21. Cao Q, Jiang Y, Shi J, et al. Artemisinin inhibits the proliferation, migration, and inflammatory reaction induced by tumor necrosis factor-alpha in vascular smooth muscle cells through nuclear factor kappa B pathway. J Surg Res. 2015; 194:667–678.
22. Lee KP, Park ES, Kim DE, Park IS, Kim JT, Hong H. Artemisinin attenuates platelet-derived growth factor BB-induced migration of vascular smooth muscle cells. Nutr Res Pract. 2014; 8:521–525.
23. Yang D, Yuan W, Lv C, et al. Dihydroartemisinin supresses inflammation and fibrosis in bleomycine-induced pulmonary fibrosis in rats. Int J Clin Exp Pathol. 2015; 8:1270–1281.
24. Yu WY, Kan WJ, Yu PX, Li MM, Song JS, Zhao F. Anti-inflammatory effect and mechanism of artemisinin and dihydroartemisinin. Zhongguo Zhong Yao Za Zhi. 2012; 37:2618–2621.