Abstract
Background and Objectives
Coronary collateral circulation (CCC) has been attributed as inborn bypass mechanisms supporting ischemic myocardium. Various factors have been postulated in CCC. Whole blood viscosity (WBV) has been an underappreciated entity despite close relationships between multiple cardiovascular diseases. WBV can be calculated with a validated equation from hematocrit and total plasma protein levels for a low and high shear rate. On the grounds, we aimed to evaluate the association between WBV and CCC in patients with chronic total occlusion.
Subjects and Methods
A total of 371 patients diagnosed as having at least one major, chronic total occluded coronary artery were included. 197 patients with good CCC (Rentrop 2 and 3) composed the patient group. The poor collateral group consisted of 174 patients (Rentrop grade 0 and 1).
Results
Patients with poor CCC had higher WBV values for a low-shear rate (LSR) (69.5±8.7 vs. 60.1±9.8, p<0.001) and high-shear rate (HSR) (17.0±2.0 vs. 16.4±1.8, p<0.001) than the good collateral group. Correlation analysis demonstrated a significant negative correlation between the grade of CCC and WBV for LSR (β=0.597, p<0.001) and HSR (β=0.494, p<0.001). WBV for LSR (β=0.476, p<0.001) and HSR (β=0.407, p<0.001) had a significant correlation with the synergy between percutaneous coronary intervention with taxus and cardiac surgery (SYNTAX) score. A multivariate analysis showed that the WBV for both shear rates were independent risk factors of poor CCC (WBV at LSR, OR: 1.362 CI 95%: 1.095-1.741 p<0.001 and WBV at HSR, 1.251 CI 95%: 1.180-1.347 p<0.001).
Coronary collateral circulation (CCC) has been attributed as an inborn bypass process supporting myocardium jeopardized by ischemia. CCC has been associated with reduced infarct size, morbidity, and mortality in the setting of myocardial infarction.1) Various factors have been postulated in the collateralization mechanism including ischemia, the pressure gradient between donor and recipient arteries, endothelial mitogens such as endothelial growth factor and endothelial shear stress (ESS).2)3)
As a determinant of shear stress, blood viscosity has been an underappreciated entity in routine cardiovascular evaluation despite close relationships between multiple cardiovascular diseases.4) Additionally, hyperviscosity has substantial pathophysiologic similarities with traditional risk factors such as hypertension, hypercholesterolemia, diabetes mellitus, male gender, cigarette smoking.5) Routine laboratory measurement of whole blood viscosity (WBV) may be compelling because of non-standardized methods, the necessity of sophisticated devices and scarce research data.6) WBV can be calculated with a validated equation from hematocrit (HCT) and total plasma protein levels (TP) for low and high shear rate.7) Obtaining an estimated value about WBV with this simple formula, can provide clinicians a new tool for patient bedside evaluations.
On the grounds, we aimed to evaluate the association between WBV and CCC in patients with chronic total occlusion (CTO).
A total of 371 patients to whom elective coronary angiography with clinical indications was performed, including symptoms of angina and abnormal noninvasive test results (either treadmill exercise tests or myocardial perfusion scintigraphy) between March 2011- April 2015 and diagnosed as having at least one major, chronic total occluded coronary artery were included in this study. CTO was defined as ≥99% epicardial coronary artery stenosis, presumed duration older than three months (based on the onset of ischemic symptoms, the history of myocardial infarction and previous coronary angiography) leading a decreased blood flow that is clinically significant (TIMI 0).8) Patients were re-evaluated according to the presence of coronary collateralization and graded based on Rentrop classification. 197 patients with good CCC (Rentrop 2 and 3) composed the patient group (57% male, mean age 65.1±8.5). The poor collateral group (61% male, mean age 66.2±9.1) consisted of 174 patients (Rentrop grade 0 and 1).
Patients with acute coronary syndromes (within 6 months), anemia, blood dyscrasias, having a blood transfusion at least 3 month time, active infection, decompensated respiratory failure (having an oxygen saturation less than 92% with or without chronic obstructive pulmonary disease), oncological or inflammatory disease, renal or hepatic insufficiency, severe valvular disease, acute decompensated heart failure, left ventricular ejection fraction <40%, hypo and hyperthyroidism, and having previous coronary artery bypass grafting surgery which can affect collateral grading were excluded. Left ventricular ejection fraction was measured via the modified Simpson technique by transthoracic echocardiography (Vivid 7 Pro, GE VingMed Ultrasound, Horten, Norway).
The study protocol was reviewed and approved by the institutional ethics committee in accordance with the Declaration of Helsinki. All patients gave informed consent.
Peripheral blood samples were collected via the antecubital vein after a 12-hour overnight fast in appropriate tubes. Dry tubes for biochemical tests and tubes with ethylenediaminetetraacetic acid (EDTA) for the hematological test were used. Erythrocyte count, hemoglobin, hematocrit, and white blood cell (WBC) count were measured using an automated hematology analyzer XE-1200 (Sysmex, Kobe, Japan). The biochemical measurements were determined by using a molecular analyzer (Roche Diagnostics, Manheim, Germany).
WBV was calculated for both low shear rate (LSR) (0.5 sec -1) and high-shear rate (HSR) (208 sec -1) from hematocrit and total plasma protein concentration with using validated formula.7)
All patients underwent elective coronary angiography with an experienced cardiologist using the standard Judkins technique by femoral or radial approach. The evaluation of coronary angiograms, determination of CCC's Rentrop grade and calculation of SYNTAX score were made by two interventional cardiologist groups (two interventional cardiologists per each group) who were blinded to the laboratory, clinical data and the coronary assessment of the other group. In the case of a discrepancy between the interpretation, a third interventional cardiologist who is blinded analyzes the groups and examines the coronary angiograms. A Rentrop score was graded in the scale of 0-3. Any visible filling on collaterals was considered as grade '0', filling of side branches without epicardial segments as grade '1', partial filling of epicardial segments as grade '2' and complete filling of epicardial segments was considered as grade '3'.9) In patients who had more than one collateral vessel with different grades, the researchers calculated the mean collateral score and accepted this value as the CC score. In the interobserver correlation analysis, intraclass correlation coefficient was delineated as 0.957 (95% CI: 0.912-0.972) for CC grade and 0.971, (95% CI: 0.929-0.989) for SYNTAX score.
The diseased coronary artery was defined as having >50% stenoses in the left anterior descending, circumflex, right coronary artery and/or main branches.
The continuous variables were reported as the mean±standard deviation (SD), and the categorical variables were expressed as the number of patients and percentages. The comparisons of between the two groups were performed with a Student's t-test for continuous variables and with the chi-squared test or Fisher's exact test for the categorical variables. A one way ANOVA was used for the comparison of WBV values between groups, and a post-hoc analysis were made with Bonferroni test. The correlations of WBV with Rentrop grades and Syntax score were examined with Spearman's correlation coefficient. We analyzed the effects of different variables on the presence of CCC in an univariate analysis and determined the variables whose unadjusted p-value was <0.05 as potential risk markers and included them in the full models. For WBV at HSR and LSR variables, two different models were composed. WBV at LSR was included in Model 1 and WBV at HSR was incorporated in Model 2. We composed the models by using backward elimination of a multivariate regression analysis, and we eliminated potential risk markers by using likelihood ratio tests. We evaluated the predictiveness of WBV for CCC with receiver operating characteristic (ROC) analysis. A p value<0.05 was considered statistically significant. Intraclass correlation coefficient and Bland & Altman methods were used for inter-observer correlations analysis. Data were analyzed by using Pls provide information below; manufacturing company name, its location and country, pls. SPSS 20.0 software (SPSS Inc., Chicago, IL, USA).
The comparisons of baseline characteristic and laboratory findings of groups were demonstrated in Table 1. In patients with poor CCC, the prevalence of diabetes mellitus (DM), WBC and low-density lipoprotein (LDL) levels were higher than patients in the good CCC group. Patients with poor CCC had higher WBV values for LSR (69.5±8.7 vs. 60.1±9.8, p<0.001) and HSR (17.0±2.0 vs. 16.4±1.8, p<0.001) than the good collateral group.
Correlation analysis demonstrated a significant negative correlation between the grade of CCC and WBV for LSR (β=0.597, p<0.001) and HSR (β=0.494, p<0.001). Also WBV for LSR (β=0.476, p<0.001) and HSR (β=0.407, p<0.001) had a significant correlation with SYNTAX score (Fig. 1).
At multivariate analysis, we composed two different models to evaluate the predictiveness of WBV parameters for each shear rate (Table 2). In model 1, adjusted with the SYNTAX score, Diabetes Mellitus, LDL, WBC levels, WBV at LSR (OR: 1.362 CI 95%: 1.095-1.741 p<0.001) was determined as an independent risk factor of poor CCC. In model 2, SYNTAX score, Diabetes Mellitus, LDL, WBC levels, and WBV at HSR, (OR: 1.251 CI 95%: 1.180-1.347 p<0.001) were found as independent predictors of poor CCC.
In ROC analysis, a cut-off value of 67.1 WBV for LSR had a 73% sensitivity and a 67.7% specificity for prediction of poor CCC (area under curve [AUC]: 0.775, p<0.001) and a cut-off value of 16.8 WBV for HSR had a 70% sensitivity and 70.5% specificity for prediction of poor CCC (AUC: 0.753, p<0.001) (Fig. 2).
The present study showed that higher WBV is an independent risk factor for poor CCC. Furthermore, there is an inverse relation between WBV and CCC formation, and SYNTAX score showed a positive correlation with WBV that hemorheological variables were both related to the extent and severity of coronary artery disease and the consequent adaptive mechanisms.
Hematocrit, plasma viscosity and red blood cell (RBC) deformability and aggregation are the main factors that determine WBV. WBV and plasma viscosity are positively related to low-density lipoprotein, cholesterol, triglycerides, uric acid, von Willebrand factor and C-reactive protein whereas these parameters negatively to high-density lipoprotein.10)11)12) Alteration in any of the variables mentioned above results in influenced plasma viscosity.13) Many therapies including lipid-lowering drugs, apheresis, and streptokinase, have beneficial effects due to the decreased plasma viscosity, RBC aggregation and improved RBC deformability.14)15)16) The RBC deformability is important because it provides oxygen delivery and determines the cell survival time in the circulation.17)
CCC alternatively provides blood supply to the ischemic myocardium and well-developed collaterals are related to better long-term prognosis in patients with coronary artery disease.18) Angiogenesis (new vessel formation) and/or arteriogenesis (growth of preexisting arterioles) result in the CCC development. Although, in the previous reports, the role of hemorheological and biochemical factors in the development CCC has been recognized,19)20) the effect of the WBV on CCC has not been studied well. The present study showed that patients with poor CCC had significantly higher WBV values for both shear rates compared to the good collateral group, and higher WBV was an independent predictor for poor CCC. In large vessels blood flow is mainly determined by hematocrit, and it decreases inversely with the vessel diameter causing blood viscosity to remain steady.13) Because, increased blood viscosity results in slowing down the blood flow stasis,21) it has been hypothesized that to sustain normal blood stream within the arterial system, blood viscosity must decrease, and erythrocyte deformability must increase.22) Therefore, in addition to RBC deformability, WBV takes an active role in the perfusion of the capillaries. Experimental studies conducted on dogs showed that WBV significantly increased when an ischemic event occurred in the left descending artery and elevated WBV impaired the collateral perfusion of the ischemic myocardium and interestingly hemodilution improved the collateral perfusion.23)24) In a rat model study, 1,5-benzothiazepine derivative increased the blood flow to femoral artery via collateral circulation and decreased WBV.25) Also, this agent improved the RBC deformability. The possible reason for the negative effect of elevated WBV in collateral formation is possibly related to restricted RBC functions and deformability in the viscose blood. Decreased deformability of the RBCs causes the production of the free oxygen radicals that may negatively affect angiogenesis/arteriogenesis in the ischemic regions. Accordingly, our study supports that improvement in blood fluidity by a decreasing plasma viscosity and RBC aggregation may improve collateral formation and functions.
The SYNTAX score predicts not only possible peri-procedural difficulties but also indicates the pattern of atheroma including length, calcification and thrombosis of the lesion.26) In the present study, WBV showed a positive correlation with The SYNTAX score. Previously, an association between multi-vessel disease and CCC has been reported.27) Also, alterations in rheological properties showed a positive correlation with the severity of atherosclerosis in the coronary artery tree.28) Atherosclerotic plaque localizations have a linear relation with the blood viscosity that frequent plaque formation were observed among the higher viscosity regions especially in the proximal segments of the coronary arteries.22) Börekçi et al. observed higher SYNTAX scores in patients with poor CCC.20) Similarly, we observed that in the poor CCC group, SYNTAX scores were significantly higher compared to good CCC group. Therefore, higher WBV may also an important interfering factor for the development of CCC via causing a high intrinsic resistance of blood flow in microvessels.
In line with the association of increased WBV with impaired CCC, monitoring of WBV and establishing the diagnosis of chronic hyperviscosity may provide additional information in conjunction with traditional risk factors. Patients with higher WBV should be specified as a high-risk group and followed in a more closely scheduled program. WBV-lowering additional strategies including more aggressive blood pressure, lipid profile control as well as HDL-raising medications may have additional benefits.
WBV-lowering therapies can interrupt or slow the progression of atherosclerosis. Thereby, theurapeutic intervenvions including therapeutic phlebotomy, hemodilution and plasmapheresis may improve CCC in patients with elevated WBV.
The limitations of the present study implicate the possible underestimation of the coronary collateral formation with Rentrop classification, and other markers including vascular endothelial growth factor, nitric oxide, and TNF-α that possibly affect CCC but were not studied. Finally, inherent to the retrospective design of the study the prognostic relation between WBV and future adverse cardiac events were not addressed.
In conclusion, WBV has been demonstrated as the overlooked predictor of poor coronary collateralization. WBV seemed to be associated with microvascular perfusion and angiogenesis/arteriogenesis process and elevated WBV impaired CCC development. Extrapolation of whole blood viscosity with simple and costless formula using hematocrit and total protein level provide a new tool in the evaluation of patients with ischemic heart disease.
Figures and Tables
Fig. 1
The correlation between the SYNTAX score with whole blood viscosity at a low shear rate (A) and high shear rate (B). Each dot represents one patient; the straight line represents the best fit line obtained by linear regression analysis. WBV: whole blood viscosity, SYNTAX: synergy between percutaneous coronary intervention with taxus and cardiac surgery.
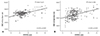
Fig. 2
The ROC curve analysis showing the predictive cut-off value of WBV at high shear rate (red line) and low shear rate (blue line) for coronary collateralization. AUC: area under curve, HSR: high shear rate, LSR: low shear rate, ROC: receiver operating curve, WBV: whole blood viscosity.
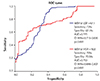
Table 1
Baseline characteristics and laboratory parameters of groups classified according to rentrop grade
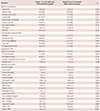
Data are expressed as mean±standard deviation for normally distributed parametric variables and percentage for categorical variables. ARB: angiotensin receptor blockers, ASA: acetylsalicylic acid, BMI: body mass index, CAD: coronary artery disease, CX: circumflex artery, HDL-C: high density lipoprotein cholesterol, HSR: high shear rate, LAD: left anterior descending artery, LDL-C: low density lipoprotein cholesterol, LSR: low shear rate, LVEF: left ventricular ejection fraction, RCA: right coronary artery, WBC: white blood count, WBV: whole blood viscosity
Table 2
Predictors of poor coronary collateral circulation in multivariate logistic regression analyses
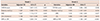
References
1. Cohen M, Rentrop KP. Limitation of myocardial ischemia by collateral circulation during sudden controlled coronary artery occlusion in human subjects: a prospective study. Circulation. 1986; 74:469–476.
2. Kilian JG, Keech A, Adams MR, Celermajer DS. Coronary collateralization: determinants of adequate distal vessel filling after arterial occlusion. Coron Artery Dis. 2002; 13:155–159.
3. van Royen N, Piek JJ, Buschmann I, Hoefer I, Voskuil M, Schaper W. Stimulation of arteriogenesis; a new concept for the treatment of arterial occlusive disease. Cardiovasc Res. 2001; 49:543–553.
4. Papaioannou TG, Stefanadis C. Vascular wall shear stress: basic principles and methods. Hellenic J Cardiol. 2005; 46:9–15.
5. Sloop G, Holsworth RE Jr, Weidman JJ, St Cyr JA. The role of chronic hyperviscosity in vascular disease. Ther Adv Cardiovasc Dis. 2015; 9:19–25.
6. Cho YI, Cho DJ, Rosenson RS. Endothelial shear stress and blood viscosity in peripheral arterial disease. Curr Atheroscler Rep. 2014; 16:404.
7. de Simone G, Devereux RB, Chien S, Alderman MH, Atlas SA, Laragh JH. Relation of blood viscosity to demographic and physiologic variables and to cardiovascular risk factors in apparently normal adults. Circulation. 1990; 81:107–117.
8. Shah PB. Management of coronary chronic total occlusion. Circulation. 2011; 123:1780–1784.
9. Rentrop KP, Cohen M, Blanke H, Phillips RA. Changes in collateral channel filling immediately after controlled coronary artery occlusion by an angioplasty balloon in human subjects. J Am Coll Cardiol. 1985; 5:587–592.
10. Sloop GD, Garber DW. The effects of low-density lipoprotein and high-density lipoprotein on blood viscosity correlate with their association with risk of atherosclerosis in humans. Clin Sci (Lond). 1997; 92:473–479.
11. Blann A, Bignell A, McCollum C. von Willebrand factor, fibrinogen and other plasma proteins as determinants of plasma viscosity. Atherosclerosis. 1998; 139:317–322.
12. Muggeo M, Calabrò A, Businaro V, Moghetti P, Padovan D, Crepaldi G. Correlation of metabolic and hemorrheological parameters in diabetes and hyperlipidemia. Ric Clin Lab. 1983; 13:Suppl 3. 165–179.
13. Wasilewski J, Turczyński B, Słowińska L, Kowalik V, Osadnik T, Poloński L. Haemorheological factors and myocardial reperfusion in patients with ST-elevation myocardial infarction undergoing primary coronary intervention. Kardiol Pol. 2007; 65:778–785. discussion 786-7.
14. Empen K, Geiss HC, Lehrke M, Otto C, Schwandt P, Parhofer KG. Effect of atorvastatin on lipid parameters, LDL subtype distribution, hemorrheological parameters and adhesion molecule concentrations in patients with hypertriglyceridemia. Nutr Metab Cardiovasc Dis. 2003; 13:87–92.
15. Mellwig KP, Baller D, Schmidt HK, et al. Myocardial perfusion under H.E.L.P.-apheresis. Objectification by PET. Z Kardiol. 2003; 92:Suppl 3. III30–III37.
16. Arntz HR, Perchalla G, Roll D, Heitz J, Schäfer JH, Schröder R. Blood rheology in acute myocardial infarction: effects of high-dose i.v. streptokinase compared to placebo. Eur Heart J. 1992; 13:275–280.
17. Mohandas N, Chasis JA, Shohet SB. The influence of membrane skeleton on red cell deformability, membrane material properties, and shape. Semin Hematol. 1983; 20:225–242.
18. Hansen JF. Coronary collateral circulation: clinical significance and influence on survival in patients with coronary artery occlusion. Am Heart J. 1989; 117:290–295.
19. Nacar AB, Erayman A, Kurt M, et al. The relationship between coronary collateral circulation and neutrophil/lymphocyte ratio in patients with coronary chronic total occlusion. Med Princ Pract. 2015; 24:65–69.
20. Börekçi A, Gür M, Şeker T, et al. Coronary collateral circulation in patients with chronic coronary total occlusion; its relationship with cardiac risk markers and SYNTAX score. Perfusion. 2015; 30:457–464.
21. Yaylali YT, Susam I, Demir E, et al. Increased red blood cell deformability and decreased aggregation as potential adaptive mechanisms in the slow coronary flow phenomenon. Coron Artery Dis. 2013; 24:11–15.
22. Soulis JV, Farmakis TM, Giannoglou GD, et al. Molecular viscosity in the normal left coronary arterial tree. Is it related to atherosclerosis? Angiology. 2006; 57:33–40.
23. Biro GP, Beresford-Kroeger D, Hendry P. Early deleterious hemorheologic changes following acute experimental coronary occlusion and salutary antihyperviscosity effect of hemodilution with stroma-free hemoglobin. Am Heart J. 1982; 103:870–878.
24. Biro GP, Beresford-Kroeger D. The effect of hemodilution with stroma-free hemoglobin and dextran on collateral perfusion of ischemic myocardium in the dog. Am Heart J. 1980; 99:64–75.
25. Doi H, Kaburaki M, Inoue H, Suzumura K, Narita H. Protective effect of TA-993, a novel therapeutic agent for peripheral circulatory insufficiency, on skeletal muscle fatigue in a rat model of hindlimb ischemia. Jpn J Pharmacol. 2000; 83:73–81.
26. van Gaal WJ, Ponnuthurai FA, Selvanayagam J, et al. The Syntax score predicts peri-procedural myocardial necrosis during percutaneous coronary intervention. Int J Cardiol. 2009; 135:60–65.
27. Kyriakides ZS, Kremastinos DT, Michelakakis NA, Matsakas EP, Demovelis T, Toutouzas PK. Coronary collateral circulation in coronary artery disease and systemic hypertension. Am J Cardiol. 1991; 67:687–690.
28. Junker R, Heinrich J, Ulbrich H, et al. Relationship between plasma viscosity and the severity of coronary heart disease. Arterioscler Thromb Vasc Biol. 1998; 18:870–875.