Abstract
Residual cardiovascular risk and failure of high density lipoprotein cholesterol raising treatment have refocused interest on targeting hypertriglyceridemia. Hypertriglyceridemia, triglyceride-rich lipoproteins, and remnant cholesterol have demonstrated to be important risk factors for cardiovascular disease; this has been demonstrated in experimental, genetic, and epidemiological studies. Fibrates can reduce cardiovascular event rates with or without statins. High dose omega-3 fatty acids continue to be evaluated and new specialized targeting treatment modulating triglyceride pathways, such as inhibition of apolipoprotein C-III and angiopoietin-like proteins, are being tested with regard to their effects on lipid profiles and cardiovascular outcomes. In this review, we will discuss the role of hypertriglyceridemia, triglyceride-rich lipoproteins and remnant cholesterol on cardiovascular disease, and the potential implications for treatment stargeting hypertriglyceridemia.
Current prevention guidelines focus on low-density lipoprotein (LDL) cholesterol lowering as the primary target of therapy, primarily by statins.1) However, many statin-treated individuals continue to experience cardiovascular events.2)3)4)5) Even after low LDL cholesterol levels (70-100 mg/dL) are achieved, residual cardiovascular risk is observed in the randomized clinical trials of high dose statins.2)5) For further reduction of residual cardiovascular risk, physicians should pay attention to modifiable risk factors such as hypertriglyceridemia, non-high-density lipoprotein (HDL) cholesterol, apolipoprotein B, low HDL cholesterol, visceral fat, and insulin resistance. As one of the correctable residual cardiovascular risk factors, hypertriglyceridemia is recently reemerging as one of the reliable therapeutic targets. In Asians, such as Koreans, Chinese, and Japanese, the prevalence of hypertriglyceridemia is greater than hypercholesterolemia within the Korean population,6)7)8) Chinese National Survey,9) and Japanese men (http://www.mhlw.go.jp/bunya/kenkou/eiyou/h25-houkoku.html). In addition, a 2007 Korean National Survey also revealed 33.2% of the general population had hypertriglyceridemia (triglycerides≥150 mg/dL) and 50.2% of them showed low HDL cholesterol levels (men<40 mg/dL, women<50 mg/dL).10) It is important to mention that recent genetic studies and randomized trials demonstrated that low HDL cholesterol might not be a cause of cardiovascular disease and hypertriglyceridemia and remnant cholesterol may be a cause.11) In this review, we reviewed updated evidence of the role of hypertriglyceridemia and triglyceride-rich lipoproteins (TRLs), remnant cholesterol as cardiovascular risk factors and the therapeutic target for reducing residual cardiovascular risk.
TRLs are too large to enter into the arterial intima and therefore cannot lead to development of atherosclerosis at greatly elevated triglycerides (TG) concentrations (>4450 mg/dL). By contrast, at mild to moderately raised TG concentrations (178-890 mg/dL), TRLs are small enough to enter into the arterial wall and thus have the potential to accumulate and cause atherosclerosis.11)12)13)
TRLs include chylomicrons, very large density lipoprotein (VLDL) and intermediate density lipoprotein (IDL). Their major protein component is apolipoprotein B. In the fasting state, only VLDL and IDL are present in plasma, whereas chylomicrons, VLDL and their remnants circulate under non-fasting conditions. TRLs undergo intravascular hydrolysis by lipoprotein lipase in the muscle, adipose tissue, heart and other tissues, where they provide free fatty acids for energy or storage. Upon lipolysis, chylomicrons and VLDL are depleted of TG and enriched in cholesterol, resulting in the formation of chylomicron remnants and VLDL remnants. In addition, their cholesterol content is further enhanced by the action of cholesterol ester transfer protein (CETP). Since most cells can degrade TG, and there are not any cells that can degrade cholesterol, the cholesterol content of TRLs is more likely to be the cause of atherosclerosis and cardiovascular disease rather than raised TG per se. Indeed, cholesterol, rather than TG accumulates in intimal foam cells and in atherosclerotic plaques, and remnant lipoproteins, much like LDL can enter the arterial intima. In contrast, chylomicrons are too large to enter. Lipoprotein lipase activity at the surface of remnant particles, either at the surface of vascular endothelium or within the intima, leads to liberation of free fatty acids, monoacylglycerols, and other molecules, each of which could cause local injury and inflammation. Although other possible mechanisms have been suggested, perhaps the simplest chain of events is that high triglyceride concentrations are a marker for raised remnants rich in cholesterol, which, upon entrance into the intima, leads to low-grade inflammation, foam cell formation, atherosclerotic plaques, and ultimately cardiovascular disease and increased mortality. (Fig.).11)1213)14)15)
Remnant cholesterol is the cholesterol content of TRLs (chylomicron remnants, VLDL, and IDL) in the fasting or non-fasting states. Because a direct assay that measures all remnants at the same time has not yet been developed, remnant cholesterol can be calculated as non-fasting total cholesterol minus HDL cholesterol minus LDL cholesterol.11) A recent study has shown that remnant cholesterol levels in TRLs are causal in ischemic heart disease independent of reduced HDL cholesterol.16) Because the level of TG is significantly correlated with the amount of remnant cholesterol in TRLs (r=0.96), the amount of TG may represent the level of remnant cholesterol.16) Therefore, the level of TG is a biomarker for circulating TRLs and their metabolic remnants.17)
TRLs drive atherothrombosis via a number of mechanisms. Particle size is a key limiting factor influencing the retention of apolipoprotein B containing lipoproteins. Although large chylomicrons (70 nm diameter) do not penetrate the arterial wall, smaller remnants of chylomicrons and large VLDL, as well as LDL and lipoprotein (a) enter the arterial wall and therefore enhance cholesterol accumulation directly.18) These TRL remnant particles contribute directly to plaque formation and progression.19) In addition, TRLs promote endothelial dysfunction by increasing immune inflammatory responses and destabilize plaque, enhance thrombus formation, promote secretion of tissue factor, fibrinogen, coagulation factors VII, XII, impair fibrinolysis, and increase plasminogen activator inhibitor-1 secretion.19)20)21)22)23)24)
Previous studies have shown that elevated fasting and non-fasting hypertriglyceridemia or very low-density lipoprotein cholesterol were associated with increased risk of coronary heart disease, even after adjustment for HDL cholesterol concentrations.9)25)26) Recent studies demonstrated that raised TG was strongly associated with increasing risks of cardiovascular disease. Raised non-fasting TG was strongly associated with increasing risks of myocardial infarction, ischemic heart disease, ischemic stroke, and all-cause mortality.27)28)29)30) Raised fasting and non-fasting TG were associated with an increased risk of coronary heart disease in an Emerging Risk Factors Collaboration study.31) This association was attenuated after adjustment for HDL cholesterol and non-HDL cholesterol. In multivariate analyses, the relationship between TG and cardiovascular diseases (CVD) is either attenuated or lost, thereby suggesting that the cholesterol content of TRLs or remnant cholesterol is causative for CVD rather than raised TG itself. In addition, data from three major Danish studies provides support for remnant cholesterol in TLRs as a causal factor for CVD by Mendelian randomization study.9)16)30) In these studies, a 39 mg/dL increase in non-fasting remnant cholesterol was associated with a 2.8 fold causal risk for ischemic heart disease which was higher than for observational data alone (hazard ratio 1.4, 95% confidence interval 1.3 to 1.5) and independent of HDL cholesterol concentration.16) These genetic studies reinforce the role of remnant cholesterol in TRLs rather than TG as a cardiovascular risk factor (Table 1). For non-fasting remnant cholesterol alone, for the ratio of non-fasting remnant cholesterol to HDL cholesterol, and for LDL cholesterol alone, causal risk estimates for genetically elevated levels were in the same direction and higher than corresponding risk estimates for corresponding increases in conventional plasma levels of the same lipoproteins; however, this was not the case for HDL cholesterol alone.16) These results demonstrated the important role of non-fasting remnant cholesterol, the ratio of non-fasting remnant cholesterol to HDL cholesterol, LDL cholesterol on the risk of ischemic heart disease than HDL cholesterol in general population.
Remnant cholesterol was causative for ischemic heart disease independent of HDL cholesterol levels (Table 2).32) In these studies, remnant cholesterol was estimated indirectly as total cholesterol minus the cholesterol contents of LDL and HDL. These population based studies clearly demonstrated a role of non-fasting TG and remnant cholesterol levels on the predictions of CVD events in general population. In the statin era, among patients receiving statin therapy after acute coronary syndrome, on-treatment TG≤150 mg/dL was associated with a lower risk of recurrent coronary heart disease events independently of the level of LDL cholesterol.33) Among patients with acute coronary syndrome treated effectively with statins, fasting TG predicts long-term and short-term cardiovascular risk.33) These data support the concept that achieving both low LDL cholesterol and low TRLs may be important therapeutic goals in patients following acute coronary syndrome.
The genetic basis of mild to moderate hypertriglyceridemia with or without elevated LDL cholesterol in most individuals is polygenic. Postprandial TG metabolism abnormalities are strongly associated with single nucleotide polymorphisms at common genetic loci. Candidate genes associated with postprandial lipemia include apolipoprotein A1, A4, A5, C3, E, lipoprotein lipase, fatty acid binding protein 2, microsomal transfer protein (MTP), scavenger receptor B1, Angiopoietin-like proteins (ANGPTL)4 and peroxisome proliferator-activated receptor (PPAR) α.32)34)
Recent Mendelian randomization studies with genetic variants reported several candidate genes that affect the concentrations of remnant cholesterol. The relative risks of remnant cholesterol on ischemic heart disease and all-cause mortality according to causal genetic variants compare with corresponding observational results are shown in Table 3.
Lipoprotein lipase (LPL) is the key TG regulating enzyme, which hydrolyzes TG in the circulation and promotes the hepatic uptake of TRLs. A common gain of function LPL variant, S447X, confers an anti-atherogenic lipid profile characterized by low levels of TG and lower incidence of vascular disease or myocardial infarction.35)36) In contrast, several loss of function LPL variants associated with elevated TG levels which have been associated with increased coronary artery disease (CAD) risk.37)38) It was identified as common non-coding variants at the LPL gene locus associated with both TG and CAD risk in the same direction by genome wide association studies.3940)41) Beyond LPL itself, common variants that influence TG levels are significantly associated with CAD risk even after adjusting for their effects on other lipid traits.42) Among the common variants with strong associations with both TG and CAD were those at a gene locus containing the genes apolipoprotein C3 (APOC3) and apolipoprotein A5 (APOA5) which encode apoC-III and apoA-V, respectively, and are found on TRLs and known to be the regulators of LPL activity and TG levels.43)
ApoC-III is a key regulator of fasting and postprandial plasma TG levels and TG homeostasis. It is synthesized principally in the liver and expressed in the liver and intestine and circulates on and exchanges between TRLs and HDL.44)45) ApoC-III inhibits LPL-mediated hydrolysis of TRLs and adversely affects receptor-mediated hepatic uptake of remnants of TRLs.45) At higher concentrations, ApoC-III also inhibits the activity of hepatic lipase, an enzyme that plays an important role in the conversion of VLDL to IDL and LDL, as well as in the remodeling of HDL. Thus, elevated levels of ApoC-III in plasma have been associated with both impaired lipolysis and impaired clearance of TRLs from the circulation, which results in the accumulation of atherogenic VLDL and chylomicron remnants.45) Furthermore, some study suggested that ApoC-III has direct proinflammatory effects at the level of the vessel wall.46)47) Elevated ApoC-III levels are an independent risk factor for cardiovascular disease and genetic variants that result in a loss of function and attenuated levels of ApoC-III in plasma are associated with a reduced risk of coronary heart disease.45)48)
APOA5 is thought to play a crucial role in TG metabolism. APOA5 knockout mice demonstrate profound TG, whereas human APOA5 transgenic mice have significantly lower plasma TG than controls.51) Studies of APOA5 have revealed several coding variants have been implicated in severe hypertriglyceridemia or hyperchylomicronemia and some common coding variants have also been attributed to increased CAD risk.52)53)54) In addition, carriers of rare non-synonymous mutations at APOA5 were at a 2.2 fold increased risk for myocardial infarct when compared with non-carriers.55) Analysis of a specific APOA5 variant (1131T>C) that regulates pathways of TG metabolism showed an association with coronary risk. The increase in risk per allele was also concordant with data from observational studies.56) Taken together, these recent studies have provided powerful evidence that plasma levels of TRLs are causally related to the development of CAD and specifically that apoC-III promotes and APOA5 protects against CAD.43) In addition, these offer strong support to the hypothesis that intervention to lower TRL levels may decrease the risk of CAD.
Taken together, genetic studies strongly support the theory that high concentrations of TRLs or remnant cholesterol are causal risk factors for cardiovascular disease and all-cause mortality, and that low HDL cholesterol might merely be a long-term marker of raised TG and remnant cholesterol.11)
First, secondary causes of hypertriglyceridemiasuch as nephrotic syndrome, alcohol, or obesity should be ruled out and treated. In addition, intensive lifestyle intervention is the fundamental first step in the management of patients with hypertriglyceridemia or cardiometabolic disease. This includes to smoking cessation, increased physical activity, adoption of a Mediterranean style diet, replacing saturated fats with unsaturated ones, reduction of carbohydrate content in the diet, avoidance of refined sugar and fructose, increased intake of complex carbohydrates with high soluble fiber, weight loss and limited alcohol intake. If TG levels remain elevated, pharmacological approaches including fibrates and omega-3 fatty acids are considered.
Fibric acid is a synthetic ligand of the nuclear receptor PPAR α that is highly expressed in skeletal muscle and the heart where it promotes β-oxidation of fatty acids to mediate hypolipidemic actions. PPAR α regulates expressions of key proteins involved in atherogenesis, vascular inflammation, plaque stability, and thrombosis.57)58) Thus, PPAR α may exert direct anti-atherogenic actions in the vascular wall. Fibrate, PPAR α agonist, therapy significantly improves the lipoprotein profile and the flow-mediated dilator response to hyperemia, reduces levels of inflammatory markers, increases adiponectin levels, and improves insulin sensitivity.57)58)59)60) The beneficial effects of fibrate on endothelial function, inflammation, and insulin sensitivity are highly relevant to CVD and are likely to simultaneously improve both cardiovascular and metabolic health in patients with hypertriglyceridemia. Fibrate therapy improved clinical outcomes in primary and secondary prevention trials especially in patients with low HDL cholesterol and high triglycerides, despite the use of established therapy.61)62)Although the combination of statins and gemfibrozil is more likely to be accompanied by myopathy due to significant pharmacokinetic interactions with statins,63) fenofibrate showed no significant side effects with combination treatment with statins.64)65) Fenofibrate did not reduce events overall in the trials, except in patients with high triglycerides and low HDL cholesterol subgroups. However, the failure of fenofibrate in other patients has been blamed due to several issues such as pretty low triglycerides levels.11) On the other hand, we discussed off-target effects of fibrates such as anti-inflammatory effect.5758)59)60) Indeed, while fibrates appear to work, their benefit does not associate with TG lowering. In other words, a high TG patient may be more likely to benefit, but not necessarily due to TG lowering itself.
Omega-3 fatty acids consumption also improves triglycerides level and endothelial function, however, demonstrates inconsistent effects on inflammation, insulin resistance, and hemostasis.58)66) Although omega-3 fatty acids are bioactive compounds that play a significant role in cardiovascular and metabolic health and produce modest reductions in the rates of cardiovascular death in earlier randomized clinical trials, however, recent double-blind, randomized, clinical trials failed to show beneficial effects on cardiovascular events under modern guideline therapy.67)68) Further, omega-3 fatty acid or fish consumption was associated with a modestly higher incidence of type 2 diabetes in observational studies.69)70) However, this untoward effect seems to be neutral.
Taken together, fibrates might play an important role in the reduction of residual cardiovascular risk by favorable lipid profiles, endothelial function, inflammation and metabolic effects in patients with cardiovascular risks especially low HDL cholesterol and high triglycerides.3)11)58)62) However, a large-scaled clinical trial to investigate the effects of fibrate based on statin in patients with low HDL cholesterol and high TG level on the cardiovascular outcomes will be needed in the future. Though some studies reported no different effects of high dose omega-3 fatty acids except the reduction of triglycerides level,71) the role of omega-3 fatty acids, particularly a high dose, will be further elucidated with regard to the reduction of residual cardiovascular risk in the future. Three ongoing randomized controlled clinical trials (the Rischio and Prevenzione study),72) a Study of Cardiovascular Events in Diabetes (ASCEND, NCT00135226), the Vitamin D and Omega-3 Trial (VITAL, NCT01169259)73) in patients with low cardiovascular risk will further answer this question. In patients with high cardiovascular risk, reduction of cardiovascular events with EPA-intervention trial (REDUCE-IT, NCT 01492361) and outcomes study to assess residual risk reduction with epanova (STRENGTH, NCT02104817) are ongoing and will answer the effectiveness of high dose omega-3 fatty acids added on statin treatment for the cardiovascular outcomes.11)
Niacin has a broad spectrum of lipid modifying activity which include HDL cholesterol raising and TG lowering effects.74) Although previous studies reported niacin had shown attenuation of atherosclerosis progression and reduction of non-fatal myocardial infarction and all-cause mortality,75)76) niacin has now been withdrawn following reports of safety issues in the HPS2-THRIVE trial.77) Bile acid binding resins are indicated for treatment of elevated plasma LDL cholesterol levels. Although hypertriglyceridemic patients with elevated LDL cholesterol levels are indicated the use of bile acid binding resins, most hypertriglyceridemia patients without elevated LDL cholesterol levels are contraindicated due to no effect or mild elevation of TG or an adverse effect on plasma TG concentration.78) Because CETP promotes exchange of cholesterol and TG between HDL and TRL, LDL and CETP inhibitors are expected to have anti-atherogenic effects by increasing the concentration of cholesterol in the protective HDL fraction, and decreasing proatherogenic LDLs or the cholesterol content of TRLs.79) However, recent large scaled randomized clinical trials such as ILLUMINATE and dal-OUTCOMES failed due to safety concerns or futility in major outcomes.80)81) It was explained that it was mainly due to adverse off-target effects or dysfunctional HDL. Therefore, currently CETP inhibitors are not recommended to management of hypertriglyceridemia. In addition, we need to wait the results of on-going clinical trials of CETP inhibitors including Randomized Evaluation of the Effects of Anacetrapib Through Lipid-modification (NCT01252953) and A Study of Evacetrapib in High-Risk Vascular Disease (ACCELERATE, NCT01687998).82)
ISIS 304801 is a second generation antisense inhibitor of APOC3 synthesis. This small chemically-modified oligonucleotide is delivered subcutaneously and is internalized in the liver where it inhibits the translation of APOC3 mRNA and promotes mRNA degradation through activation of RNase H.43) A randomized, double-blind, placebo controlled, dose ranging, phase 2 study revealed that treatment with ISIS 304801, at doses ranging from 100 to 300 mg, once weekly for 13 week, resulted in dose-dependent and prolonged decreases in plasma APOC3 levels when the drug was administered as a single agent.45) In addition, when it was administered as in combination with fibrates, treatment with ISIS 304801 resulted in reduction of APOC3 levels. Concordant reductions of 31.3 to 70.9% were observed in TG levels without any safety concerns. This study provides evidence for a causal relationship between APOC3 and TG metabolism. In addition, it supports the continued development of ISIS304801 for the treatment of patients who remain at risk for cardiovascular events and pancreatitis because of very high TG levels.
Like apoC-III, ANGPTL3 and ANGPTL4 are thought to inhibit LPL activity, leading to elevate plasma TG levels. Pharmacological inhibition of these ANGPTLs could reduce plasma TG by a mechanism similar to that of anti-APOC3-focused therapies and result in reduced CAD risk.43) However, recent evidence linking ANGPTL3 and ANGPTLs loss of function to CAD risk has been smaller or inconsistent.83)84)85)
Lomitapide, an MTP inhibitor that interferes with apoB containing lipoprotein assembly in the apoB100 and apoB48 pathway, reduces both chylomicron and VLDL secretion.32) It is currently available for the management of homozygous familial hypercholesterolemia to treat a patient with extremely severe hypertriglyceridemia due to LPL deficiency.86) The limitation of lomitapide treatment is the possibility of developing steatohepatitis and fibrosis. The research for DGAT1 and LPL gene replacement treatment is still being tested.
Residual cardiovascular risk still exists even after optimal medical treatment including high dose statin treatment. After failure of HDL cholesterol raising treatment and genetic studies on HDL cholesterol, concern of the role of TRLs and remnant cholesterol regarding cardiovascular risk are increasing. Experimental, epidemiological and genetic evidence support the harmful effects of TRLs and remnant cholesterol. To reduce risk by hypertriglyceridemia, lifestyle intervention plays a key role. However, when hypertriglyceridemia or high levels of TRLs or non-HDL cholesterol are not corrected after lifestyle intervention with statin treatment, current available drugs such as fibrates are options for management of hypertriglyceridemia. Omega-3 fatty acids should be held until ongoing clinical trials are reported. In addition, new treatment options such as antisense inhibition of apolipoprotein C-III, ANGPTLs are being tested. The effectiveness of treatment of hypertriglyceridemia on cardiovascular outcomes will be answered in the future.
There is increasing evidence of the role of hypertriglyceridemia, TRLs and remnant cholesterol on cardiovascular outcomes. Effort for reducing levels of TG and TRLs including correction of secondary cause of hypertriglyceridemia and lifestyle intervention, fibrates, omega-3 fatty acids and new treatment options are encouraging. Randomized clinical trials of these treatments on beneficial cardiovascular outcomes are ongoing and will answer the exact role of treatment of TG and TRLs in the future.
Figures and Tables
Fig
The production of TRLs, remnant cholesterol which induces the formation of atherosclerosis. TRLs include chylomicrons, VLDL and IDL. Their major protein component is apolipoprotein B. In the fasting state, only VLDL and IDL are present in plasma, whereas chylomicrons, VLDL and their remnants circulate under non-fasting conditions. TRLs undergoes intravascular hydrolysis by lipoprotein lipase in muscle, adipose tissue, heart and other tissues, where they provide free fatty acids for energy or storage. Upon lipolysis, chylomicrons and VLDL are depleted of TG and enriched in cholesterol, resulting in the formation of chylomicron remnants and VLDL remnants. CETP mediates major lipid transfer and exchange between HDL and TRLs. During this process, cholesteryl esters are transferred from HDL to TRLs and TG move from TRLs to HDL. In addition, the plasma HDL pool involves hydrolysis of TG in VLDL, IDL, and chylomicrons. In this process, which is catalyzed by LPL, phospholipids as well as several apolipoproteins (such as apoCI, CII, CIII) are transferred to HDL. Since most cells can degrade TG, and there are not any cells that can degrade cholesterol, the cholesterol content of TRLs is more likely to be the cause of atherosclerosis and cardiovascular disease rather than raised TG per se. Indeed, cholesterol, rather than TG accumulates in intimal foam cells and in atherosclerotic plaques, and remnant lipoproteins like LDL can enter the arterial intima. In contrast, chylomicrons are too large to enter. LPL activity at the surface of remnant particles, either at the surface of vascular endothelium or within the intima, leads to liberation of free fatty acids, monoacylglycerols, and other molecules, each of which could cause local injury and inflammation. Although other possible mechanisms have been suggested, perhaps the simplest chain of events is that high triglyceride concentrations are a marker for raised remnants rich in cholesterol, which, upon entrance into the intima, leads to low-grade inflammation, foam cell formation, atherosclerotic plaques, and ultimately cardiovascular disease and increased mortality.11) TRLs: triglyceride rich lipoproteins, VLDL: very large density lipoprotein, IDL: intermediate density lipoprotein, TG: triglyceride, LDL: low density lipoprotein, CETP: cholesteryl ester transfer protein, HDL: high density lipoprotein, LPL: lipoprotein lipase, CE: cholesterol ester.
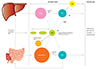
Table 1
Causal and observational risk estimates for ischemic heart disease16)
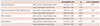
Table 2
Studies for the use of non-fasting TG and remnant cholesterol levels as predictors of CVD events
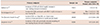
Primary endpoint | Sample size | Primary endpoint Hazard ratio (95% CI) | |
---|---|---|---|
Varbo et al.16) | Incidence of CVD with 88.41 mg/dL increase in non-fasting remnant cholesterol | 73513 | 1.4 (1.3-1.5) |
Nordestgaard et al.27) | Incident MI, CVD, and death with 88.41 mg/dL increase in non-fasting TG | 13981 |
Men 1.14 (1.10-1.19) Women 1.30 (1.22-1.40) |
The Women's Health Study28) | Incident CVD, non-fatal MI, non-fatal stroke, coronary revascularization or cardiovascular death | 26509 | 2.53 (1.69-3.79) |
Jørgensen et al.30) | Incidence of MI with genetically elevated doubling of non-fasting TG and remnant cholesterol | 10391 | 1.87 (1.25-2.81) |
Table 3
Observational and causal (by use of genetics) associations of raised remnant cholesterol and TG with risk of ischemic heart disease, myocardial infarction and all cause mortality11)
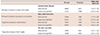
Acknowledgments
Dr. Koh holds a certificate of patent, 10-1579656 (A pharmaceutical composition comprising pravastatin and valsartan).
References
1. Stone NJ, Robinson JG, Lichtenstein AH, et al. 2013 ACC/AHA guideline on the treatment of blood cholesterol to reduce atherosclerotic cardiovascular risk in adults: a report of the American College of Cardiology/American Heart Association task force on practice guidelines. J Am Coll Cardiol. 2014; 63(25 Pt B):2889–2934.
2. Mora S, Wenger NK, DeMicco DA, et al. Determinants of residual risk in secondary prevention patients treated with high- versus low-dose statin therapy: the treating to new targets (TNT) study. Circulation. 2012; 125:1979–1987.
3. Lim S, Park YM, Sakuma I, Koh KK. How to control residual cardiovascular risk despite statin treatment: Focusing on HDL–cholesterol. Int J Cardiol. 2013; 166:8–14.
4. Koh KK. How to control residual risk during statin era? J Am Coll Cardiol. 2015; 66:1848.
5. Mora S, Caulfield MP, Wohlgemuth J, et al. Atherogenic lipoprotein subfractions determined by ion mobility and first cardiovascular events after random allocation to high-intensity statin or placebo: the JUPITER trial. Circulation. 2015; 132:2220–2229.
6. Lee MH, Kim HC, Ahn SV, et al. Prevalence of dyslipidemia among Korean adults: Korea National Health and Nutrition Survey 1998-2005. Diabetes Metab J. 2012; 36:43–55.
7. Kim K. Distribution of blood cholesterol profile in untreated Korean population. Korean Circ J. 2015; 45:108–109.
8. Park JH, Lee MH, Shim JS, et al. Effects of age, sex, and menopausal status on blood cholesterol profile in the Korean population. Korean Circ J. 2015; 45:141–148.
9. Ren J, Grundy SM, Liu J, et al. Long-term coronary heart disease risk associated with very-low-density lipoprotein cholesterol in Chinese: the results of a 15-Year Chinese Multi-Provincial Cohort Study (CMCS). Atherosclerosis. 2010; 211:327–332.
10. Lim S, Shin H, Song JH, et al. Increasing prevalence of metabolic syndrome in Korea: the Korean National Health and Nutrition Examination Survey for 1998-2007. Diabetes Care. 2011; 34:1323–1328.
11. Nordestgaard BG, Varbo A. Triglycerides and cardiovascular disease. Lancet. 2014; 384:626–635.
12. Nordestgaard BG, Wootton R, Lewis B. Selective retention of VLDL, IDL, and LDL in the arterial intima of genetically hyperlipidemic rabbits in vivo. Molecular size as a determinant of fractional loss from the intima-inner media. Arterioscler Thromb Vasc Biol. 1995; 15:534–542.
13. Goldberg IJ, Eckel RH, McPherson R. Triglycerides and heart disease: still a hypothesis? Arterioscler Thromb Vasc Biol. 2011; 31:1716–1725.
14. Proctor SD, Vine DF, Mamo JC. Arterial retention of apolipoprotein B(48)- and B(100)-containing lipoproteins in atherogenesis. Curr Opin Lipidol. 2002; 13:461–470.
15. Chapman MJ, Ginsberg HN, Amarenco P, et al. Triglyceride-rich lipoproteins and high-density lipoprotein cholesterol in patients at high risk of cardiovascular disease: evidence and guidance for management. Eur Heart J. 2011; 32:1345–1361.
16. Varbo A, Benn M, Tybjærg-Hansen A, Jørgensen AB, Frikke-Schmidt R, Nordestgaard BG. Remnant cholesterol as a causal risk factor for ischemic heart disease. J Am Coll Cardiol. 2013; 61:427–436.
17. Hegele RA, Ginsberg HN, Chapman MJ, et al. The polygenic nature of hypertriglyceridaemia: implications for definition, diagnosis, and management. Lancet Diabetes Endocrinol. 2014; 2:655–666.
18. Rapp JH, Lespine A, Hamilton RL, et al. Triglyceride-rich lipoproteins isolated by selected-affinity anti-apolipoprotein B immunosorption from human atherosclerotic plaque. Arterioscler Thromb. 1994; 14:1767–1774.
19. Alaupovic P, Mack WJ, Knight-Gibson C, Hodis HN. The role of triglyceride-rich lipoprotein families in the progression of atherosclerotic lesions as determined by sequential coronary angiography from a controlled clinical trial. Arterioscler Thromb Vasc Biol. 1997; 17:715–722.
20. Zheng XY, Liu L. Remnant-like lipoprotein particles impair endothelial function: direct and indirect effects on nitric oxide synthase. J Lipid Res. 2007; 48:1673–1680.
21. Alipour A, van Oostrom AJ, Izraeljan A, et al. Leukocyte activation by triglyceride-rich lipoproteins. Arterioscler Thromb Vasc Biol. 2008; 28:792–797.
22. Wang L, Gill R, Pedersen TL, Higgins LJ, Newman JW, Rutledge JC. Triglyceride-rich lipoprotein lipolysis releases neutral and oxidized FFAs that induce endothelial cell inflammation. J Lipid Res. 2009; 50:204–213.
23. Moyer MP, Tracy RP, Tracy PB, van't Veer Cvt, Sparks CE, Mann KG. Plasma lipoproteins support prothrombinase and other procoagulant enzymatic complexes. Arterioscler Thromb Vasc Biol. 1998; 18:458–465.
24. Kohler HP, Grant PJ. Plasminogen-activator inhibitor type 1 and coronary artery disease. N Engl J Med. 2000; 342:1792–1801.
25. Patel A, Barzi F, Jamrozik K, et al. Serum triglycerides as a risk factor for cardiovascular diseases in the Asia-Pacific Region. Circulation. 2004; 110:2678–2686.
26. Sarwar N, Danesh J, Eiriksdottir G, et al. Triglycerides and the risk of coronary heart disease 10,158 incident cases among 262,525 participants in 29 western prospective studies. Circulation. 2007; 115:450–458.
27. Nordestgaard BG, Benn M, Schnohr P, Tybjærg-Hansen A. Nonfasting triglycerides and risk of myocardial infarction, ischemic heart disease, and death in men and women. JAMA. 2007; 298:299–308.
28. Bansal S, Buring JE, Rifai N, Mora S, Sacks FM, Ridker PM. Fasting compared with nonfasting triglycerides and risk of cardiovascular events in women. JAMA. 2007; 298:309–316.
29. Langsted A, Freiberg J, Tybjærg-Hansen A, Schnohr P, Jensen GB, Nordestgaard B. Nonfasting cholesterol and triglycerides and association with risk of myocardial infarction and total mortality: the Copenhagen City Heart Study with 31 years of follow-up. J Intern Med. 2011; 270:65–75.
30. Jørgensen AB, Frikke-Schmidt R, West AS, Grande P, Nordestgaard BG, Tybjærg-Hansen A. Genetically elevated non-fasting triglycerides and calculated remnant cholesterol as causal risk factors for myocardial infarction. Eur Heart J. 2013; 34:1826–1833.
31. Emerging Risk Factors Collaboration. Di Angelantoni E, Sarwar N, et al. Major lipids, apolipoproteins, and risk of vascular disease. JAMA. 2009; 302:1993–2000.
32. Rosenson RS, Davidson MH, Hirsh BJ, Kathiresan S, Gaudet D. Genetics and causality of triglyceride-rich lipoproteins in atherosclerotic cardiovascular disease. J Am Coll Cardiol. 2014; 64:2525–2540.
33. Schwartz GG, Abt M, Bao W, et al. Fasting triglycerides predict recurrent ischemic events in patients with acute coronary syndrome treated with statins. J Am Coll Cardiol. 2015; 65:2267–2275.
34. Jackson KG, Poppitt SD, Minihane AM. Postprandial lipemia and cardiovascular disease risk: interrelationships between dietary, physiological and genetic determinants. Atherosclerosis. 2012; 220:22–33.
35. Rip J, Nierman MC, Ross CJ, et al. Lipoprotein lipase S447X a naturally occurring gain-of-function mutation. Arterioscler Thromb Vasc Biol. 2006; 26:1236–1245.
36. Humphries SE, Nicaud V, Margalef J, Tiret L, Talmud PJ. Lipoprotein lipase gene variation is associated with a paternal history of premature coronary artery disease and fasting and postprandial plasma triglycerides: the European Atherosclerosis Research Study (EARS). Arterioscler Thromb Vasc Biol. 1998; 18:526–534.
37. Wittrup HH, Tybjærg-Hansen A, Nordestgaard BG. Lipoprotein lipase mutations, plasma lipids and lipoproteins, and risk of ischemic heart disease. A meta-analysis. Circulation. 1999; 99:2901–2907.
38. Henderson HE, Kastelein JJ, Zwinderman AH, et al. Lipoprotein lipase activity is decreased in a large cohort of patients with coronary artery disease and is associated with changes in lipids and lipoproteins. J Lipid Res. 1999; 40:735–743.
39. Lettre G, Palmer CD, Young T, et al. Genome-wide association study of coronary heart disease and its risk factors in 8,090 African Americans: the NHLBI CARe project. PLoS Genet. 2011; 7:e1001300.
40. Teslovich TM, Musunuru K, Smith AV, et al. Biological, clinical and population relevance of 95 loci for blood lipids. Nature. 2010; 466:707–713.
41. Waterworth DM, Ricketts SL, Song K, et al. Genetic variants influencing circulating lipid levels and risk of coronary artery disease. Arterioscler Thromb Vasc Biol. 2010; 30:2264–2276.
42. Do R, Willer CJ, Schmidt EM, et al. Common variants associated with plasma triglycerides and risk for coronary artery disease. Nat Genet. 2013; 45:1345–1352.
43. Khetarpal SA, Rader DJ. Triglyceride-rich lipoproteins and coronary artery disease risk: new insights from human genetics. Arterioscler Thromb Vasc Biol. 2015; 35:e3–e9.
44. Ooi EM, Barrett PH, Chan DC, Watts GF. Apolipoprotein C-III: understanding an emerging cardiovascular risk factor. Clin Sci (Lond). 2008; 114:611–624.
45. Gaudet D, Alexander VJ, Baker BF, et al. Antisense inhibition of apolipoprotein C-III in patients with hypertriglyceridemia. N Engl J Med. 2015; 373:438–447.
46. Kawakami A, Osaka M, Tani M, et al. Apolipoprotein CIII links hyperlipidemia with vascular endothelial cell dysfunction. Circulation. 2008; 118:731–742.
47. Abe Y, Kawakami A, Osaka M, et al. Apolipoprotein CIII induces monocyte chemoattractant protein-1 and interleukin 6 expression via Toll-like receptor 2 pathway in mouse adipocytes. Arterioscler Thromb Vasc Biol. 2010; 30:2242–2248.
48. Qamar A, Khetarpal SA, Khera AV, Qasim A, Rader DJ, Reilly MP. Plasma apolipoprotein C-III levels, triglycerides, and coronary artery calcification in type 2 diabetics. Arterioscler Thromb Vasc Biol. 2015; 35:1880–1888.
49. TG and HDL Working Group of the Exome Sequencing Project, National Heart, Lung, and Blood Institute. Crosby J, Peloso GM, et al. Loss-of-function mutations in APOC3, triglycerides, and coronary disease. N Engl J Med. 2014; 371:22–31.
50. Jørgensen AB, Frikke-Schmidt R, Nordestgaard BG, Tybjærg-Hansen A. Loss-of-function mutations in APOC3 and risk of ischemic vascular disease. N Engl J Med. 2014; 371:32–41.
51. Pennacchio LA, Olivier M, Hubacek JA, et al. An apolipoprotein influencing triglycerides in humans and mice revealed by comparative sequencing. Science. 2001; 294:169–173.
52. Tang Y, Sun P, Guo D, et al. A genetic variant c.553G > T in the apolipoprotein A5 gene is associated with an increased risk of coronary artery disease and altered triglyceride levels in a Chinese population. Atherosclerosis. 2006; 185:433–437.
53. Johansen CT, Wang J, Lanktree MB, et al. Excess of rare variants in genes identified by genome-wide association study of hypertriglyceridemia. Nat Genet. 2010; 42:684–687.
54. Soufi M, Sattler AM, Kurt B, Schaefer JR. Mutation screening of the APOA5 gene in subjects with coronary artery disease. J Investig Med. 2012; 60:1015–1019.
55. Do R, Stitziel NO, Won HH, et al. Exome sequencing identifies rare LDLR and APOA5 alleles conferring risk for myocardial infarction. Nature. 2015; 518:102–106.
56. Triglyceride Coronary Disease Genetics Consortium and Emerging Risk Factors collaboration. Sarwar N, Sandhu MS, et al. Triglyceride-mediated pathways and coronary disease: collaborative analysis of 101 studies. Lancet. 2010; 375:1634–1639.
57. Han SH, Quon MJ, Koh KK. Beneficial vascular and metabolic effects of peroxisome proliferator-activated receptor-alpha activators. Hypertension. 2005; 46:1086–1092.
58. Han SH, Oh PC, Lim S, Eckel RH, Koh KK. Comparative cardiometabolic effects of fibrates and omega-3 fatty acids. Int J Cardiol. 2013; 167:2404–2411.
59. Koh KK, Ahn JY, Han SH, et al. Effects of fenofibrate on lipoproteins, vasomotor function, and serological markers of inflammation, plaque stabilization, and hemostasis. Atherosclerosis. 2004; 174:379–383.
60. Koh KK, Han SH, Quon MJ, Ahn JY, Shin EK. Beneficial effects of fenofibrate to improve endothelial dysfunction and raise adiponectin levels in patients with primary hypertriglyceridemia. Diabetes Care. 2005; 28:1419–1424.
61. Jun M, Foote C, Lv J, et al. Effects of fibrates on cardiovascular outcomes: a systematic review and meta-analysis. Lancet. 2010; 375:1875–1884.
62. Sacks FM, Carey VJ, Fruchart JC. Combination lipid therapy in type 2 diabetes. N Engl J Med. 2010; 363:692–694. author reply 694-5.
63. Taher TH, Dzavik V, Reteff EM, Pearson GJ, Woloschuk BL, Francis GA. Tolerability of statin-fibrate and statin-niacin combination therapy in dyslipidemic patients at high risk for cardiovascular events. Am J Cardiol. 2002; 89:390–394.
64. Koh KK, Quon MJ, Han SH, et al. Additive beneficial effects of fenofibrate combined with atorvastatin in the treatment of combined hyperlipidemia. J Am Coll Cardiol. 2005; 45:1649–1653.
65. ACCORD Study Group. Ginsberg HN, Elam MB, et al. Effects of combination lipid therapy in type 2 diabetes mellitus. N Engl J Med. 2010; 362:1563–1574.
66. Koh KK, Quon MJ, Shin KC, et al. Significant differential effects of omega-3 fatty acids and fenofibrate in patients with hypertriglyceridemia. Atherosclerosis. 2012; 220:537–544.
67. Kromhout D, Giltay EJ, Geleijnse JM. Alpha Omega Trial Group. n-3 fatty acids and cardiovascular events after myocardial infarction. N Engl J Med. 2010; 363:2015–2026.
68. Kwak SM, Myung SK, Lee YJ, Seo HG. Korean Meta-analysis Study Group. Efficacy of omega-3 fatty acid supplements (eicosapentaenoic acid and docosahexaenoic acid) in the secondary prevention of cardiovascular disease: a meta-analysis of randomized, double-blind, placebo-controlled trials. Arch Intern Med. 2012; 172:686–694.
69. Kaushik M, Mozaffarian D, Spiegelman D, Manson JE, Willett WC, Hu FB. Long-chain omega-3 fatty acids, fish intake, and the risk of type 2 diabetes mellitus. Am J Clin Nutr. 2009; 90:613–620.
70. Djoussé L, Gaziano JM, Buring JE, Lee IM. Dietary omega-3 fatty acids and fish consumption and risk of type 2 diabetes. Am J Clin Nutr. 2011; 93:143–150.
71. Oh PC, Koh KK, Sakuma I, et al. Omega-3 fatty acid therapy dose-dependently and significantly decreased triglycerides and improved flow-mediated dilation, however, did not significantly improve insulin sensitivity in patients with hypertriglyceridemia. Int J Cardiol. 2014; 176:696–702.
72. Rischio and Prevenzione Investigators. Efficacy of n-3 polyunsaturated fatty acids and feasibility of optimizing preventive strategies in patients at high cardiovascular risk: rationale, design and baseline characteristics of the Rischio and Prevenzione study, a large randomised trial in general practice. Trials. 2010; 11:68.
73. Manson JE, Bassuk SS, Lee IM, et al. The VITamin D and OmegA-3 TriaL (VITAL): rationale and design of a large randomized controlled trial of vitamin D and marine omega-3 fatty acid supplements for the primary prevention of cancer and cardiovascular disease. Contemp Clin Trials. 2012; 33:159–171.
74. Chapman MJ, Redfern JS, McGovern ME, Giral P. Niacin and fibrates in atherogenic dyslipidemia: pharmacotherapy to reduce cardiovascular risk. Pharmacol Ther. 2010; 126:314–345.
75. Clofibrate and niacin in coronary heart disease. JAMA. 1975; 231:360–381.
76. Canner PL, Berge KG, Wenger NK, et al. Fifteen year mortality in Coronary Drug Project patients: long-term benefit with niacin. J Am Coll Cardiol. 1986; 8:1245–1255.
77. HPS2-THRIVE Collaborative Group. Landray MJ, Haynes R, et al. Effects of extended-release niacin with laropiprant in high risk patients. N Engl J Med. 2014; 371:203–212.
78. Crouse JR 3rd. Hypertriglyceridemia: a contraindication to the use of bile acid binding resins. Am J Med. 1987; 83:243–248.
79. Chapman MJ, Le Goff W, Guerin M, Kontush A. Cholesteryl ester transfer protein: at the heart of the action of lipid-modulating therapy with statins, fibrates, niacin, and cholesteryl ester transfer protein inhibitors. Eur Heart J. 2010; 31:149–164.
80. Barter PJ, Caulfield M, Eriksson M, et al. Effects of torcetrapib in patients at high risk for coronary events. N Engl J Med. 2007; 357:2109–2122.
81. Schwartz GC, Olsson AG, Abt M, et al. Effects of dalcetrapib in patients with a recent acute coronary syndrome. New Engl J Med. 2012; 367:2089–2099.
82. Nicholls SJ, Lincoff AM, Barter PJ, et al. Assessment of the clinical effects of cholesteryl ester transfer protein inhibition with evacetrapib in patients at high-risk for vascular outcomes: rationale and design of the ACCELERATE trial. Am Heart J. 2015; 170:1061–1069.
83. Korstanje R, Eriksson P, Samnegård A, et al. Locating Ath8, a locus for murine atherosclerosis susceptibility and testing several of its candidate genes in mice and humans. Atherosclerosis. 2004; 177:443–450.
84. Hatsuda S, Shoji T, Shinohara K, et al. Association between plasma angiopoietin-like protein 3 and arterial wall thickness in healthy subjects. J Vasc Res. 2007; 44:61–66.
85. Talmud PJ, Smart M, Presswood E, et al. ANGPTL4 E40K and T266M: effects on plasma triglyceride and HDL levels, postprandial responses, and CHD risk. Arterioscler Thromb Vasc Biol. 2008; 28:2319–2325.
86. Sacks FM, Stanesa M, Hegele RA. Severe hypertriglyceridemia with pancreatitis: thirteen years' treatment with lomitapide. JAMA Intern Med. 2014; 174:443–447.