Abstract
Background and Objectives
Recent studies showed that, in addition to parasympathetic nerves, cervical vagal nerves contained significant sympathetic nerves. We hypothesized that cervical vagal nerve stimulation (VNS) may capture the sympathetic nerves within the vagal nerve and activate the stellate ganglion.
Materials and Methods
We recorded left stellate ganglion nerve activity (SGNA), left thoracic vagal nerve activity (VNA), and subcutaneous electrocardiogram in seven dogs during left cervical VNS with 30 seconds on-time and 30 seconds off time. We then compared the SGNA between VNS on and off times.
Results
Cervical VNS at moderate (0.75 mA) output induced large SGNA, elevated heart rate (HR), and reduced HR variability, suggesting sympathetic activation. Further increase of the VNS output to >1.5 mA increased SGNA but did not significantly increase the HR, suggesting simultaneous sympathetic and parasympathetic activation. The differences of integrated SGNA and integrated VNA between VNS on and off times (ΔSGNA) increased progressively from 5.2 mV-s {95% confidence interval (CI): 1.25-9.06, p=0.018, n=7} at 1.0 mA to 13.7 mV-s (CI: 5.97-21.43, p=0.005, n=7) at 1.5 mA. The difference in HR (ΔHR, bpm) between on and off times was 5.8 bpm (CI: 0.28-11.29, p=0.042, n=7) at 1.0 mA and 5.3 bpm (CI 1.92 to 12.61, p=0.122, n=7) at 1.5 mA.
Cervical vagal nerve stimulation (VNS) has been used clinically to treat drug refractory epilepsy.1) Three recent clinical trials have tested the effects of VNS on heart failure with mixed results.2)3)4) It has been hypothesized that VNS improved heart failure through parasympathetic nerve activation, that in turn reduced heart rate (HR) and inflammation.5)6) However, immunohistochemical studies showed that both cervical and thoracic vagal nerves contained abundant sympathetic components.7)8)9) It is likely that VNS activation of the sympathetic components of the cervical vagal nerve is also important to its therapeutic effects. Schwartz et al.10) showed that stimulating the central cut end of the cervical vagal nerve may suppress sympathetic discharges. Shen et al.11) also found that acute VNS can immediately suppress stellate ganglion nerve activity (SGNA) in dogs. However, after a few days of continuous VNS, there is further reduction of SGNA, suggesting stellate ganglion remodeling. Histological studies and Western blot analyses showed that intermittent VNS increased expression of the small conductance calcium activated K (SK) channel expression in the stellate ganglion.12) Increased SK current, that reduced somatic excitability of the neuron,13) can explain the mechanism by which SGNA is further reduced after several days of VNS. The mechanisms by which VNS caused stellate ganglion remodeling are unclear. We hypothesized that intermittent VNS may activate the stellate ganglion during on time by capturing the sympathetic component within the vagal nerve. Intermittent stellate ganglion activation then caused remodeling that reduced overall SGNA, including that during the off-time.
The animal protocol was approved by the Institutional Animal Care and Use Committee of the Indiana University School of Medicine and the Methodist Research Institute (Indianapolis, IN, USA) and conformed to the Guide for Care and Use of Laboratory Animals.
Seven adult male mongrel dogs (weighing 20 to 30 kg) were used in this study. All first (sterile) surgeries were performed under isoflurane inhalation general anesthesia. A small incision was made on the left anterior neck. The left cervical vagal nerve was identified and isolated from the left carotid artery. The nerve was then wrapped with a pair of Cyberonics spiral electrodes and a spiral anchor for stable bipolar stimulation. The cathode was cranial and the anode was caudal. The lead was connected to a subcutaneously positioned neurostimulator (VNS Therapy System; Cyberonics Inc., Houston, TX, USA). Subsequently, a left thoracotomy was performed through the 3rd intercostal space for the implantation of a radiotransmitter (D70-EEE, Data Sciences International, St. Paul, MN, USA) in all dogs studied, according to methods described previously.14)15) The first pair of electrodes was inserted beneath the fascia of the left stellate ganglion. A second pair of bipolar leads was attached to the left thoracic vagal nerve, 2 to 4 cm above the aortic arch. A third pair of bipolar electrodes was used to record subcutaneous electrocardiogram (ECG), with one electrode inserted under the subcutaneous tissue of left thorax and left lower abdomen. The transmitter and remaining wires were inserted into a subcutaneous pocket. The chest wall was then closed. After 2 weeks of recovery, the radiotransmitter was turned on to record nerve signals and ECGs. To reduce the number of animals used in experimental studies, the results of the baseline recording of the same dogs were also used in a recent manuscript.16)
We performed a left cervical VNS (frequency 30 Hz, pulse width 0.5 ms, duty cycle 30 seconds on alternating with 30 seconds off) for 24 hours in all seven dogs. The current output of VNS started at 0.25 mA for 24 hours. The output was then up-titrated every 24 hours in 0.25 mA increments until the dog failed to tolerate the VNS. Dry cough and drooling initially occurred between 0.75 mA to 1.0 mA, but these symptoms usually disappeared after continued stimulation. Intermittent vomiting and anorexia occurred at higher current outputs (1.5 mA to 2.0 mA) and marked the humane endpoints for the nerve stimulation. The lead impedances of all seven dogs were within normal range (1435 to 2702 ohms) during the study. There was no evidence of broken VNS leads in any of the dogs studied at the time of euthanasia.
We analyzed recordings from all channels using custom-made software. To optimize nerve signals, data from the left stellate ganglion and the left thoracic vagal nerve were high-pass filtered at 100 Hz. Nerve activities were then integrated every 30 seconds over 24 hours. The average integrated nerve activities during 30 seconds of VNS was compared to the average 30 seconds nerve activities without stimulation for each 24 hours period. We also compared the average HR during the on and off times. The data are presented as mean and 95% confidence interval. Paired t-tests were used to compare the SGNA, vagal nerve activity (VNA), and HR between on and off times.
To study the effects of VNS on SGNA, we first analyzed episodes of VNS that occurred at a time when SGNA was quiescent. Fig. 1A shows that cervical VNS induced large SGNA but minimal thoracic VNA at 0.75 mA output. These effects were associated with elevated HR and reduced HR variability, consistent with a net increase in sympathetic tone. Fig. 1B shows that doubling cervical VNS output to 1.5 mA in the same dog resulted in a large increase in both SGNA and thoracic VNA while once again decreasing HR variability. To further understand the mechanisms of SGNA induction, we analyzed the effects of VNS on SGNA at different VNS outputs. Fig. 2A shows 1.0 mA of aVNS induced small VNA response but even smaller immediate SGNA response. When we enlarged the 1 second period around the time of VNS onset (Fig. 2B), we observed periodic SGNA at about 33.3 ms intervals (30 Hz), consistent with 1:1 transmission of VNS-induced nerve activity to the left stellate ganglion. Fig. 2C shows the effects of VNS at 1.5 mA output in another dog. There is a burst-termination pattern of SGNA (arrows in Fig. 2C) after the first episode of VNS, consistent with afterdischarges induced by an indirect left stellate ganglion stimulation. The onset of cervical VNS was followed immediately by the SGNA, but no definite periodic activation was observed (Fig. 2D) during the initial portion of the stimulation. To eliminate the stimulus artifacts, we applied 30-Hz notch filter on the same signal. As shown in Fig. 3, removing the stimulus artifacts by 30 Hz notch filtering did not significantly alter the morphology of the SGNA.
Fig. 4 shows a different mode of SGNA induction. In this episode, VNS at 1.5 mA resulted in a transient complete heart block (black arrows point to P waves) within seconds after onset. Bradycardia persisted for approximately 10 seconds, followed by large SGNA discharges and HR acceleration. These findings were consistent with reflex elevation of SGNA rather than direct VNS excitation of the left stellate ganglion. These figures (Figs. 1, 2, 3, 4) also showed a lack of consistent relationship between HR and VNS.
To study the effects of VNS on VNA, we identified segments of the recordings in which VNS occurred at the time when SGNA was quiescent. Fig. 5 shows a representative example. The thoracic VNA did not show any response to the cervical VNS at 0.25 mA or 0.5 mA in any of the seven dogs studied. As the cervical VNS output was increased from 0.5 mA, however, the thoracic VNA increased in a dose-dependent manner. Integrated nerve activities were also examined to better understand overall trends in nerve activity. Fig. 5B shows a slight increase in integrated VNA (iVNA) during VNS at 0.5 mA. Fig. 5C shows further increase in VNS to 1.0 mA, resulting in an initial large VNA response (gray arrow) and followed by a rapid reduction of iVNA. Fig. 5D shows that, at 1.5 mA, there was a sharp increase of iVNA (arrow) followed by persistent high level of VNA. These changes were associated with a reduction of HR and reduced HR variability throughout the VNS. These findings suggested that high output VNS might have activated the parasympathetic component of the vagus nerve, thus causing sinus node suppression. Fig. 5E shows a magnified view of the initial portion of the VNA of Fig. 5D. Note, the thoracic VNA showed a burst-termination pattern during VNS. These patterns of activity are consistent with nerve discharge and not with the stimulus artifacts. Fig. 5F shows further magnification of the window between the two vertical red bars in Fig. 5E. Fig. 5F shows that the thoracic VNA occurred at about 33.3 microseconds intervals, consistent with 1:1 transmission from cervical VNS.
While left cervical VNS had a clear effect on both sympathetic and VNA, the HR response to VNS was variable. For example, in Fig. 5B, it is evident that increasing the VNS to 0.75 mA transiently increased the HR while reducing its variability (between two black arrows in Fig. 5B). The increased HR during a low output VNS suggested that VNS might have selectively activated the sympathetic component of the cervical vagal nerve, not through the activation of SGNA. However, in some dogs, VNS at 0.5 mA was associated with a reduction in HR. Thus, the relationship between low output VNS and HR acceleration was not always observed. Fig. 5C demonstrates a loss of HR variability during the first half but not the second half of the 30 seconds stimulation (between two black arrows).
We analyzed the HR responses to VNS in each of the 7 dogs studied. Fig. 6 shows the mean SGNA, VNA, and HR at different VNS outputs in each dog studied. There was no change in the HR between 'on' and 'off' windows with a VNS output of 0.25 mA. However, when the amplitude of VNS was titrated up to 0.5 mA, 4 of 7 dogs showed a significant increase in HR. One dog (D2) showed a gradual decrease in HR when VNS output was titrated above 1.0 mA. Overall, although the differences in HR (ΔHR) between on and off windows increased with increased VNS output, the HR itself did not consistently increase with increased VNS output. Similarly, although the differences in SGNA (ΔSGNA) in general increased with increased VNS output, the SGNA itself did not consistently increase with increased VNS output. In dogs 3 and 4, the SGNA of intermediate strength (1.5 mA and 1.75 mA in Dog 3, 0.75-1.75 mA in Dog 4) appear to be lower than the SGNA of either the low output stimulus (0.5 mA) or high output stimulus (2.0 mA).
To further determine if VNS increased the overall sympathetic tone, we averaged the integrated SGNA of all dogs studied at all VNS outputs (Fig. 7). Fig. 7A shows that the mean SGNA and mean VNA during on-time mostly increased with increased VNS output. However, during off-time, the SGNA is mostly lower than the baseline and dipped at 1.5 mA (red arrow) before rising above baseline. The VNA dipped at 0.75 mA (red arrow) before returning to baseline value. The increased SGNA and VNA were associated with HR reduction, with the maximum effect at 1.5 mA output. The latter finding showed that VNA exerts a dominant effect on HR at 1.5 mA (downward arrow).
Fig. 7B shows the differences (Δ) between on and off times. The delta was zero for SGNA, VNA, and HR at 0.25 mA. Increasing the VNS output was associated with increased SGNA and VNA, but the peak effect was reached at 1.75 mA for SGNA and 1.25 mA for VNA. The delta SGNA and VNA reached a plateau around 1.25-1.5 mA. The HR changes were more complex. The ΔHR (Fig. 7B) showed positive values, indicating that the VNS output at 0.5 mA or higher had resulted in a higher HR during the on time rather than during the off time.
We found that an intermittent cervical VNS might selectively capture the sympathetic components of the vagal nerve and excite the stellate ganglion at moderate (approximately 0.75 mA) output. Increasing the output might result in simultaneously sympathetic and parasympathetic capture. Both sympathetic and parasympathetic nerve stimulation may be important for the therapeutic effects of VNS.
While VNS has been studied for many years, the phenomenon of sympathetic activation during VNS in ambulatory animals has not been well documented. Because sympathetic nerves are peripherally located in the cervical vagal nerve,7) low output VNS may selectively activate these sympathetic nerves to increase HR and reduce HR variability. That phenomenon was observed in the present study. In addition, anatomical studies have documented rich interactions between the left thoracic vagal nerve and the left stellate ganglion at all levels.17,18,19) Therefore, it is possible that VNS can directly activate the stellate ganglion through these interacting nerve fibers. This may also be the reason why the amplitudes of SGNA increase with increasing VNS output. In addition to direct sympathetic stimulation, VNS can also cause reflex SGNA activation after VNS-induced bradycardic episodes during a transient complete heart block. The increased SGNA competed successfully with the parasympathetic effects of VNS on the AVN, resulting in tachycardia.
Nervous systems are highly plastic. The rapid activation of the stellate ganglion may be responsible for the remodeling changes in the stellate ganglion observed in dogs with VNS.12) It is possible that VNS with a short on time is sufficient to remodel the left stellate ganglion, leading to a significant reduction of the nerve discharges during the off time. If the off time is optimally programmed, it is possible to achieve an overall reduction of the sympathetic outflow and control cardiac diseases. Consistent with the latter hypothesis, a recent clinical trial successfully used a duty cycle of 17.5% (14 seconds on, 66 off) to achieve therapeutic effects in heart failure.2)20)
We documented that, at higher outputs (>1.0 mA), both SGNA and VNA were higher during the on-time than off-time, suggesting simultaneous sympathetic and parasympathetic nerve captures. It is known that combined sympathetic and parasympathetic activation is highly arrhythmogenic in the atria. Sympathetic activation increases intracellular calcium while parasympathetic activation shortens the action potential duration. These effects promote a late phase 3 early, afterdepolarization to cause atrial fibrillation (AF).21)22) High output cervical VNS has been frequently used to induce AF during animal studies.23) The mechanism of AF induction during high output VNS may be attributed to the proarrhythmic effects of simultaneous sympathetic and parasympathetic activations.
The burst-termination pattern is well documented in single neurons as well as in the stellate ganglion of ambulatory dogs.14)24) This phenomenon occurs because rapid nerve discharges result in intracellular calcium accumulation that activates SK channels to cause after-hyperpolarization and prevent continuous nerve discharges.13)25) In this study, we documented burst-termination pattern of VNA and SGNA in response to cervical VNS. Furthermore, we documented that the response of VNA and SGNA was higher at the onset of the VNS rather than during the latter part of the VNS. These observations were important to the programming of the VNS as the response to the VNS was expected to be the most robust at the beginning of the therapy. Prolonged VNS may be counterproductive because the SK current activation may prevent effective neural stimulation.
One limitation of the study was that only the left vagus nerve was subjected to stimulation; whether or not these findings are relevant to right VNS remains unclear. Other limitations include the fact that we only have information on 50% duty cycle and 30 Hz stimulation. Thus, the optimal programming parameters for VNS in treating various diseases remain unclear. A third limitation was that we did not study the time-dependent changes of SGNA. It is possible that a longer duration of intermittent VNS may result in stellate ganglion remodeling and reduce the SGNA, as shown in previous studies.11)12)
Figures and Tables
Fig. 1
The effects of cervical VNS on SGNA, thoracic VNA, and HR. Red horizontal lines indicate VNS. A: VNS at 0.75 mA increased SGNA and HR with minimal increase of VNA. B: VNS at 1.5 mA induced both stronger SGNAs with burst termination patterns (arrows) and distinct VNA. However, no apparent increase of HR was noted. VNS: vagal nerve stimulation, SGNA: stellate ganglion nerve activity, HR: heart rate, VNA: vagal nerve activity, ECG: electrocardiogram.
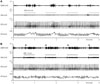
Fig. 2
Immediate induction of SGNA by cervical VNS. Red horizontal bars indicate the 30 seconds of "on-time" of VNS, which was followed by 30 seconds of off time. A: cervical VNS 1.0 mA (started at the blue vertical lines) induced small VNA and even smaller SGNA, without obvious changes of HR. B: the onset of VNA in greater detail by changing the vertical calibration and, by expanding to 1 second duration around the time of stimulation. There was SGNA activating at about 33.3 microseconds interval (30 Hz), consistent with a 1:1 transmission of cervical VNS to the stellate ganglion. C: cervical VNS at 1.5 mA in another dog induced a larger SGNA response and an initial HR acceleration. D: the detailed recording of SGNA at the beginning of VNS in C. The nerve activities immediately exhibited complex activation patterns at the onset of VNS (blue vertical line), without apparent 30 Hz activation patterns. VNS: vagal nerve stimulation, SGNA: stellate ganglion nerve activity, HR: heart rate, VNA: vagal nerve activity, ECG: electrocardiogram.
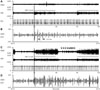
Fig. 3
Effects of notch filtering. A: the frequency distribution of the same episode of data shown in Fig. 2. The frequency of pacing artifacts (arrow on the left panel) was detected at 30 Hz. Signals associated with that frequency were removed by applying a 30 Hz notch filter (arrow on the right panel). B: the raw signals, signals after 30 Hz notch filter, and signals after both notch filtering and 150 Hz high pass filter. Removing the stimulus artifacts by 30 Hz notch filtering did not significantly alter the morphology of the SGNA. HP: high pass.
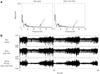
Fig. 4
Reflex activation of SGNA by bradycardia induced by cervical VNS. VNS at 1.5 mA resulted in initial bradycardia (atrioventricular block). Arrows point to non-conducted P waves. The bradycardia was followed by a large SGNA and increased HR. Arrow indicates VNS at 1.5 mA. VNS: vagal nerve stimulation, SGNA: stellate ganglion nerve activity, HR: heart rate, VNA: vagal nerve activity, ECG: electrocardiogram.
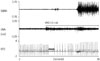
Fig. 5
Effects of the cervical VNS on SGNA, thoracic VNA, HR, iSGNA, and iVNA. We chose for analyses the time segments without either SGNA or VNA prior to the onset of VNS. Red horizontal bars indicated the 30-seconds "on-time" of VNS and was followed by 30-seconds of off time. A: VNS at 0.5 mA had no effects on either SGNA or VNA. B-D: a progressively increased VNS strength resulting in the suppression of the HR variability and increased VNA. The iVNA was the largest at the beginning of VNS and progressively reduced over time. This finding indicated progressively reduced amplitudes of VNA responses to the cervical VNS. Strong VNS may reduce the HR in the absence of SGNA (D). E: the detailed recording at the beginning of VNS in D. There was a "burst termination" pattern typical of nerve discharges. F: further magnification of E from the period between the red vertical bars. The interval between red arrows (33.3 microseconds) showed the frequency of thoracic VNA and was the same at the frequency of VNS (30 Hz). The latter finding indicated a one-to-one activation of thoracic vagus nerve by cervical VNS. VNS: vagal nerve stimulation, SGNA: stellate ganglion nerve activity, HR: heart rate, VNA: vagal nerve activity, ECG: electrocardiogram, iSGNA: integrated SGNA, iVNA: integrated VNA, TVNA: thoracic vagus nerve activity.
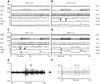
Fig. 6
The SGNA, VNA, and HR of all dogs. D1 to D7 identified dogs 1 to 7, respectively. The abscissa showed the baseline (B) and the mA outputs (0.25 to 2). The SGNA from consecutive 30 seconds VNS 'on (red)' and 'off (blue) windows' for 24 hours are compared using paired t-tests. The HR responses of D3, D4, and D5 roughly followed that of the integrated SGNAs. However, in the remaining dogs, the SGNA continued to increase but the HR did not increase with the increased VNS outputs. *p<0.05. VNS: vagal nerve stimulation, SGNA: stellate ganglion nerve activity, HR: heart rate, VNA: vagal nerve activity, ECG: electrocardiogram.
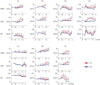
Fig. 7
Changes of average nerve activities and HRs according to the strength of cervical VNS. The abscissa showed the mA outputs (0.25 to 2). The ordinate is the integrated nerve activity. A: showed the nerve activities and HR of VNS on (red) and off (blue) averaged from all seven dogs. B: showed the differences (Δ) of average nerve activities and HRs between the on and off windows. Both average nerve activities and HRs during the on windows were higher than those of off windows for VNS of 0.75 mA or above. *p<0.05. VNS: vagal nerve stimulation, SGNA: stellate ganglion nerve activity, HR: heart rate, VNA: vagal nerve activity, ECG: electrocardiogram, B: baseline.
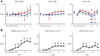
Acknowledgments
We thank Nicole Courtney, Jessica Warfel, Lei Lin, and Jian Tan for their assistance. We also thank Medtronic Inc., St Jude Inc., and Cyberonics Inc. for donating research equipment to our laboratory.
This study was supported in part by National Institutes of Health grants P01 HL78931, R01 71140, R21 HL106554, R41HL124741, a Piansky Endowment (M.C.F.), a Medtronic-Zipes Endowment (P.S.C.), the Indiana University Health-Indiana University School of Medicine Strategic Research Initiative and Chonbuk National University, Jeonju, Korea. The content is solely the responsibility of the authors and does not necessarily represent the official views of the National Institutes of Health.
References
1. Terry R. Vagus nerve stimulation: a proven therapy for treatment of epilepsy strives to improve efficacy and expand applications. Conf Proc IEEE Eng Med Biol Soc. 2009; 2009:4631–4634.
2. Premchand RK, Sharma K, Mittal S, et al. Autonomic regulation therapy via left or right cervical vagus nerve stimulation in patients with chronic heart failure: results of the ANTHEM-HF trial. J Card Fail. 2014; 20:808–816.
3. Zannad F, De Ferrari GM, Tuinenburg AE, et al. Chronic vagal stimulation for the treatment of low ejection fraction heart failure: results of the neural cardiac therapy for heart failure (NECTAR-HF) randomized controlled trial. Eur Heart J. 2014; 36:425–433.
4. De Ferrari GM, Crijns HJ, Borggrefe M, et al. Chronic vagus nerve stimulation: a new and promising therapeutic approach for chronic heart failure. Eur Heart J. 2011; 32:847–855.
5. Klein HU, Ferrari GM. Vagus nerve stimulation: a new approach to reduce heart failure. Cardiol J. 2010; 17:638–644.
6. Sabbah HN. Electrical vagus nerve stimulation for the treatment of chronic heart failure. Cleve Clin J Med. 2011; 78:Suppl 1. S24–S29.
7. Onkka P, Maskoun W, Rhee KS, et al. Sympathetic nerve fibers and ganglia in canine cervical vagus nerves: localization and quantitation. Heart Rhythm. 2013; 10:585–591.
8. Kawagishi K, Fukushima N, Yokouchi K, Sumitomo N, Kakegawa A, Moriizumi T. Tyrosine hydroxylase-immunoreactive fibers in the human vagus nerve. J Clin Neurosci. 2008; 15:1023–1026.
9. Seki A, Green HR, Lee TD, et al. Sympathetic nerve fibers in human cervical and thoracic vagus nerves. Heart Rhythm. 2014; 11:1411–1417.
10. Schwartz PJ, Pagani M, Lombardi F, Malliani A, Brown AM. A cardiocardiac sympathovagal reflex in the cat. Circ Res. 1973; 32:215–220.
11. Shen MJ, Shinohara T, Park HW, et al. Continuous low-level vagus nerve stimulation reduces stellate ganglion nerve activity and paroxysmal atrial tachyarrhythmias in ambulatory canines. Circulation. 2011; 123:2204–2212.
12. Shen MJ, Hao-Che Chang, Park HW, et al. Low-level vagus nerve stimulation upregulates small conductance calcium-activated potassium channels in the stellate ganglion. Heart Rhythm. 2013; 10:910–915.
13. Adelman JP, Maylie J, Sah P. Small-conductance Ca2+-activated K+ channels: form and function. Annu Rev Physiol. 2012; 74:245–269.
14. Ogawa M, Zhou S, Tan AY, et al. Left stellate ganglion and vagal nerve activity and cardiac arrhythmias in ambulatory dogs with pacing-induced congestive heart failure. J Am Coll Cardiol. 2007; 50:335–343.
15. Tan AY, Zhou S, Ogawa M, et al. Neural mechanisms of paroxysmal atrial fibrillation and paroxysmal atrial tachycardia in ambulatory canines. Circulation. 2008; 118:916–925.
16. Robinson EA, Rhee KS, Doytchinova A, et al. Estimating sympathetic tone by recording subcutaneous nerve activity in ambulatory dogs. J Cardiovasc Electrophysiol. 2015; 26:70–78.
17. Mizeres NJ. The anatomy of the autonomic nervous system in the dog. Am J Anat. 1955; 96:285–318.
18. Ellison JP, Williams TH. Sympathetic nerve pathways to the human heart, and their variations. Am J Anat. 1969; 124:149–162.
19. Armour JA, Hageman GR, Randall WC. Arrhythmias induced by local cardiac nerve stimulation. Am J Physiol. 1972; 223:1068–1075.
20. Dicarlo L, Libbus I, Amurthur B, Kenknight BH, Anand IS. Autonomic regulation therapy for the improvement of left ventricular function and heart failure symptoms: the ANTHEM-HF study. J Card Fail. 2013; 19:655–660.
21. Burashnikov A, Antzelevitch C. Reinduction of atrial fibrillation immediately after termination of the arrhythmia is mediated by late phase 3 early afterdepolarization-induced triggered activity. Circulation. 2003; 107:2355–2360.
22. Patterson E, Po SS, Scherlag BJ, Lazzara R. Triggered firing in pulmonary veins initiated by in vitro autonomic nerve stimulation. Heart Rhythm. 2005; 2:624–631.
23. Goldberger AL, Pavelec RS. Vagally-mediated atrial fibrillation in dogs: conversion with bretylium tosylate. Int J Cardiol. 1986; 13:47–55.
24. del Negro CA, Hsiao CF, Chandler SH. Outward currents influencing bursting dynamics in guinea pig trigeminal motoneurons. J Neurophysiol. 1999; 81:1478–1485.
25. Köhler M, Hirschberg B, Bond CT, et al. Small-conductance, calciumactivated potassium channels from mammalian brain. Science. 1996; 273:1709–1714.