Abstract
Background and Objectives
Subjects and Methods
Results
Figures and Tables
Fig. 1
Association of right ventricular longitudinal strain (RVLS) with clinical symptoms. Patients with advanced symptoms (NYHA Fc III/IV) have significantly impaired global right ventricular longitudinal strain (RVLSglobal) value. Error bar equals 1SD. RV: right ventricle, NYHA Fc: New York Heart Association functional class.
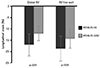
Fig. 2
Correlations between A) right ventricular global longitudinal strain of right ventricle and 6-minute walking distance; B) right ventricular global longitudinal strain of right ventricle and logarithmic transformation of B-type natriuretic peptide. C) global longitudinal strain of right ventricular free wall and 6-minute walking distance; D) global longitudinal strain of right ventricular free wall and logarithmic transformation of B-type natriuretic peptide. RVLSglobal: Right ventricular global longitudinal strain of right ventricle, RVLSFW: global longitudinal strain of right ventricular free wall, LogBNP: B-type natriuretic polypeptide concentration expressed as a logarithm of its value, RV: right ventricle.
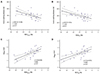
Fig. 3
Correlations between A) right ventricular global longitudinal strain and mean pulmonary arterial pressure; B) global longitudinal strain of right ventricular free wall and mean pulmonary arterial pressure; C) right ventricular global longitudinal strain and cardiac index; D) global longitudinal strain of right ventricular free wall and cardiac index; E) right ventricular global longitudinal strain and pulmonary vascular resistance; F) global longitudinal strain of right ventricular free wall and pulmonary vascular resistance. mPAP: mean pulmonary arterial pressure, RVLSglobal: right ventricular global longitudinal strain of right ventricle, RVLSFW: global longitudinal strain of right ventricular free wall, PVR: pulmonary vascular resistance.
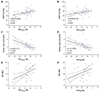
Fig. 4
Correlations between the changes of hemodynamic parameters and right ventricular global longitudinal strain with specific pulmonary vasodilator therapy. Change of variables was derived by subtraction of follow-up value from baseline value, and positive values indicate better response. A) change in right ventricular global longitudinal strain of right ventricle versus change in mean pulmonary arterial pressure; B) change in global longitudinal strain of right ventricular free wall versus change in mean pulmonary arterial pressure; C) change in right ventricular global longitudinal strain of right ventricle versus change in pulmonary vascular resistance; D) change in global longitudinal strain of right ventricular free wall versus change in pulmonary vascular resistance. mPAP: mean pulmonary arterial pressure, RVLSglobal: right ventricular global longitudinal strain of right ventricle, PVR: pulmonary vascular resistance, WU: wood units, RVLSFW: global longitudinal strain of right ventricular free wall.
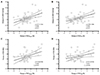
Fig. 5
RVLSglobal during follow up. Data are presented at baseline, within the first 6 months, and after more than a year after initial echocardiogram. Patients were separated into the group already on treatment for pulmonary hypertension (n=6), and a group of in whom treatment was initiated or in intensified (n=28) after the baseline echocardiogram. Patients who were already on treatment had better RVLSglobal (p=0.04) during the study period. However, patients in whom treatment was initiated or intensified showed significant improvement in RVLSglobal during follow up (p=0.03). Error bars show 1 standard deviation. RVLSglobal: right ventricular global longitudinal strain of right ventricle.
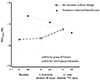
Table 1
Baseline characteristics (n=34)
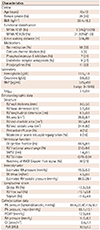
BMI: body mass index, NYHA FC: New York Heart Association Functional Class, BNP: B-type natriuretic peptide, LogBNP: B-type natriuretic polypeptide concentration expressed as a logarithm of its value. RV: right ventricle, RA: right atrium, LV: left ventricle, TAPSE: tricuspid annular plane systolic excursion, RVOT: right ventricular outflow tract, TR: tricuspid regurgitation, PA: pulmonary artery, PCWP: pulmonary capillary wedge pressure, CI: cardiac index, PVR: pulmonary vascular resistance, WU: wood units
Table 2
Echocardiographic and invasive parameters prior to and after the treatment (n=25)
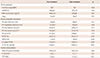
6MWD: 6-minute walking distance, BNP: B-type natriuretic peptide, RV: right ventricle, RA: right atrium, RVFAC: right ventricular fractional area change, TAPSE: tricuspid annular plane systolic excursion, LS: longitudinal strain, PA: pulmonary artery, CI: cardiac index, PVR: pulmonary vascular resistance, WU: wood units