Abstract
Background and Objectives
Subjects and Methods
Results
Figures and Tables
Fig. 1
Comparison of hMVRI in patients with and without MACEs. hMVRI: hyperemic microvascular resistance index, MACE: major adverse cardiovascular event.
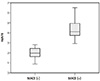
Fig. 2
Receiver-operating characteristic curve showing the optimal cut-off value of hMVRI for predicting long-term MACEs. BCV: best cut-off value, hMVRI: hyperemic microvascular resistance index.
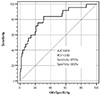
Fig. 3
The Kaplan-Meier curves using the cut-off value of hMVRI for MACEs. hMVRI: hyperemic microvascular resistance index, MACE: major adverse cardiovascular event.
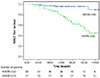
Table 1
Clinical characteristics of patients with and without cardiovascular events
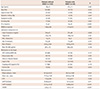
MACE: major adverse cardiovascular event, EF: ejection fraction, WMSI: wall motion score index, HDL: high density lipoprotein, LDL: low density lipoprotein, CK: creatine kinase, CK-MB: creatine kinase-myocardial band, ACE: angiotensin converting enzyme, ARB: angiotensin II receptor blocker, CFR: coronary flow velocity reserve, bAPV: baseline average peak velocity, hAPV: hyperemic average peak velocity, hMVRI: hyperemic microvascular resistance index
Table 2
Angiographic and procedural characteristics of patients with and without cardiovascular events
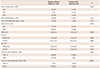
MACE: major adverse cardiovascular event, LAD: left anterior descending artery, LCX: left circumflex artery, RCA: right coronary artery, DES: drug-eluting artery, BMS: bare metal stent, RVD: reference vessel diameter, PCI: percutaneous coronary intervention, MLD: minimal lumen diameter, DS: diameter stenosis, TIMI: thrombolysis in myocardial infarction
Table 3
The Cox hazards analysis for predictors of cardiovascular events
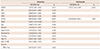
HR: hazard ratio, CI: confidence interval, hMVRI: hyperemic microvascular resistance index, CFR: coronary flow velocity reserve, bAPV: baseline average peak velocity, hAPV: hyperemic average peak velocity, EF: ejection fraction, WMSI: wall motion score index, CK: creatine kinase, CK-MB: creatine kinase-myocardial band, PCI: percutaneous coronary intervention, TIMI: thrombolysis in myocardial infarction
Table 4
Clinical, angiographic, and procedural characteristics
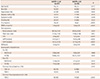
hMVRI: hyperemic microvascular resistance index, EF: ejection fraction, WMSI: wall motion score index, CFR: coronary flow velocity reserve, bAPV: baseline average peak velocity, hAPV: hyperemic average peak velocity, RVD: reference vessel diameter, PCI: percutaneous coronary intervention, MLD: minimal lumen diameter, DS: diameter stenosis, TIMI: thrombolysis in myocardial infarction