Abstract
Sinoatrial node (SAN) automaticity is jointly regulated by a voltage (cyclic activation and deactivation of membrane ion channels) and Ca2+ clocks (rhythmic spontaneous sarcoplasmic reticulum Ca2+ release). Using optical mapping in Langendorff-perfused canine right atrium, we previously demonstrated that the β-adrenergic stimulation pushes the leading pacemaker to the superior SAN, which has the fastest activation rate and the most robust late diastolic intracellular calcium (Cai) elevation. Dysfunction of the superior SAN is commonly observed in animal models of heart failure and atrial fibrillation (AF), which are known to be associated with abnormal SAN automaticity. Using the 3D electroanatomic mapping techniques, we demonstrated that superior SAN served as the earliest atrial activation site (EAS) during sympathetic stimulation in healthy humans. In contrast, unresponsiveness of superior SAN to sympathetic stimulation was a characteristic finding in patients with AF and SAN dysfunction, and the 3D electroanatomic mapping technique had better diagnostic sensitivity than corrected SAN recovery time testing. However, both tests have significant limitations in detecting patients with symptomatic sick sinus syndrome. Recently, we reported that the location of the EAS can be predicted by the amplitudes of P-wave in the inferior leads. The inferior P-wave amplitudes can also be used to assess the superior SAN responsiveness to sympathetic stimulation. Inverted or isoelectric P-waves at baseline that fail to normalize during isoproterenol infusion suggest SAN dysfunction. P-wave morphology analyses may be helpful in determining the SAN function in patients at risk of symptomatic sick sinus syndrome.
The sinoatrial node (SAN) automaticity is essential for maintaining normal cardiac function. Sick sinus syndrome is an abnormality involving the generation of the action potential by the SAN and is characterized by an atrial rate inappropriate for physiological requirements. The sick sinus syndrome occurs in 1 of every 600 cardiac patients older than 65 years and accounts for approximately half of implantations of pacemakers in the United States.1) A better understanding of the mechanisms of SAN automaticity and sick sinus syndrome is therefore clinically important. The SAN automaticity is maintained by synergistic actions of a "voltage clock" mediated by voltage-sensitive membrane ionic currents such as the hyperpolarization-activated pacemaker current (If)2) and a "Ca2+ clock" mediated by rhythmic spontaneous sarcoplasmic reticulum Ca2+ release.3) While extensive work has been performed to document the interactions of voltage and Ca2+ clocks of the SAN in animal models, translating these findings to human patient care is difficult. Our review will focus on the translational studies of human SAN function.
Cardiac automaticity at the organ level is very complex. In addition to cellular mechanisms, integrative anatomical and physiological factors are involved in cardiac pacemaking. The intact SAN is a heterogeneous structure that includes multiple different cell types interacting with each other.4)5)6) The Ca2+ clock in the superior SAN is primarily responsible for rate acceleration during sympathetic stimulation.7)8)9)10)11) However, the relative importance of the voltage and Ca2+ clocks for pacemaking in different regions of the SAN, and in response to neurohumeral stimuli such as β-agonists, may be different. Indeed, activation maps in intact canine right atrium (RA) have shown that the SAN impulse origin is multicentric,12 and sympathetic stimulation predictably results in a cranial (superior) shift of the pacemaking site in human and dogs.13)14) Based on evidence from isolated SAN myocytes, late diastolic Cai elevation relative to the action potential upstroke is a key signature of pacemaking by the Ca2+ clock.15)16)17)18)19)20) The same phenomenon could provide insights into the relative importance of the Ca2+ and voltage clock mechanisms in pacemaking in intact RA tissue,7) subsidiary pacemakers21)22) or diseased state.23)24)
Schuessler et al.14) reported in a canine model that sympathetic stimulation in general tends to induce a cranial shift in the location of the pacemaker within the pacemaker complex. With the development of 3 dimensional (3D) endocardial electroanatomical mapping techniques, it is possible to define the activation patterns within the human atria to accurately locate the earliest atrial activation site (EAS).25) If the findings in canine models are applicable to humans, then the superior SAN should vigorously respond to sympathetic stimulation and serve as the dominant pacemaker during sympathetic stimulation. Indeed, EAS of control human subjects shifted cranially during isoproterenol infusion (Fig. 1A).26) These findings indicate that in both canine models and humans, the superior SAN is primarily responsible for heart rate acceleration during sympathetic stimulation in patients with normal SAN function.
Atrial fibrillation (AF) is associated with significant electrophysiological and structural remodeling of the atria, and is often associated with sick sinus syndrome.27)28) The SAN dysfunction may be reversible after successful catheter ablation of AF.29) In dogs, persistent (>2 weeks) rapid atrial pacing and chronic AF resulted in SAN dysfunction, as evidenced by prolongation of the SAN recovery time and decreases in the intrinsic heart rates.30) Unresponsiveness of the Ca2+ clock in the superior SAN to sympathetic stimulation is a characteristic finding in dogs with AF and heart failure.31)32) Consistent with that found in a canine model,31) the patient with AF with SAN dysfunction had impaired heart rate acceleration and absence of upward shift of the EAS during isoproterenol stimulation (Fig. 1B). These findings suggest that Ca2+ clock malfunction underlies these abnormal physiological responses to isoproterenol infusion.
Previous studies have shown that the impulse from the SAN propagates into RA through an upper and a lower exit site.33) It is possible that isoproterenol preferentially shifts the exit site to the upper portion of the SAN instead of the activation of the superior SAN by the activation of Ca2+ clock. Because the extracellular electrograms cannot be used to differentiate SAN activation and RA activation, the shifting of SAN exit sites and the shifting of the actual pacemaking sites may look the same on the 3D map. The data from our human 3D mapping study cannot be used to differentiate these two mechanisms.26) However, we found that isoproterenol infusion reactivates the pacemaker in the superior SAN in several patients with extensive RA fibrosis and junctional rhythm at baseline (Fig. 2). In these patients, changing the sinoatrial node-right atrium exit pathway is clearly not a mechanism of upward shift of the EAS. Moreover, in other patients with upward shifting of EAS during isoproterenol infusion, the conduction between SAN to RA occurred at multiple directions, without evidence of conduction delay along the crista terminalis or the septum. Based on these findings and the mapping of the Ca2+ clock activity in the canine models, we propose that the pacemaker hierarchy is likely to be created by differential responses of Ca2+ clock to sympathetic tone at different portions of the SAN rather than changing the exit from a fixed pacemaking site.
As the elderly population continues to expand, AF is becoming an increasingly common medical condition.34)35)36)37) Amiodarone is the most frequently used agent for maintaining sinus rhythm in patients with AF. Amiodarone impairs SAN function in one-third of patients and is associated with an increased risk of pacemaker insertion.38) Our study also showed that amiodarone induced SAN dysfunction in one-fourth of patients without SAN dysfunction at baseline.39) Amiodarone caused impaired heart rate acceleration and impaired cranial shift of EAS after sympathetic stimulation (Fig. 3).39) Therefore, Ca2+ clock malfunction of the superior SAN might be related with impaired heart rate acceleration and cranial shift of the pacemaking site. Amiodarone inhibits multiple ion channels (Ito, IKr, IKs, ICa, INa) and is a beta adrenergic blocker.40) Because amiodarone is a potent blocker of Ica,40) it may suppress the Ca2+ clock in the SAN.41) In addition, amiodarone can inhibit the sympathetic activity. A downward shift of the EAS has been reported in human patients after esmolol infusion.25) Therefore, the beta blocking effects of amiodarone may also influence the location of the EAS. Amiodarone may induce hypothyroidism, and this effect may, in part, decrease SAN function.38) Finally, Turker et al.42) recently showed that amiodarone inhibits the small-conductance calcium-activated K (SK) channel. Because the SK channel is important in SAN and atrioventricular node function,43)44) SK channel inhibition may affect SAN automaticity.
Sanders et al.45) previously reported that multicentric activation is found both in normal control and heart failure. However, the EASs are more numerous in normal control (average 4 sites) than in heart failure (average 2.5 sites). They also reported that the SAN complex in patients with SAN dysfunction is more often unicentric, localized to the lower crista terminalis at the site of the largest residual voltage amplitude.45) We also found that most healthy control patients had multicentric SAN activation pattern (Fig. 1A), and that the number of EAS in AF patients is smaller than that in healthy controls (Fig. 1B). A reduction of the EAS suggests that there are fewer backup pacemaking sites to respond to sympathetic stimulation, which could be a sign of SAN dysfunction.
The P-wave morphology has been used to identify the origins of focal atrial tachycardias with a high sensitivity and specificity.46)47)
Fig. 4 shows that compared with sinus rhythm (arrows), atrial tachycardia originating from the inferior part of crista terminalis had smaller amplitude of P-wave in leads II, III, and aVF (broken arrows). We found that the distances from the superior vena cava-right atrial junction to the EAS were negatively correlated with the amplitude of P-wave in inferior leads. If the EAS was shifted to the superior part of crista terminalis or superior vena cava, the P-wave amplitudes were increased in most of the patients without SAN dysfunction.39) In contrast, the shift of the EAS to the inferior part of crista terminalis or inferior vena cava was related with decreased P-wave amplitude in inferior leads in patients with SAN dysfunction (Fig. 5A). These findings suggest that the change of P-wave morphology in inferior leads during isoproterenol infusion can be used to assess the superior SAN responsiveness to isoproterenol infusion. Patients with SAN dysfunction showed low amplitude of P-waves at baseline and during sympathetic stimulation (Fig. 5B).
We analyzed the comparative efficacy of corrected sinoatrial node recovery time (CSNRT) and 3D mapping in differentiating AF patients with and without sinus bradycardia. CSNRT>550 ms is considered a positive CSNRT test. The failure of superior SAN to serve as EAS during isoproterenol infusion is considered a positive 3D mapping test (Fig. 6). The sensitivity, specificity, positive predicative efficacy, and negative predicative efficacy of the CSNRT test are 35%, 89%, 45% and 84%, respectively (Fig. 6A), and of the 3D mapping test are 78%, 78%, 47% and 93%, respectively (Fig. 6B). The 3D mapping test was twice as sensitive but slightly less specific than the CSNRT test in detecting AF patients with sinus bradycardia. However, both tests have limitations as they are invasive and time consuming tests.
Recently, we analyzed the poor increase in inferior P-wave amplitude during sympathetic stimulation for the diagnosis of sick sinus syndrome. The poor increases of P-wave amplitude of lead aVF (<0.1 mV) during isoproterenol infusion showed a sensitivity of 78% and specificity of 89% for the diagnosis of sick sinus syndrome. Finally, the combined algorithm using CSNRT of >550 ms and poor increases of P-wave amplitude of lead aVF showed further improved diagnostic accuracy (sensitivity of 89% and specificity of 76%).
Calmodulin-dependent protein kinase II (CaMKII) has emerged as a central regulator of physiological SAN responses and a key determinant of SAN dysfunction.48) The calcium and CaMKII is especially important for physiological "fight or flight" SAN beating rate responses.49) Inhibition of CaMKII in SAN does not affect baseline heart rate, but reduces heart rate increases in response to physiological stress. Excessive CaMKII activity, as occurs under pathological conditions such as heart failure, ischemia, and diabetes, can promote intracellular Ca2+ overload and reactive oxygen species production. Nicotinamide adenine dinucleotide phosphate oxidase is activated in conditions with increased angiotensin II, leading to oxidation of two methionine residues of CaMKII, rendering the enzyme autonomously active. Increased CaMKII activation leads to SAN cell death, reducing the threshold volume of automatic cells of the SAN and increasing non-excitable tissue in the form of fibrosis. CaMKII-induced apoptosis results in SAN cell loss, which disrupts the normal source-sink balance leading to sinoatrial node dysfunction.50)51) In a canine heart failure model, increased expression of adenosine receptors within the SAN region together with increased fibrosis have been reported to be related with the loss of synchrony and sinoatrial node dysfunction.52) A source-sink mismatch caused by cell loss has been observed in a mouse model of diabetes and in ankyrin-B syndrome.53)54)
The voltage and Ca2+ clocks jointly regulate SAN automaticity. In various models of sick sinus syndrome, the dysfunction of superior SAN during sympathetic stimulation was consistently observed. In human 3D mapping, superior SAN was identified as the leading pacemaker site during sympathetic stimulation. However, unresponsiveness of superior SAN to sympathetic stimulation was commonly observed in patients with sinus dysfunction. 3D mapping, which showed unresponsiveness of the superior SAN or inferior P-wave amplitude, was more sensitive than classic CSNRT in identifying patients with sinus dysfunction. These novel tools obtained from basic research might help to diagnose sick sinus syndrome
This study was supported in part by research grants from the Basic Science Research Program through the National Research Foundation of Korea funded by the Korean Heart Rhythm Society (2011-3), the Ministry of Education, Science and Technology (NRF-2010-0021993, NRF-2012R1A2A2A02045367), a grant of the Korean Healthcare technology R&D project funded by Ministry of Health & Welfare (HI12C1552), United States National Institutions of Health grants R01 HL71140 and P01 HL78931, the Indiana University Health/Indiana University School of Medicine Strategic Research Initiative and a Medtronic-Zipes Endowment of the Indiana University.
Acknowledgements
Medtronic, St. Jude, and Cyberonics donated equipment to Dr. Chen's research laboratory.
References
1. Adán V, Crown LA. Diagnosis and treatment of sick sinus syndrome. Am Fam Physician. 2003; 67:1725–1732. PMID: 12725451.
2. DiFrancesco D. The role of the funny current in pacemaker activity. Circ Res. 2010; 106:434–446. PMID: 20167941.
3. Lakatta EG, Maltsev VA, Vinogradova TM. A coupled SYSTEM of intracellular Ca2+ clocks and surface membrane voltage clocks controls the timekeeping mechanism of the heart's pacemaker. Circ Res. 2010; 106:659–673. PMID: 20203315.
4. Verheijck EE, van Kempen MJ, Veereschild M, Lurvink J, Jongsma HJ, Bouman LN. Electrophysiological features of the mouse sinoatrial node in relation to connexin distribution. Cardiovasc Res. 2001; 52:40–50. PMID: 11557232.
5. Lancaster MK, Jones SA, Harrison SM, Boyett MR. Intracellular Ca2+ and pacemaking within the rabbit sinoatrial node: heterogeneity of role and control. J Physiol. 2004; 556:481–494. PMID: 14724216.
6. Tellez JO, Dobrzynski H, Greener ID, et al. Differential expression of ion channel transcripts in atrial muscle and sinoatrial node in rabbit. Circ Res. 2006; 99:1384–1393. PMID: 17082478.
7. Joung B, Tang L, Maruyama M, et al. Intracellular calcium dynamics and acceleration of sinus rhythm by beta-adrenergic stimulation. Circulation. 2009; 119:788–796. PMID: 19188501.
8. Joung B, Chen PS, Lin SF. The role of the calcium and the voltage clocks in sinoatrial node dysfunction. Yonsei Med J. 2011; 52:211–219. PMID: 21319337.
9. Chen PS, Joung B, Shinohara T, Das M, Chen Z, Lin SF. The initiation of the heart beat. Circ J. 2010; 74:221–225. PMID: 20019407.
10. Joung B, Ogawa M, Lin SF, Chen PS. The calcium and voltage clocks in sinoatrial node automaticity. Korean Circ J. 2009; 39:217–222. PMID: 19949626.
11. Efimov IR, Fedorov VV, Joung B, Lin SF. Mapping cardiac pacemaker circuits: methodological puzzles of the sinoatrial node optical mapping. Circ Res. 2010; 106:255–271. PMID: 20133911.
12. Boineau JP, Miller CB, Schuessler RB, et al. Activation sequence and potential distribution maps demonstrating multicentric atrial impulse origin in dogs. Circ Res. 1984; 54:332–347. PMID: 6697451.
13. Boineau JP, Schuessler RB, Mooney CR, et al. Multicentric origin of the atrial depolarization wave: the pacemaker complex. Relation to dynamics of atrial conduction, P-wave changes and heart rate control. Circulation. 1978; 58:1036–1048. PMID: 709760.
14. Schuessler RB, Boineau JP, Wylds AC, Hill DA, Miller CB, Roeske WR. Effect of canine cardiac nerves on heart rate, rhythm, and pacemaker location. Am J Physiol. 1986; 250:H630–H644. PMID: 3963219.
15. Li J, Qu J, Nathan RD. Ionic basis of ryanodine's negative chronotropic effect on pacemaker cells isolated from the sinoatrial node. Am J Physiol. 1997; 273:H2481–H2489. PMID: 9374788.
16. Ju YK, Allen DG. Intracellular calcium and Na+-Ca2+ exchange current in isolated toad pacemaker cells. J Physiol. 1998; 508:153–166. PMID: 9490832.
17. Hüser J, Blatter LA, Lipsius SL. Intracellular Ca2+ release contributes to automaticity in cat atrial pacemaker cells. J Physiol. 2000; 524(Pt 2):415–422. PMID: 10766922.
18. Vinogradova TM, Bogdanov KY, Lakatta EG. Beta-adrenergic stimulation modulates ryanodine receptor Ca(2+) release during diastolic depolarization to accelerate pacemaker activity in rabbit sinoatrial nodal cells. Circ Res. 2002; 90:73–79. PMID: 11786521.
19. Maltsev VA, Vinogradova TM, Lakatta EG. The emergence of a general theory of the initiation and strength of the heartbeat. J Pharmacol Sci. 2006; 100:338–369. PMID: 16799255.
20. Vinogradova TM, Lyashkov AE, Zhu W, et al. High basal protein kinase A-dependent phosphorylation drives rhythmic internal Ca2+ store oscillations and spontaneous beating of cardiac pacemaker cells. Circ Res. 2006; 98:505–514. PMID: 16424365.
21. Park S, Park H, Hwang HJ, et al. Heart rate acceleration of a subsidiary pacemaker by β-adrenergic stimulation. Korean Circ J. 2011; 41:658–665. PMID: 22194761.
22. Kim D, Shinohara T, Joung B, et al. Calcium dynamics and the mechanisms of atrioventricular junctional rhythm. J Am Coll Cardiol. 2010; 56:805–812. PMID: 20797495.
23. Joung B, Zhang H, Shinohara T, et al. Delayed afterdepolarization in intact canine sinoatrial node as a novel mechanism for atrial arrhythmia. J Cardiovasc Electrophysiol. 2011; 22:448–454. PMID: 21040091.
24. Joung B, Shinohara T, Zhang H, et al. Tachybradycardia in the isolated canine right atrium induced by chronic sympathetic stimulation and pacemaker current inhibition. Am J Physiol Heart Circ Physiol. 2010; 299:H634–H642. PMID: 20601460.
25. Marrouche NF, Beheiry S, Tomassoni G, et al. Three-dimensional nonfluoroscopic mapping and ablation of inappropriate sinus tachycardia. Procedural strategies and long-term outcome. J Am Coll Cardiol. 2002; 39:1046–1054. PMID: 11897449.
26. Joung B, Hwang HJ, Pak HN, et al. Abnormal response of superior sinoatrial node to sympathetic stimulation is a characteristic finding in patients with atrial fibrillation and symptomatic bradycardia. Circ Arrhythm Electrophysiol. 2011; 4:799–807. PMID: 22007035.
27. Gomes JA, Kang PS, Matheson M, Gough WB Jr, El-Sherif N. Coexistence of sick sinus rhythm and atrial flutter-fibrillation. Circulation. 1981; 63:80–86. PMID: 7438410.
28. van den Berg MP, van Gelder IC. Atrial fibrillation and sinus node dysfunction. J Am Coll Cardiol. 2001; 38:1585–1586. PMID: 11691547.
29. Hocini M, Sanders P, Deisenhofer I, et al. Reverse remodeling of sinus node function after catheter ablation of atrial fibrillation in patients with prolonged sinus pauses. Circulation. 2003; 108:1172–1175. PMID: 12952840.
30. Elvan A, Wylie K, Zipes DP. Pacing-induced chronic atrial fibrillation impairs sinus node function in dogs. Electrophysiological remodeling. Circulation. 1996; 94:2953–2960. PMID: 8941126.
31. Joung B, Lin SF, Chen Z, et al. Mechanisms of sinoatrial node dysfunction in a canine model of pacing-induced atrial fibrillation. Heart Rhythm. 2010; 7:88–95. PMID: 19914141.
32. Shinohara T, Park HW, Han S, et al. Ca2+ clock malfunction in a canine model of pacing-induced heart failure. Am J Physiol Heart Circ Physiol. 2010; 299:H1805–H1811. PMID: 20889842.
33. Fedorov VV, Schuessler RB, Hemphill M, et al. Structural and functional evidence for discrete exit pathways that connect the canine sinoatrial node and atria. Circ Res. 2009; 104:915–923. PMID: 19246679.
34. Aronow WS. Management of the older person with atrial fibrillation. J Am Geriatr Soc. 1999; 47:740–748. PMID: 10366178.
35. Chugh SS, Blackshear JL, Shen WK, Hammill SC, Gersh BJ. Epidemiology and natural history of atrial fibrillation: clinical implications. J Am Coll Cardiol. 2001; 37:371–378. PMID: 11216949.
36. Go AS, Hylek EM, Phillips KA, et al. Prevalence of diagnosed atrial fibrillation in adults: national implications for rhythm management and stroke prevention: the AnTicoagulation and Risk Factors in Atrial Fibrillation (ATRIA) Study. JAMA. 2001; 285:2370–2375. PMID: 11343485.
37. Moon J, Hong YJ, Shim J, et al. Right atrial anatomical remodeling affects early outcomes of nonvalvular atrial fibrillation after radiofrequency ablation. Circ J. 2012; 76:860–867. PMID: 22293450.
38. Essebag V, Hadjis T, Platt RW, Pilote L. Amiodarone and the risk of bradyarrhythmia requiring permanent pacemaker in elderly patients with atrial fibrillation and prior myocardial infarction. J Am Coll Cardiol. 2003; 41:249–254. PMID: 12535818.
39. Mun HS, Shen C, Pak HN, et al. Chronic amiodarone therapy impairs the function of the superior sinoatrial node in patients with atrial fibrillation. Circ J. 2013; 77:2255–2263. PMID: 23739532.
40. Kodama I, Kamiya K, Toyama J. Cellular electropharmacology of amiodarone. Cardiovasc Res. 1997; 35:13–29. PMID: 9302343.
41. Maltsev VA, Lakatta EG. Dynamic interactions of an intracellular Ca2+ clock and membrane ion channel clock underlie robust initiation and regulation of cardiac pacemaker function. Cardiovasc Res. 2008; 77:274–284. PMID: 18006441.
42. Turker I, Yu CC, Chang PC, et al. Amiodarone inhibits apamin-sensitive potassium currents. PLoS One. 2013; 8:e70450. PMID: 23922993.
43. Chen WT, Chen YC, Lu YY, et al. Apamin modulates electrophysiological characteristics of the pulmonary vein and the Sinoatrial Node. Eur J Clin Invest. 2013; 43:957–963. PMID: 23834267.
44. Zhang Q, Timofeyev V, Lu L, et al. Functional roles of a Ca2+-activated K+ channel in atrioventricular nodes. Circ Res. 2008; 102:465–471. PMID: 18096820.
45. Sanders P, Kistler PM, Morton JB, Spence SJ, Kalman JM. Remodeling of sinus node function in patients with congestive heart failure: reduction in sinus node reserve. Circulation. 2004; 110:897–903. PMID: 15302799.
46. Kistler PM, Roberts-Thomson KC, Haqqani HM, et al. P-wave morphology in focal atrial tachycardia: development of an algorithm to predict the anatomic site of origin. J Am Coll Cardiol. 2006; 48:1010–1017. PMID: 16949495.
47. Uhm JS, Shim J, Wi J, et al. An electrocardiography algorithm combined with clinical features could localize the origins of focal atrial tachycardias in adjacent structures. Europace. 2014; 16:1061–1068. PMID: 24381331.
48. Wu Y, Rasmussen TP, Koval OM, et al. The mitochondrial uniporter controls fight or flight heart rate increases. Nat Commun. 2015; 6:6081. PMID: 25603276.
49. Wu Y, Gao Z, Chen B, et al. Calmodulin kinase II is required for fight or flight sinoatrial node physiology. Proc Natl Acad Sci U S A. 2009; 106:5972–5977. PMID: 19276108.
50. Swaminathan PD, Purohit A, Soni S, Voigt N, et al. Oxidized CaMKII causes cardiac sinus node dysfunction in mice. J Clin Invest. 2011; 121:3277–3288. PMID: 21785215.
51. Huke S, Knollmann BC. Oxidized CaMKII: a "heart stopper" for the sinus node? J Clin Invest. 2011; 121:2975–2977. PMID: 21785211.
52. Lou Q, Hansen BJ, Fedorenko O, et al. Upregulation of adenosine A1 receptors facilitates sinoatrial node dysfunction in chronic canine heart failure by exacerbating nodal conduction abnormalities revealed by novel dual-sided intramural optical mapping. Circulation. 2014; 130:315–324. PMID: 24838362.
53. Luo M, Guan X, Luczak ED, et al. Diabetes increases mortality after myocardial infarction by oxidizing CaMKII. J Clin Invest. 2013; 123:1262–1274. PMID: 23426181.
54. Wolf RM, Glynn P, Hashemi S, et al. Atrial fibrillation and sinus node dysfunction in human ankyrin-B syndrome: a computational analysis. Am J Physiol Heart Circ Physiol. 2013; 304:H1253–H1266. PMID: 23436330.
Fig. 1
Effects of isoproterenol infusion on EAS. A: cranial shift of the EAS in a healthy control patient. The EAS at baseline (a) was in the superior one-third of crista terminalis (CT). The EAS during isoproterenol infusion (b) was at the junction between the SVC and the RA. B: impaired cranial shift of the EAS in an AF patient with symptomatic bradycardia. The EAS at baseline (a) was ectopic (at the RA free wall). The EAS during isoproterenol infusion (b) was located at the mid one-third of CT. The superior SAN in this patient was inactive with or without isoproterenol. The dashed line in each panel marks the CT. EAS: earliest atrial activation site, SVC: superior vena cava, RA: right atrial, AF: atrial fibrillation, SAN: sinoatrial node. Modified with permission from Joung et al.26)
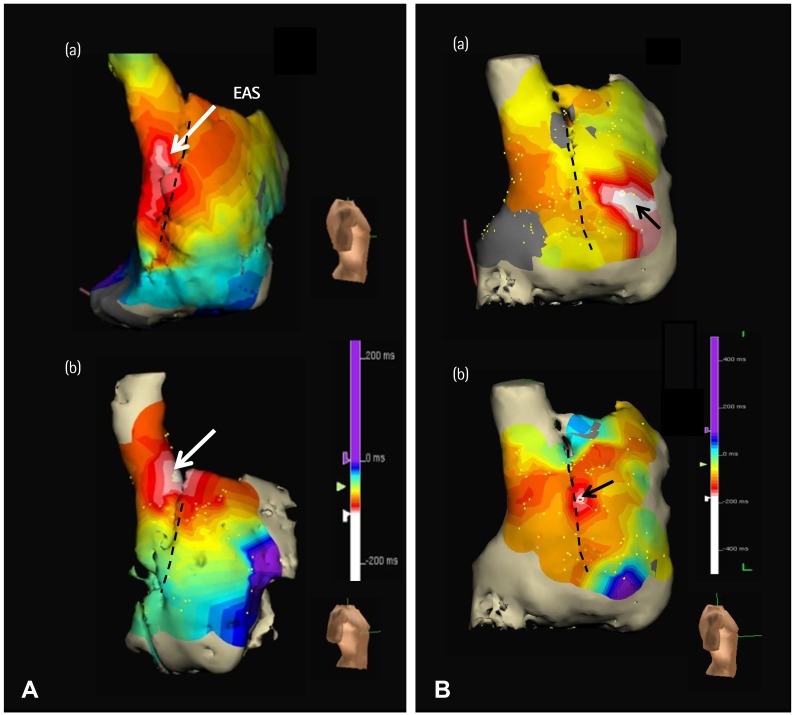
Fig. 2
The different leading pacemaker sites during heart rate acceleration. A 50-year-old women had long-standing persistent AF and SAN dysfunction. A: (a) Junctional rhythm of 40 bpm after termination of AF. (b) Sinus P-wave appeared and sinus rate increased to 110 bpm during isoproterenol infusion of 10 µg/min. B: (a) RA activation map. The superior crista terminalis was recovered as the EAS (arrow) during isoproterenol infusion. (b) RA voltage map. The middle and lower part of RA and crista terminalis is a low voltage area with gray color (<0.5 mV). AF: atrial fibrillation, SAN: sinoatrial node, RA: right atrial, EAS: earliest atrial activation site. Modified with permission from Joung et al.26)
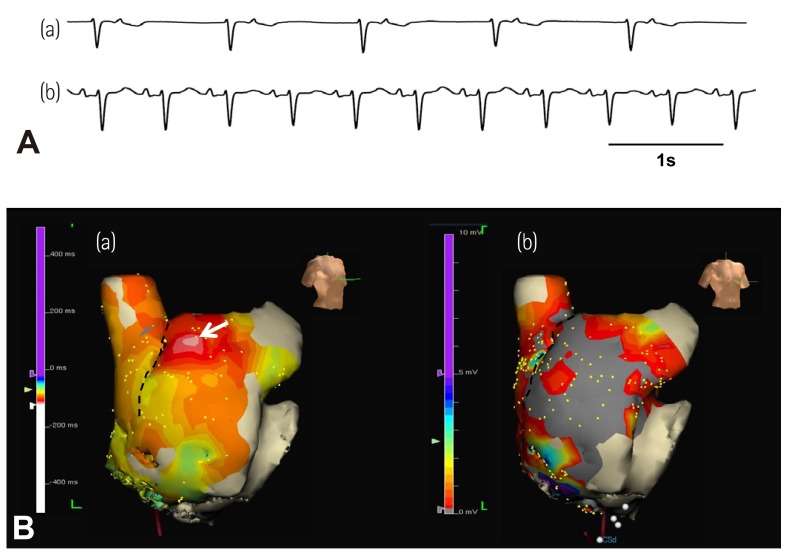
Fig. 3
Inferior shift of EAS during isoproterenol infusion in a patient taking amiodarone. A: RA activation map at baseline (left panel) and during isoproterenol infusion (right panel). EAS was shifted to the lower part of crista terminalis (arrow) during isoproterenol infusion. B: EKG at baseline (left panel) and during isoproterenol infusion (right panel). Note the decreased amplitude of P-waves in leads II, III, and aVF after inferior shift of EAS during isoproterenol infusion (arrows). EAS: earliest atrial activation site, RA: right atrial. Modified with permission from Mun et al.39)
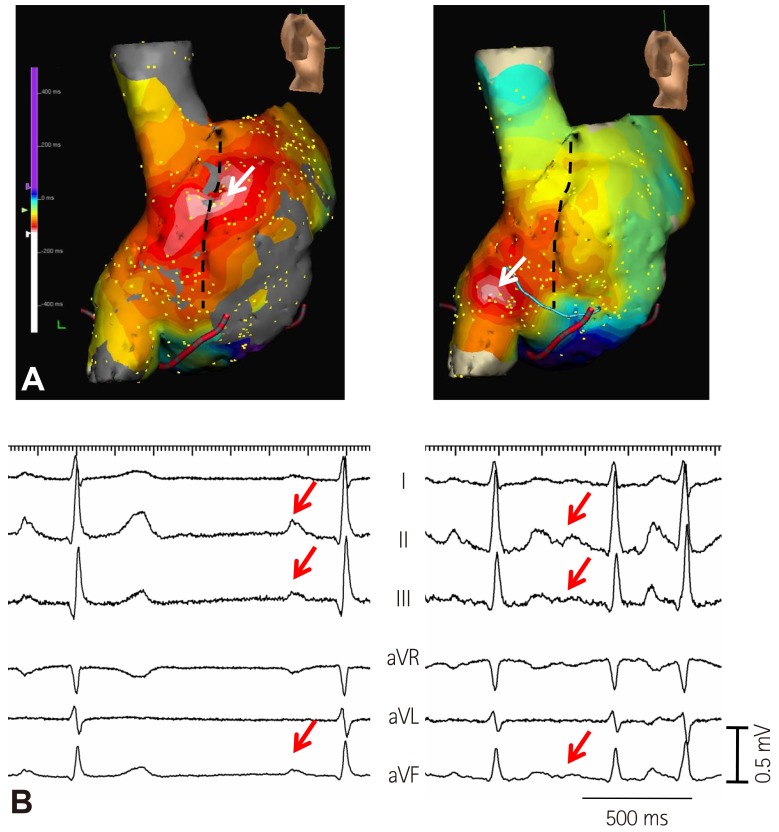
Fig. 4
Atrial tachycardia originating from lower crista terminalis. A: RA activation map showing sinus beat (a) and atrial tachycardia (b) from superior and inferior crista terminalis, respectively. B: EKG showing sinus beat (a) and atrial tachycardia (b). Atrial tachycardia originating from lower crista terminalis had decreased amplitude of P-waves in leads II, III, and aVF. RA: right atrium, CT: crista terminalis.
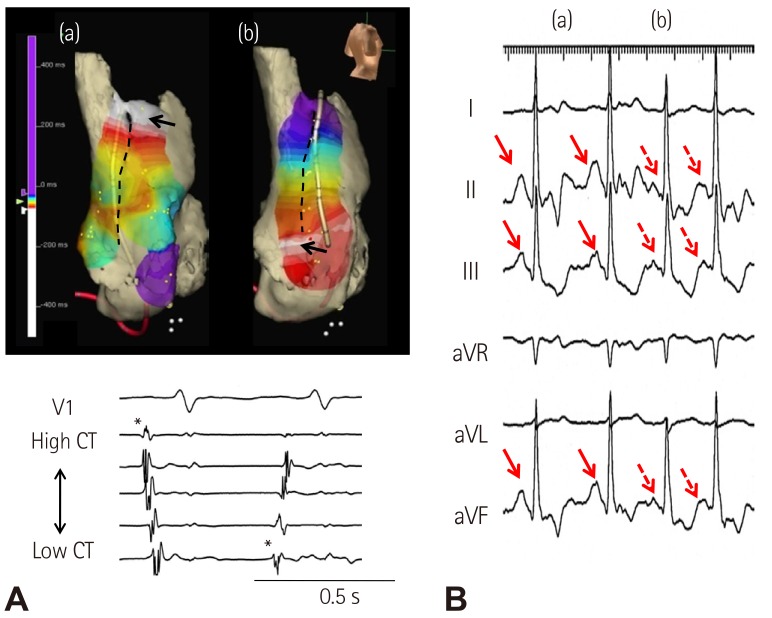
Fig. 5
The P-wave changes induced by sympathetic stimulation. A: the negative correlation between the distance from the SVC-RA junction to the most cranial EAS and P-wave amplitude in leads II (left panel), III (middle panel), and aVF (right panel). Unfilled and filled circles represent P-wave amplitude at baseline and during isoproterenol infusion, respectively. Black and red circles represent the patients without and with sick sinus syndrome (SSS), respectively. B: the change of P-wave amplitude in leads II (left panel), III (middle panel), and aVF (right panel) during isoproterenol infusion. Note that the patients with SSS tend to have low P-wave amplitude and low EAS location both at baseline and during isoproterenol infusion. SVC-RA: superior vena cavaright atria, EAS: earliest atrial activation site, aVF: , ISO: isoproterenol. Modified with permission from Mun et al.39)
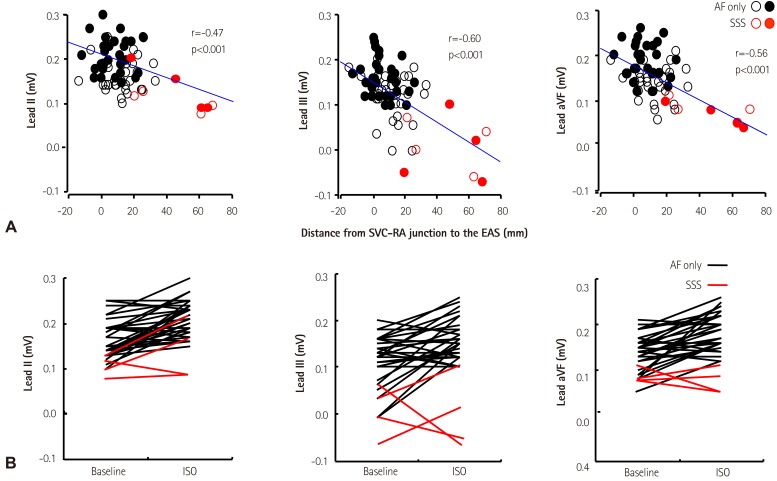
Fig. 6
Comparative Efficacy of Testing for Symptomatic Bradycardia. A,: the dot plots of the CSNRT from AF patients without (No SSS) and with sinus bradycardia (SSS) at each pacing cycle length (PCL) showing large overlaps between the two groups. The dashed line shows the CSNRT of 550 ms. B: the distance from the SVC-RA junction to the most cranial earliest activation site at baseline and during isoproterenol infusion. CSNRT: corrected sinoatrial node recovery time, AF: atrial fibrillation, SSS: sick sinus syndrome, SVC-RA: superior vena cava-right atrial, ISO: isoproterenol. Modified with permission from Joung et al.26)
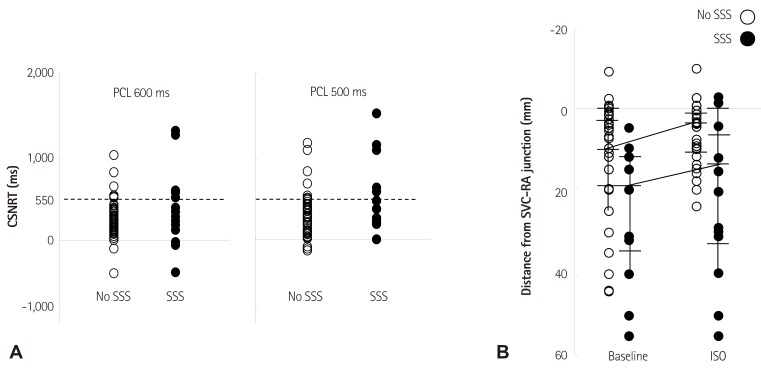