Abstract
Background and Objectives
Previously, various methodologies were used to enumerate the endothelial progenitor cells (EPCs). We now know that these methodologies enumerate at least three different EPC subsets: circulating angiogenic cells (CACs), colony-forming unit endothelial cells (CFU-ECs), and endothelial colony-forming cells (ECFCs). It is not clear whether there is a correlation between changes in the number of these subsets. The aim of the current study is to find an answer to this question.
Materials and Methods
The number of all EPC subsets was quantified in the peripheral blood of nine pregnant women in their first and third trimesters of pregnancy. We enumerated 14 cell populations by quantitative flow-cytometry using various combinations of the markers, CD34, CD133, CD309, and CD45, to cover most of the reported phenotypes of CACs and ECFCs. Culturing technique was used to enumerate the CFU-ECs. Changes in the number of cells were calculated by subtracting the number of cells in the first trimester peripheral blood from the number of cells in the third trimester peripheral blood, and correlations between these changes were analyzed.
Results
The number of CFU-ECs did not correlate with the number of ECFCs and CACs. Also, CACs and ECFCs showed independent behaviors. However, the number of CACs showed a strong correlation with the number of CD133+CD309+ cells (p=0.001) and a moderate correlation with the number of CD34+CD309+ cells (p=0.042). Also, the number of ECFCs was correlated with the number of CD309+CD45- cells (p=0.029) and CD34+CD45- cells (p=0.03).
The dominant paradigm indicating that in adults, the formation of new blood vessels occurs only by migration and proliferation of mature endothelial cells (ECs) in a process termed 'angiogenesis' was overturned in recent years by the discovery of endothelial progenitor cells (EPCs), which differentiate into ECs in a process referred to as vasculogenesis.1) Currently, several assessment techniques and definitions have been proposed for EPCs, since they were first described by Asahara et al.2) Hill et al.3) using culturing techniques, introduced a colony-forming assay {colony forming unit Hill (CFU-Hill's)} in the field.3) They showed that there was a significant inverse correlation between the concentration of CFU-Hill and Framingham cardiovascular risk score in human subjects. Other researchers showed that the concentration of CFUs is decreased in patients with type 1 diabetes, chronic obstructive pulmonary disease, congestive heart failure, acute stroke, rheumatoid arthritis, obesity, and many other peripheral vascular diseases.4) On the other hand, several flow cytometric assays have been proposed to measure the frequency of circulating EPCs. For example, Peichev et al.5) have suggested the use of the combination of CD34, CD133, and CD309 for the flow cytometric identification of the phenotype of EPCs th-rough indirect evidence and many investigators have used the flow cytometric method to enumerate the so-called circulating EPCs. For instance, Werner et al.6) demonstrated that a lower frequency of CD34+CD133+CD309+ cells is correlated with poorer vascular endothelial status. However, the scientific community has not reached a consensus on the combination of markers that should be used to enumerate these cells7) and various combinations have been used such as: CD34+133+,8) CD34+309+,9) CD133+45-,10) and CD133+309+.11) Furthermore, different studies presented the resultant data in two distinct forms: number of cells in the volume of blood and frequencies in the defined number of mononuclear cells (MNCs), which makes the comparison between different clinical studies more difficult.
Nevertheless, more recent data have clarified that the two mentioned methods have presented cells which cannot differentiate into ECs or assemble into vascular networks in vitro and show monocytic characteristics such as expressing CD14 and CD45. These findings demonstrate that these cells are not true EPCs.5) Eventually, Ingram et al.12) introduced cells with appropriate characteristics that can be considered as true EPCs, now called "endothelial colony-forming cells (ECFCs)". These cells lack CD14, CD45, and CD133 markers while they still express CD34 and CD309.12) Nevertheless, both CFU-Hills and circulating EPCs measured by flow cytometry are still classified as EPC subsets in the literature4) but with new names: "CFU-ECs" and "circulating angiogenic cells (CACs)", respectively.11) In addition, the strong correlation between the reduced number of CFU-ECs and CACs and increased risk of cardiovascular disease cannot be ignored, and although they are not true EPCs, they are true markers of vascular and endothelial status.11)
Now we know that these commonly used assays for measurement of the three EPC subsets quantify distinct cells,13) and whether there is a correlation between the changes in the number of these subsets is a subject of investigation. Currently, only two studies have systematically examined the correlation between some of these EPC subsets, and in both studies some assays were not included.14)15) In the current study, we have addressed this question in a prospective manner to assess the correlation between the changes in the number of all EPC subsets (CACs, ECFCs, and CFU-ECs) together. In this regard, we chose normal pregnancy as the model. Normal pregnancy is associated with enhanced endothelial function and formation of new blood vessels. Significant changes in the number of EPC subsets in the maternal circulation have been demonstrated during the three consecutive trimesters by using various mentioned methodologies.16) This gave us the chance of obtaining two samples from one person with an anticipated change in the number of EPCs. The resultant prospective approach provides a unique opportunity to measure the changes in the number of EPC subsets instead of only quantifying the absolute numbers. This is important because the changes in the number of these cells are a more realistic representation of their association with changes in the endothelial and vascular status compared to a cross-sectional enumeration.
During April 2011 to May 2012, nine healthy pregnant women were enrolled in this study and peripheral blood samples were taken in their first and third trimesters. All the participants gave their written informed consent and the study was approved by the local Ethics Committee and it conformed to the Declaration of Helsinki.
Peripheral blood samples were transferred onto the layer of lymphosep (Biosera, UckfieldEast Sussex, UK) and centrifuged at 300×g for 25 minutes without break. Finally, the buffy coat layer was extracted. The total number of the isolated cells was determined using a hemocytometer and the purified MNCs were divided into two tubes for the following steps.
Because of the low frequency of the target cells in the peripheral blood, we performed a four-color quantitative flow cytometry using the rare cell analysis protocols.17) All the MNCs purified from half of the blood sample (equivalent to 10 mL or at least 106 cells) were stained with mouse derived antibodies against human CD34 conjugated with fluorescein isothiocyanate, CD133 conjugated with Phycoerythrin, CD309 conjugated with Allophycocyanin, and CD45 conjugated with Peridinin chlorophyll protein (all obtained from Miltenyi Biotec GmbH, Bergisch Gladbach, Germany). To enumerate the cell population of interest, TruCOUNT kit (BD Biosciences, San Jose, CA, USA) was used according to the manufacturer's instructions and by using the following formula: number of events in the target region×number of beads in the tube divided by the number of events in the bead region×sample volume. Samples were analyzed by a four-color FACS-Calibur instrument (BD Biosciences). CellQuest Pro software (BD Biosciences) was used for data acquisition and WinMDI software was used for data analysis and graphical presentation of data.
To cover most of the reported phenotypes of CACs and ECFCs in the literature, we enumerated the 14 cell populations listed in Table 1.
Half of the MNCs were resuspended in the Endocult medium (Stem Cell Technologies, Vancouver, BC, Canada) supplemented with 100 U/mL penicillin and 100 µg/mL streptomycin (Gibco/Invitrogen, Carlsbad, CA, USA). The suspension was seeded into one well of 6-well fibronectin-coated plates (BD Biosciences) and incubated for two days at 37℃, 5% CO2, and 95% humidity. Then, the non-adherent cells were collected and replated onto 24-well fibronectin-coated plates (BD Biosciences). After 5 days, the culture medium was removed and the plates were washed with phosphate buffered saline. To increase the accuracy, the tests were performed in duplicate and the colonies were counted by two independent expert technicians.
The parameters analyzed for determining the correlations were calculated as follows:
"Absolute changes=Absolute number of target cells in the third trimester peripheral blood-Absolute number of target cells in the first trimester peripheral blood".
"Relative changes=(Absolute changes divided by absolute number of target cells in the first trimester peripheral blood)×100".
To cover the different methodologies for presenting the final number of EPC subsets in the literature, we calculated the number of target cells derived from flow cytometry or culturing techniques (CFU-EC assay), both in a milliliter of whole blood or in a million purified MNCs.
Eventually, we gathered 64 parameters at the end of the study. The correlations between parameters were evaluated by Spearman's correlation study. Mann-Whitney test was used wherever appropriate. P less than 0.05 were considered statistically significant. Statistical Package for the Social Sciences (SPSS) software version 19.0 for Windows (SPSS Inc., Chicago, IL, USA) was used for statistical analysis. Graphpad Prism software (Graphpad, USA) was used for graphical presentation of data.
In the present investigation, we studied the correlations between the absolute and relative changes in the number of EPC subsets in the peripheral blood samples from 9 healthy pregnant women. Some of the clinical characteristics of the patients are presented in Table 2. Flow cytometry was used to enumerate CACs (Fig. 1); precursors of CACs and ECFCs, and culture techniques were used to enumerate the number of CFU-ECs (Fig. 2). The resultant data were presented both in a milliliter of blood and in one million purified MNCs. The complete data on correlations between the absolute and relative changes are reflected in Appendices 1 and 2, respectively, and the summary of the main findings is presented in Table 3 and 4 in the same order.
Neither absolute nor relative changes in the number of CFU-ECs were correlated with those in the number of CACs or ECFCs, and their absolute changes reported in both in a milliliter of blood or in one million MNCs were inversely correlated with those in the number of CAC precursors/mL (coefficient=-0.717, p=0.03; and coefficient=-0.9, p=0.001, respectively). The relative changes in the number of ECFCs/mL and also the relative changes in the number of CAC precursors/mL were correlated with relative changes in the number of CACs/mL (coefficient=0.714, p=0.031; and coefficient=0.713, p=0.032, respectively).
Regarding the correlations between the alternatively reported phenotypes with the gold standard (Table 1), the number of CD34+CD133+CD309+CD45+ cells as the indicator of CACs, was almost invariably similar to the number of CD34+CD133+CD309+ cells with a very strong correlation (coefficient=1, p<0.001), showing that the two groups are the same. The number of CACs was also moderately correlated with the number of CD34+CD309+ cells (coefficient=0.745, p=0.042) and was very strongly correlated with the number of CD133+CD309+ cells (coefficient=0.911, p=0.001), but it was not correlated with the number of CD34+CD133+ cells (coefficient=0.478, p=0.193). On assessing the correlations of ECFCs (CD34+CD133-CD309+CD45- cells) with their alternatively reported phenotypes, we found a significant correlation of these cells with two other groups; CD309+CD45- and CD34+CD45- phenotypes (correlation coefficient=0.756 and 0.717, respectively; p=0.029 and p=0.03, respectively).
With respect to how the type of reporting the data would affect the final results, we found that the frequency of CACs in a unit of peripheral blood was significantly increased at higher gestational ages of normal pregnancies. It was 106 cells/mL in the first trimester peripheral blood, while it increased up to 239 cells/mL in the third trimester peripheral blood (p=0.016) (Fig. 3A). However, this increase was not significant when the number of CACs was calculated in one million MNCs (p=0.136) (Fig. 3B). Accordingly, the relative changes in the number of CACs/mL and CACs/106 MNCs were not correlated (coefficient=0.349, p=0.358). Furthermore, absolute changes in the number of CFU-ECs when calculated in a milliliter of whole blood and in 106 MNCs showed only modest correlations (coefficient=0.683, p=0.042), but the relative changes showed a very strong correlation (coefficient=0.987, p<0.001).
The number of EPCs in pathologic conditions is quite important as the changes in their number are strongly associated with several cardiovascular diseases. Nevertheless, there is no consensus on the definition of EPCs, and consequently a variety of surface markers and functional assays have been used to enumerate these cells. Therefore, a systematically designed study for comparing these various methodologies seems necessary. In the present study, we performed an analysis of several methods used for enumerating EPCs and correlated them with each other. We observed that the changes in the number of the most frequently measured cells in the blood (CFU-ECs, CACs, and ECFCs) are independent of each other. However, there are some interesting correlations between them and other cell populations.
With respect to the number of EPCs, one should be aware that the data in previous studies are reported in two ways: number of cells in a defined volume of blood and frequencies in a defined number of MNCs, which may lead to a significant difference in the final results. For example, in our study, the number of CACs showed an increase with advancement of the gestational age, which was in accordance with the previous reports.18) However, this increase became significant only when the number of CACs was reported in one milliliter of blood and not when the number of CACs was calculated in one million MNCs (Fig. 3). To explain this difference, one should notice that the number of EPCs in MNCs can be calculated as the number of EPCs per milliliter of blood divided by the number of MNCs per milliliter of blood. As changes occur in the number of EPCs in a manner independent of the number of MNCs, the final result of this calculation would be different from the calculation of the number of EPCs in one milliliter of blood.
Another important hint is that the way in which changes occur in the number of these cells during pathologic conditions may be more important than their absolute numbers because the changes in the number of these cells are a more realistic representation of their association with changes in the endothelial and vascular status compared to a cross-sectional enumeration. To address this issue, we used normal pregnancy as the model. Changes in the number of EPCs in different trimester peripheral blood samples have been shown using various methodologies,16) and this gave us an opportunity for obtaining two samples from one person with expected changes in the number of EPCs using a prospective approach, and offered the possibility of comparing the changes in the number of EPCs instead of the absolute values. However, limiting the study model to pregnancy also has some limitations. For example, we do not know the effects of estrogen or progesterone-induced microenvironment during pregnancy on EPCs. Future studies in young non-pregnant females would help in extrapolating these effect on EPCs.
Furthermore, the significance of changes in the number of EPCs is also influenced by the baseline number of these cells. Indeed, a small change in the number of cells can be important when the basal frequency is low, but the same change may be unimportant when higher quantities of cells are present initially. To address this problem, we analyzed a new parameter, the "relative change" in the number of cells. Interestingly, when we re-performed the correlation studies using this new parameter, correlations became more significant, indicating again that relying only on the absolute number of EPCs may be misleading, and when changes compared to the baseline are analyzed, a more accurate estimation of EPC status can be achieved. For example, the absolute changes in the number of CFU-ECs when calculated in a milliliter of whole blood and in 106 MNCs showed only a modest correlation (coefficient=0.683, p=0.042), but when the relative changes were reassessed, this correlation became very strong (coefficient=0.987, p<0.001).
Although there are only two systematic comparisons of EPCs in the literature,14)15) in several other studies, there are reports of discrepancies between EPCs as identified by different techniques.13)20)21)22) Unfortunately, none of these studies included the ECFCs or CAC precursors in their investigation and none of them enumerated the CACs through their complete platform of antigen markers as CD34+CD133+CD309+CD45+ cells. Instead most of the studies used two marker combinations such as CD34+CD133+ or CD34+CD309+. In our study, the number of CACs (enumerated as CD34+CD133+CD309+CD45+ cells) was almost invariably similar to the number of CD34+ CD133+CD309+ cells with a very strong correlation (coefficient=1, p<0.001), indicating that all these cells co-express CD45 and the two populations are the same. The number of CACs was also moderately correlated with the number of CD34+CD309+ cells and very strongly correlated with the number of CD133+CD309+ cells, but it was not correlated with the number of CD34+CD133+ cells. The same findings were previously demonstrated by George et al.15) They also showed that only the number of CD34+CD309+ cells and CACs was correlated with VEGF serum levels, and not the number of CD34+ CD133+ cells. Povsic et al.13) observed an association between the number of CD133+ cells and the number of CD34+ cells; however, they found no correlation between the number of either of these two cell groups and the number of CD309+ cells.13) We obtained similar findings, and we found a strong correlation between the number of CD34+ cells and the number of CD133+ cells, both of which failed to correlate with the CD309 marker. The number of CD309+ cells also failed to correlate with the number of CD133+CD34+ cells. These findings are consistent with the known coexpression of CD34 and CD133 as the markers for hematopoietic progenitor/stem cells other than EPCs. In fact, considering our data, any study that assesses EPCs with a marker combination that lacks CD309 is inaccurate and it has mostly evaluated hematopoietic progenitor/stem cells instead of EPCs. Therefore, the use of a three marker combination for assessment of CACs, instead of the four marker combination, is very logical and if a two marker combination is to be used, it would be better to use the CD133+CD309+ combination and not the CD34+CD133+ combination; which unfortunately, was previously used frequently.
Data regarding the correlations between changes in the number of CFU-ECs are slightly more common. George et al.15) found no correlations between the number of CFU-ECs and the number of CD34+CD309+ cells. In another study, Heiss et al.20) obtained the same findings and they did not find any correlation between the number of CD34+CD309+ cells or CD133+CD309+ cells and the number of CFU-ECs. Powell et al.21) noted that there was no correlation between the number of CD133+CD309+ cells and the number of CFU-ECs. Moreover, Povsic et al.13) in their study demonstrated a correlation between the number of CFU-ECs and the number of both CD309+ and CD34+CD309+ cells, but not with the number of CD133+, CD34+, or CD133+CD34+ cells. They also showed that the number of CFU-ECs did not correlate with the number of CD34+CD309+ or CD34+CD133+CD309+ cells, but it was negatively correlated with the number of CD34+CD133+ cells. Tura et al.14) could not find any correlation between the number of subpopulations expressing CD34 or CD133 or CD309, singly or in any combination, and the number of CFU-ECs. They only observed a trend for correlation between the number of CFU-ECs and the number of CD14+ cells. Similarly, we could not identify any correlation between the number of CFU-ECs and the number of any other measured target cells except for a moderate correlation with the number of CD34+ cells.
Although the culturing techniques are the standard methods used for enumerating ECFCs, we passed up this modality because of the following two reasons: first, their frequency in the peripheral blood is very low and large volume blood samples are needed to obtain accurate measurements; also, most of our participants refused to donate such large volumes of blood during their pregnancy period. In addition, a strong correlation between the number of culture-derived ECFC colonies and the number of flow cytometrically enumerated CD34+CD45- and CD34+CD309+ cells has been demonstrated without any correlation with the CD34+CD133+ as the presumed marker of CACs.22)23) Consequently, in our study, we used a flow cytometric technique to enumerate the likely ECFCs. We could not identify a significant correlation between changes in the number of ECFCs and the number of CFU-ECs, but there were moderate correlations with the number of CACs, CD34+CD45-, and CD309+CD45- cells. In fact, none of the correlations with the number of ECFCs were strong enough so that we cannot recommend other marker combinations instead. Changes in the number of CAC precursors were correlated with changes in the number of CACs, indicating that changes in the number of CACs can be due to both the differentiation and mobilization processes.
It can be concluded that the current three populations representing EPC subsets are different cells with independent behaviors. In addition, parameters such as the type of reporting the data and the baseline frequency of cells can affect the final results. There is a real need for a universal definition of EPCs and also for standardization of the assays used for their measurement. We can suggest that relative changes in the number of measured cells are a more convenient parameter that reflects the EPC status in each individual. We can also suggest that the CD133+CD309+ combination can be used as a substitute for appropriate measurement of CACs instead of the CD34+CD133+CD309+CD45+ combination.
Figures and Tables
Fig. 1
Typical density plot diagrams defining some of the variable regions used to enumerate absolute counts of the circulating angiogenic cells marked as CD34+CD133+CD309+CD45+ cells.

Fig. 3
Box plot defining the median and range of the number of CACs in the 1st and 3rd trimester peripheral blood. Number of CACs increases as the gestational age advances, but it only reaches significance when it is reported as the number of cells in a milliliter of blood. This shows that the way of reporting the data affects the final results. CAC: circulating angiogenic cell.
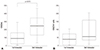
Table 1
Marker combinations used for flow cytometric measurement of different EPC subsets

Marker combination | Used as the gold standard for | Used as an alternative for |
---|---|---|
CD34+ | - | CAC and ECFC |
CD133+ | - | CAC precursors and CAC |
CD309+ | - | CAC precursors, CAC, and ECFC |
CD34+CD133+ | - | CAC8) |
CD34+CD309+ | - | CAC9) |
CD133+CD45- | - | CAC10) |
CD133+CD309+ | - | CAC11) |
CD34+CD45- | - | ECFC22) |
CD309+CD45- | - | ECFC23) |
CD34+CD133+CD309+ | - | CAC6) |
CD34+CD133-CD309+ | - | ECFC12) |
CD34-CD133+CD309+ | CAC precursors19) | |
CD34+CD133+CD309+CD45+ | CAC12) | |
CD34+CD133-CD309+CD45- | ECFC12) |
Table 2
Clinical profiles of women having a normal pregnancy

Table 3
Summary of correlations between absolute changes in the number of EPC subsets

Table 4
Summary of correlations between relative changes in the number of EPC subsets

Acknowledgments
This investigation is the result of a thesis supported by the research grant #93-7682 from the Vice Chancellor of research at Shiraz University of Medical Sciences. The authors would like to thank Dr. Nasrin Shokrpour working at the Center for Development of Clinical Research of Nemazee Hospital for the editorial assistance.
References
1. Attar A, Khosravi Maharlooi M, Khoshkhou S, et al. Colony forming unit endothelial cells do not exhibit telomerase alternative splicing variants and activity. Iran Biomed J. 2013; 17:146–151.
2. Asahara T, Murohara T, Sullivan A, et al. Isolation of putative progenitor endothelial cells for angiogenesis. Science. 1997; 275:964–967.
3. Hill JM, Zalos G, Halcox JP, et al. Circulating endothelial progenitor cells, vascular function, and cardiovascular risk. N Engl J Med. 2003; 348:593–600.
4. Lin C, Rajakumar A, Plymire DA, Verma V, Markovic N, Hubel CA. Maternal endothelial progenitor colony-forming units with macrophage characteristics are reduced in preeclampsia. Am J Hypertens. 2009; 22:1014–1019.
5. Peichev M, Naiyer AJ, Pereira D, et al. Expression of VEGFR-2 and AC133 by circulating human CD34(+) cells identifies a population of functional endothelial precursors. Blood. 2000; 95:952–958.
6. Werner N, Kosiol S, Schiegl T, et al. Circulating endothelial progenitor cells and cardiovascular outcomes. N Engl J Med. 2005; 353:999–1007.
7. Timmermans F, Plum J, Yöder MC, Ingram DA, Vandekerckhove B, Case J. Endothelial progenitor cells: identity defined? J Cell Mol Med. 2009; 13:87–102.
8. Güven H, Shepherd RM, Bach RG, Capoccia BJ, Link DC. The number of endothelial progenitor cell colonies in the blood is increased in patients with angiographically significant coronary artery disease. J Am Coll Cardiol. 2006; 48:1579–1587.
9. Allanore Y, Batteux F, Avouac J, Assous N, Weill B, Kahan A. Levels of circulating endothelial progenitor cells in systemic sclerosis. Clin Exp Rheumatol. 2007; 25:60–66.
10. Schömig K, Busch G, Steppich B, et al. Interleukin-8 is associated with circulating CD133+ progenitor cells in acute myocardial infarction. Eur Heart J. 2006; 27:1032–1037.
11. Hirschi KK, Ingram DA, Yoder MC. Assessing identity, phenotype, and fate of endothelial progenitor cells. Arterioscler Thromb Vasc Biol. 2008; 28:1584–1595.
12. Ingram DA, Mead LE, Tanaka H, et al. Identifi cation of a novel hierarchy of endothelial progenitor cells using human peripheral and umbilical cord blood. Blood. 2004; 104:2752–2760.
13. Povsic TJ, Zavodni KL, Vainorius E, Kherani JF, Goldschmidt-Clermont PJ, Peterson ED. Common endothelial progenitor cell assays identify discrete endothelial progenitor cell populations. Am Heart J. 2009; 157:335–344.
14. Tura O, Barclay GR, Roddie H, Davies J, Turner ML. Absence of a relationship between immunophenotypic and colony enumeration analysis of endothelial progenitor cells in clinical haematopoietic cell sources. J Transl Med. 2007; 5:37.
15. George J, Shmilovich H, Deutsch V, Miller H, Keren G, Roth A. Comparative analysis of methods for assessment of circulating endothelial progenitor cells. Tissue Eng. 2006; 12:331–335.
16. Parsanezhad ME, Attar A, Namavar-Jahromi B, et al. Changes in endothelial progenitor cell subsets in normal pregnancy compared with preeclampsia. J Chin Med Assoc. 2015; 78:345–352.
17. Khan SS, Solomon MA, McCoy JP Jr. Detection of circulating endothelial cells and endothelial progenitor cells by flow cytometry. Cytometry B Clin Cytom. 2005; 64:1–8.
18. Buemi M, Allegra A, D'Anna R, et al. Concentration of circulating endothelial progenitor cells (EPC) in normal pregnancy and in pregnant women with diabetes and hypertension. Am J Obstet Gynecol. 2007; 196:68.e1–68.e6.
19. Friedrich EB, Walenta K, Scharlau J, Nickenig G, Werner N. CD34-/CD133+/VEGFR-2+ endothelial progenitor cell subpopulation with potent vasoregenerative capacities. Circ Res. 2006; 98:e20–e25.
20. Heiss C, Keymel S, Niesler U, Ziemann J, Kelm M, Kalka C. Impaired progenitor cell activity in age-related endothelial dysfunction. J Am Coll Cardiol. 2005; 45:1441–1448.
21. Powell TM, Paul JD, Hill JM, et al. Granulocyte colony-stimulating factor mobilizes functional endothelial progenitor cells in patients with coronary artery disease. Arterioscler Thromb Vasc Biol. 2005; 25:296–301.
22. Massa M, Campanelli R, Bonetti E, Ferrario M, Marinoni B, Rosti V. Rapid and large increase of the frequency of circulating endothelial colony-forming cells (ECFCs) generating late outgrowth endothelial cells in patients with acute myocardial infarction. Exp Hematol. 2009; 37:8–9.
23. Davani S, Gozalo C, Gambert S, et al. The polymorphism Trp719Arg in the kinesin-like protein 6 is associated with the presence of late outgrowth endothelial progenitor cells in acute myocardial infarction. Atherosclerosis. 2010; 210:48–50.
Supplementary Materials
The online-only Data Supplement is available with this article at http://dx.doi.org/10.4070/kcj.2015.45.4.325.