Abstract
Background and Objectives
Large-artery stiffness is an independent predictor of cardiovascular disease (CVD), and carotid-femoral pulse wave velocity (cfPWV) is considered the gold standard measure of arterial stiffness. A resting heart rate is an easily measured vital sign that is also associated with CVD morbidity and mortality. Previous studies have reported the significant relationship of a resting heart rate with arterial stiffness as measured by cfPWV only in hypertensive subjects; their relationship in nonhypertensive subjects remains unknown. The present study, therefore, examined their relationship in normotensive subjects.
Subjects and Methods
In 102 healthy Korean Americans between ages 20 and 60 years, their resting heart rate was measured by an automated blood pressure measuring device after a 10 minute rest in the supine position. Arterial stiffness was measured by cfPWV using the SphygmoCor device.
Results
The mean resting heart rate of participants (mean age, 39.64 years; 59% women) was 61.91 bpm (standard deviation [SD], 9.62 bpm) and mean the cfPWV was 6.99 (SD, 1.14) m/s. A multiple regression analysis showed that a resting heart rate is a significant predictor of cfPWV after controlling for age, body mass index, and mean arterial pressure. For one bpm increase of resting heart rate, cfPWV increased approximately 0.02 m/s.
Compelling evidence has been accumulated for the role of arterial stiffness in the development of cardiovascular disease (CVD). When arterial walls lose elastic properties and become stiff, pulse waves that are generated from the heart, reflect back during the late systole period instead of diastolic period, resulting in an augmentation of systolic blood pressure (BP) and left ventricular load.1) Arterial stiffness is identified as an independent predictor of CVD including hypertension2) and left ventricular hypertrophy.1) Since pulse waves travel faster in stiffer arteries, determining pulse wave velocity (PWV) is generally accepted as a simple, noninvasive, and reproducible method of measuring arterial stiffness.3) Carotid-femoral PWV (cfPWV) is considered the 'gold-standard' measure of arterial stiffness because it measures along the aorto-iliac pathway, which makes the largest contribution to the arterial buffering function.3)
Several epidemiological studies demonstrated that heart rate is an independent predictor of development of sustained hypertension,4) atherosclerosis,5) and cardiovascular mortality.6)7) A population-based study conducted in 1780 Japanese participants suggested that even with systolic BP less than 135 mmHg, individuals with a heart rate equal to or more than 70 beats per min (bpm) had a higher risk of cardiovascular mortality; and an increase of 5 bpm in a resting heart rate was associated with a 17% increase in the 10-year risk of cardiovascular mortality after controlling for BP.6) Another longitudinal study on 4695 patients with isolated systolic hypertension demonstrated that individuals with heart rates more than 79 bpm had a 1.89 fold greater risk of mortality than those with a heart rate equal to or less than 79 bpm.7) Despite the prognostic value of a resting heart rate in cardiovascular morbidity and mortality, the mechanism to explain the association of a resting heart rate with CVD has not been fully elucidated. It is suggested that a chronic increase in heart rate may exacerbate structural and functional changes of arterial walls.8) The impact of an elevated heart rate on excessive arterial stiffness may explain the link between resting heart rate to CVD.
Previously, several studies have demonstrated the association between arterial stiffness and resting heart rate among hypertensive subjects. Issues of measuring arterial stiffness,9) small sample size,10)11) subjects' advanced age,11) and group heterogeneity9)12) have been questioned by their results. In particular, the relationship of a heart rate with arterial stiffness remains elusive in nonhypertensive individuals. The present study, therefore, aims to examine the relationship between resting heart rate and arterial stiffness measured by cfPWV in nonhypertensive adults.
After the approval of the institutional review board at the University of North Carolina at Chapel Hill, a total of 120 Korean American adults were recruited from the Southeastern United States by subject recruitment notices posted on bulletin boards and by word of mouth. The inclusion criteria were: (a) aged 21 to 60 years; (b) the absence of cardiovascular conditions (e.g., pacemaker, heart failure, coronary artery disease, valvular heart diseases, or diabetes) or vascular conditions (e.g., peripheral vascular diseases). The exclusion criteria were: (a) pregnant; (b) having irregular cardiac rhythm; (c) taking medication that may change heart rate or blood pressure (e.g., antihypertensive medication, β-blockers, thyroid, hormones, or steroids). Prior to data collection, participants provided informed consent.
Study procedures complied with the European Society of Cardiology's recommendations to standardize subject conditions.3) To minimize the variation caused by circadian rhythm of arterial stiffness, data collection was conducted in each participant's home between 7 and 11 AM. Participants were instructed to refrain from smoking, eating and consuming coffee for at least 3 hours, and drinking alcohol for 10 hours prior to measurement.
Demographic variables included age, sex, current smoking status, and body mass index (BMI). BMI was calculated by dividing weight by squared height (kg/m2). Height and weight were measured with an Accu-Hite portable mounted portable stadiometer (Seca, CA, USA) and a portable electronic scale (Penn Scale, PA, USA).
After participants rested at least 10 minutes in the recumbent position, heart rate and peripheral brachial systolic/diastolic blood pressure (BP) were measured using an automated BP monitoring device, Welch Allyn Vital Signs Monitor 300 Series (Skaneateles Falls, New York, NY, USA). Since an incorrect cuff size is likely to cause an error in BP measurement, the mid-section circumference of the dominant upper arm was measured with a tape measure, and the proper sized cuff was selected according to the upper arm circumference. In the supine position, heart rate and BP were measured in the dominant arm twice with a 2-minute rest period between the measurements, and mean of resting heart rate and BP were calculated.
After measuring BP, pulse wave velocity (PWV) was measured noninvasively at the carotid and femoral arterial sites by using the SphygmoCor system (AtCor Medical, Sydney, AU). The distance from the suprasternal notch to the carotid artery site (d-carotid) and the distance from the suprasternal notch to the femoral artery site (d-femoral) were measured in meters by using a standard measuring tape. The pressure sensitive transducer (tonometer) was applied on the carotid and femoral arterial sites along with three electrocardiogram leads attached on subjects' chest in order to measure the transit time of the pulse wave from the left ventricle to the carotid arterial site (t1) and from the left ventricle to the femoral arterial site (t2), respectively. Carotid-femoral PWV (cfPWV) was calculated as the distance difference between d-femoral and d-carotid divided by the mean difference between t1 and t2.3)
SAS 9.1 software (SAS Institute Inc., NC, USA) was used for statistical analyses. Data are expressed as mean±standard deviation (SD) for normally distributed continuous variables and as frequency (percentage) for categorical variables. A Pearson correlation was used to test bivariate relationships between variables, and a simple linear regression was conducted to examine the relationship between resting heart rate and PWV. Systolic blood pressure (SBP) and diastolic blood pressure (DBP) were highly correlated. To prevent multicollinearity issue, further analysis used the mean arterial pressure (MAP), which reflects both SBP and DBP. Resting heart rate was categorized into quartiles as follows: Q1 <56, 56≤ Q2 <61, 61≤ Q3 <67, and 67≤ Q4 <94. A one-way analysis of covariance (ANCOVA) was used to examine significant differences in cfPWV among four groups of resting heart rates, controlling for age, MAP, and BMI. Additionally, simultaneous multiple regression analysis was performed to identify significant predictors of PWW. All statistical tests were two-tailed and the level of statistical significance was set at p value less than 0.05.
Table 1 presents the characteristics of the study sample and the descriptive results of their physiological measures. A total of 102 subjects comprised of 61 (59.80%) women and 41 (40.19%) men with a mean age of 39.64 (SD: 9.89) years old. After 10 minutes of rest, the mean resting heart rate of this study group was 61.91 (SD 9.62) beats per min (bpm) and the mean cfPWV was 6.99 (SD 1.14) m/s.
The results of the bivariate correlations are presented in Table 2. Resting heart rate was significantly associated with cfPWV (r=0.233, p=0.019), DBP (r=0.261, p=0.008), and MAP (r=0.203, p=0.040). CfPWV showed strong relationships with age (r=0.609, p=0.000), BMI (r=0.277, p=0.005), SBP (r=0.450, p=0.000), DBP (r=0.558, p=0.000), and MAP (r=0.536, p=0.000). A two-dimensional distribution of resting heart rate and cfPWV is displayed in Fig. 1.
Table 3 displays the distribution of participants' resting heart rate and cfPWV for each quartile.
The results of a one-way ANCOVA between heart rate quartiles and cfPWV are presented in Table 4. Test of equality of error variances showed that variances among the four groups are homogenous (p=0.516). An overall difference of cfPWV in four quartiles of resting heart rate after controlling for age, BMI, and MAP was observed but not statistically significant (F=2.51, p=0.063) (Table 4).
The results indicated that the average cfPWV in four groups of resting heart rate were Q1=6.83 (SD=0.161), Q2=6.73 (SD=0.181), Q3=7.01 (SD=0.149), and Q4=7.32 (SD=0.155), after controlling for age, BMI, and MAP (Fig. 2). Pairwise comparisons showed that the Q4 group is significantly different only from the Q1 (mean difference .491 m/s, p=0.031) and the Q2 (mean difference .587 m/s, p=.016) (Fig. 2).
The results of a multiple regression analysis of the factors influencing PWV revealed that resting heart rate is a significant predictor of cfPWV independent of age, BMI, MAP (Table 5). For each 1 bpm increase in resting heart rate, cfPWV increases about 0.02 m/s. The effects of BMI on PWV did not remain significant in this model with resting heart rate, age, and MAP as covariates.
This cross-sectional study found that resting heart rate is associated with cfPWV among 102 normotensive Korean Americans aged 21-60 years old. The significant association remained after controlling for classic cardiovascular risk factors including age, MAP, and BMI.
This is consistent with previous studies, which have measured arterial stiffness using methods other than cfPWV. One population-based study found an independent association of resting heart rate with arterial stiffness which was measured by the relative change in carotid diameter and aortic diameter for a cardiac cycle divided by the brachial pulse pressure.9) However, the shape of pulse waves change as it travels distally, and the amplitude of pulse waves measured at a peripheral arterial site increases with heart rate; thus, further studies were suggested to calculate arterial distensability using central aortic pulse pressure.13) A few other studies similarly reported a significant association of heart rate and arterial stiffness as measured by brachial-ankle pulse wave velocity (ba-PWV).14) Although ba-PWV is more convenient because it does not require access to the groin to measure femoral arterial pulse waves, the brachial artery and tibial artery contain more smooth muscle than the central arteries. Therefore, a number of chemical stimuli such as norepinephrine or vasopressin can increase heart rate and cause tension in the muscular arteries, resulting in a false relationship between resting heart rate and arterial stiffness. Thus, this relationship should be examined by measuring aortic stiffness.
Previous studies that used cfPWV reported mixed results on the association of heart rate and arterial stiffness according to whether subjects were hypertensive or not. For example, a study with hypertension patients reported that cfPWV was associated with a 24-hour ambulatory heart rate.15) However, another study demonstrated that such a relationship was observed only in hypertensive subjects not in normotensive subjects. Their 6-year follow-up data also showed that heart rate was the major determinants of accelerated progression of cfPWV only in hypertensive subjects.16) The relationship between heart rate and cfPWV in nonhypertensive people is unclear. There is one study that reported a significant relationship in nonhypertesive subjects but their subjects' BP levels actually belonged to either prehypertension or stage 1 hypertension.12) The current study demonstrates that the independent association of resting heart rate and cfPWV is also observed in normotensive adults.
A few previous studies illuminate the methodological issues in investigating the association between heart rate and PWV. Lantelme et al.10) suggested that heart rate might be a confounder in PWV assessment, demonstrating that heart rate changed by permanent cardiac pacing was a factor for the intra-individual variation of cfPWV with no significant blood pressure variation. However, three other studies conducted in paced patients reported that the change of heart rate made no substantial change in cfPWV.11)17)18) In the present study, descriptive analysis showed that cfPWV gradually increases as resting heart rate increases by quartiles (Table 3). However, the ANCOVA results showed that after for controlling age, MAP, and BMI, the second quartile group with resting heart rate between 56 and 60 bpm had the lowest level of cfPWV, demonstrating a U-shaped relationship between resting heart rate and cfPWV. It is interesting to note that in one epidemiological study that reported a strong association of heart rate with sudden death from coronary heart disease (CHD) and non-CVD death, a U-shaped relationship was observed between heart rate and mortality. Their findings suggested that the associations of heart rate with other CVD death and non-sudden CHD might be secondary to associations between heart rate and other CVD risk factors. Further studies that include both arterial stiffness and heart rate may help to determine whether arterial stiffness is the factor to explain the link between heart rate and mortality.
As for the different measures of resting heart rate, 24-hour heart rate had a better reproducibility over office heart rate, showing office heart rate was not reproducible in heart rate levels higher than 85 bpm and in people with severe office hypertension.19) However, the Syst-Eur study showed that a significant association with non-cardiovascular mortality was found only with a clinic heart rate not with a 24-hour ambulatory heart rate.7) Heart rate is a simple and easy noninvasive measure; however, numerous confounding factors including physical fitness, activity level, fitness level, air temperature, body position, emotions, body size, and medications that decrease heart rate (e.g., b-blocker) can be a hindrance to use heart rate as clinical index to assess cardiovascular health. In particular, heart rate measured in the clinic may be heightened due to the white-coat effect and this may reflect autonomic reactivity to the environment rather than resting autonomic tone. Self-measurement of resting heart rate at home using a home BP measurement device can be used to eliminate the white-coat effect. One population based study demonstrated that the morning home-measured resting heart rate is significantly associated with the 10-year risk of cardiovascular mortality after controlling for home BP.20) Careful consideration on valid measures of resting heart rate would be an important component in studies that investigate heart rate and cardiovascular risk. In the present study, resting heart rate was measured after at least a 10-minute rest in a quiet and non-threatening home environment; therefore, it is unlikely that there was a white-coat effect in measuring resting heart rate.
There could be several potential mechanisms that explain the positive association observed between resting heart rate and arterial stiffness. First, sympathetic activity manifested as an increased heart rate may facilitate the renin angiotensin aldosterone system.21) Angiotensin II, converted from angiotensin I by angiotensin converting enzyme (ACE), is known as an important stimulus of NADPH oxidase that generate reactive oxygen species (ROS). Studies have shown that angiotensin II – induced ROS decrease the bioavailability of endothelial nitric oxide synthase (eNOS),22) which results in a decreased production of a potent vasodilator, nitric oxide (NO). Further, ACE, which is released not only from the lung but also from vascular endothelium, is also known to degrade bradykinin.23) Bradykinin releases a number of vasodilators such as NO through bradykinin B1 and B2 receptors expressed in the vasculature. Arterial stiffness is inversely related to bioavailability of NO;23) thus, ACE may contribute to arterial stiffness by increasing angiotensin II and degrading bradykinin.
Overdrive of sympathetic functioning can also contribute to arterial stiffness through its effect on catecholamines (CA) and matrix metalloproteinase (MMP). In response to the activity of sympathetic function, CA such as norepinephrine and epinephrine is released from the locus coeruleus (LC) in the brain stem, sympathetic nerve terminals in various organs, and the adrenal medulla.24) MMPs are a family of proteinases that degrade extracellular matrix proteins. In particular, MMP2 and MMP9, which degrade both type IV collagen and gelatin, have been associated with arterial stiffness in healthy subjects25) and in patients with essential hypertension.26) In-vitro studies showed that norepinephrine upregulated the expression of MMP9 and MMP2 in a variety of cells including the aorta.27) Further, plasma norepinephrine levels had a positive correlation with MMP2 in healthy subjects.28) Therefore, CA may contribute to arterial stiffness through its effects on MMP levels. Whether one of these mechanisms (ACE versus MMP) may work relatively stronger in hypertensive versus nonhypertensive populations is unknown.
Some limitations to the current study should be acknowledged. First, pulse wave velocity is largely subject to blood pressure.29) Despite our efforts to minimize the influence of blood pressure on arterial stiffness by selecting nonhypertensive individuals and using statistical adjustment, the intrinsic nature of the bi-directional relationship between blood pressure and pulse wave should be taken into account. Second, there are several factors that may influence arterial stiffness and they include hypercholesterolemia and diabetes. This study tried to exclude people who have any condition that may possibly increase arterial stiffness. However, the screening was conducted based on self-reporting, not an actual blood test. Third, physical activity is an important determinant of resting heart rate and athletic people are known to have a relatively low resting heart rate.30) In this study that did not include the factor of physical activity; effects of physical activity on resting heart rate are unknown. For future studies, stratification of physical activity levels is suggested when studying about a resting heart rate.
The present study clearly shows that the significant association between heart rate and aortic stiffness measured by cfPWV is observed even in normotensive adults. Autonomic dysfunction characterized by a greater sympathetic drive has been established in the early stages of essential hypertension.4) Given the association between heart rate and aortic stiffness in normotensive subjects and the epidemiological study reporting that arterial stiffness may itself predict progression of hypertension in normotensive subjects,2) a persistent increase of sympathetic function may promote the development of hypertension through its impact on arterial stiffness. Further studies are recommended to replicate this result in a large sample and to establish the prognostic value of heart rate in assessing arterial stiffness.
Figures and Tables
Fig. 1
The scatterplot graphic between resting heart rate and cfPWV. PWV=5.293+0.028 RHR, p=0.019, R-Square= 0.054. cfPWV: carotid-femoral pulse wave velocity, RHR: resting heart rate.
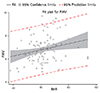
Fig. 2
Mean cfPWV in resting heart rate quartile groups. Covariates appearing in the model are evaluated at the following values: Age=39.64 years, BMI=23.59, and MAP=87.85. cfPWV difference between Q1&Q4=0.491 m/s (p=0.031) and between Q2&Q4=0.589 m/s (p=0.016). cfPWV: carotid-femoral pulse wave velocity, BMI: body mass index, MAP: mean arterial pressure.
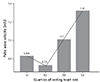
Table 2
Correlations
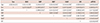
*Correlation is significant at the 0.05 level (2-tailed), †Correlation is significant at the 0.01 level (2-tailed). BMI: body mass index, SBP: systolic blood pressure, DBP: diastolic blood pressure, MAP: mean arterial pressure, HR: heart rate, cfPWV: carotid-femoral pulse wave velocity, r(p): pearson product-moment correlation coefficient (probability)
References
1. Chung CM, Lin YS, Chu CM, et al. Arterial stiffness is the independent factor of left ventricular hypertrophy determined by electrocardiogram. Am J Med Sci. 2012; 344:190–193.
2. Dernellis J, Panaretou M. Aortic stiffness is an independent predictor of progression to hypertension in nonhypertensive subjects. Hypertension. 2005; 45:426–431.
3. Laurent S, Cockcroft J, Van Bortel L, et al. Expert consensus document on arterial stiffness: methodological issues and clinical applications. Eur Heart J. 2006; 27:2588–2605.
4. Palatini P, Dorigatti F, Zaetta V, et al. Heart rate as a predictor of development of sustained hypertension in subjects screened for stage 1 hypertension: the HARVEST Study. J Hypertens. 2006; 24:1873–1880.
5. Bønaa KH, Arnesen E. Association between heart rate and atherogenic blood lipid fractions in a population. The tromosø study. Circulation. 1992; 86:394–405.
6. Hozawa A, Ohkubo T, Kikuya M, et al. Prognostic value of home heart rate for cardiovascular mortality in the general population: the Ohasama study. Am J Hypertens. 2004; 17(11 Pt 1):1005–1010.
7. Palatini P, Thijs L, Staessen JA, et al. Predictive value of clinic and ambulatory heart rate for mortality in elderly subjects with systolic hypertension. Arch Intern Med. 2002; 162:2313–2321.
8. Palatini P, Parati G. Persistently elevated heart rate accelerates the progression of arterial stiffness. J Hypertens. 2010; 28:653–656.
9. Whelton SP, Blankstein R, Al-Mallah MH, et al. Association of resting heart rate with carotid and aortic arterial stiffness: multi-ethnic study of atherosclerosis. Hypertension. 2013; 62:477–484.
10. Lantelme P, Mestre C, Lievre M, Gressard A, Milon H. Heart rate: an important confounder of pulse wave velocity assessment. Hypertension. 2002; 39:1083–1087.
11. Wilkinson IB, Mohammad NH, Tyrrell S, et al. Heart rate dependency of pulse pressure amplification and arterial stiffness. Am J Hypertens. 2002; 15(1 Pt 1):24–30.
12. Sa Cunha R, Pannier B, Benetos A, et al. Association between high heart rate and high arterial rigidity in normotensive and hypertensive subjects. J Hypertens. 1997; 15(12 Pt 1):1423–1430.
13. Avolio A, Butlin M, Tan I. Importance of pressure pulse amplification in the association of resting heart rate and arterial stiffness. Hypertension. 2013; 62:e46.
14. Tomiyama H, Hashimoto H, Tanaka H, et al. Synergistic relationship between changes in the pulse wave velocity and changes in the heart rate in middle-aged Japanese adults: a prospective study. J Hypertens. 2010; 28:687–694.
15. Albaladejo P, Asmar R, Safar M, Benetos A. Association between 24-hour ambulatory heart rate and arterial stiffness. J Hum Hypertens. 2000; 14:137–141.
16. Benetos A, Adamopoulos C, Bureau JM, et al. Determinants of accelerated progression of arterial stiffness in normotensive subjects and in treated hypertensive subjects over a 6-year period. Circulation. 2002; 105:1202–1207.
17. Albaladejo P, Laurent P, Pannier B, Achimastos A, Safar M, Benetos A. Influence of sex on the relation between heart rate and aortic stiffness. J Hypertens. 2003; 21:555–562.
18. Albaladejo P, Copie X, Boutouyrie P, et al. Heart rate, arterial stiffness, and wave reflections in paced patients. Hypertension. 2001; 38:949–952.
19. Palatini P, Winnicki M, Santonastaso M, et al. Reproducibility of heart rate measured in the clinic and with 24-hour intermittent recorders. Am J Hypertens. 2000; 13(1 Pt 1):92–98.
20. Hozawa A, Ohkubo T, Kikuya M, et al. Prognostic value of home heart rate for cardiovascular mortality in the general population: the Ohasama study. Am J Hypertens. 2004; 17(11 Pt 1):1005–1010.
21. Grippo AJ, Johnson AK. Stress, depression and cardiovascular dysregulation: a review of neurobiological mechanisms and the integration of research from preclinical disease models. Stress. 2009; 12:1–21.
22. Amiya E, Watanabe M, Takeda N, et al. Angiotensin II impairs endothelial nitric-oxide synthase bioavailability under free cholesterol-enriched conditions via intracellular free cholesterol-rich membrane microdomains. J Biol Chem. 2013; 288:14497–14509.
23. Hornig B, Kohler C, Drexler H. Role of bradykinin in mediating vascular effects of angiotensin-converting enzyme inhibitors in humans. Circulation. 1997; 95:1115–1118.
24. Kawahara H, Kawahara Y, Westerink BH. The role of afferents to the locus coeruleus in the handling stress-induced increase in the release of noradrenaline in the medial prefrontal cortex: a dual-probe microdialysis study in the rat brain. Eur J Pharmacol. 2000; 387:279–286.
25. Yasmin , McEniery CM, Wallace S, et al. Matrix metalloproteinase-9 (MMP-9), MMP-2, and serum elastase activity are associated with systolic hypertension and arterial stiffness. Arterioscler Thromb Vasc Biol. 2005; 25:372.
26. Tan J, Hua Q, Xing X, Wen J, Liu R, Yang Z. Impact of the metalloproteinase-9/tissue inhibitor of metalloproteinase-1 system on large arterial stiffness in patients with essential hypertension. Hypertens Res. 2007; 30:959–963.
27. Hu Z, Wang Z, Wu H, et al. Ang II enhances noradrenaline release from sympathetic nerve endings thus contributing to the up-regulation of metalloprotease-2 in aortic dissection patients' aorta wall. PLoS One. 2013; 8:e76922.
28. Yang EV, Bane CM, MacCallum RC, Kiecolt-Glaser JK, Malarkey WB, Glaser R. Stress-related modulation of matrix metalloproteinase expression. J Neuroimmunol. 2002; 133:144–150.
29. Chen W, Li S, Fernandez C, et al. Temporal relationship between elevated blood pressure and arterial stiffening among middle-aged black and white adults: The bogalusa heart study. Am J Epidemiol. 2016; 183:599–608.
30. Kwon O, Park S, Kim YJ, et al. The exercise heart rate profile in master athletes compared to healthy controls. Clin Physiol Funct Imaging. 2016; 36:286–292.