Abstract
Background and Objectives
Vascular wall remodeling in pulmonary hypertension can be caused by an aberration in the normal balance between proliferation and apoptosis of endothelial cell in the pulmonary artery. The objective of this study was to evaluate the effect of bosentan on apoptosis in monocrotaline (MCT)-induced pulmonary hypertension.
Materials and Methods
Sprague-Dawley rats were divided into three groups: control (C) group, M group (MCT 60 mg/kg) and B group (MCT 60 mg/kg plus bosentan 20 mg/day orally). Gene expressions of Bcl (B cell leukemia/lymphoma)-2, caspase-3, complement component (C)-6, vascular endothelial growth factor (VEGF), interleukin (IL)-6 and tumor necrosis factor-alpha (TNF-α) were analyzed by real time polymerase chain reaction and western blot analysis.
Results
The messenger ribonucleic acid (mRNA) expressions of caspase-3 and VEGF were significantly increased in the M group compared with the C group, and significantly decreased in the B group compared with the M group in week 4. mRNA expression of IL-6 was significantly decreased in weeks 1, 2, and 4 in the B group compared with the M group. mRNA expression of TNF-α was significantly decreased on day 5 and in weeks 1 and 2 in the B group compared with the M group.
Pulmonary arterial hypertension (PAH) is a disease characterized by elevated pulmonary artery pressures. PAH is associated with significant morbidity and mortality.1) Elevated pulmonary vascular resistance results in high right ventricular systolic pressures causing right ventricular remodeling, ultimately progressing to heart failure.
Pulmonary arterial hypertension is a progressive and lethal disease. Current therapies are mostly vasodilator agents and they may be less effective in treating arterial remodeling.2) The lung pathology of PAH is characterized by medial hypertrophy and intimal hyperplasia of muscular arteries, as well as plexiform lesions, which lead to a widespread narrowing or obliteration of the pulmonary arteriolar bed.2) However, the pathogenesis of the functional and structural abnormalities of the lung microcirculation in PAH is poorly understood.
Apoptosis is the organized cell death process that is associated with less inflammation compared to necrosis.3)4) Apoptotic cells are phagocytized by macrophages before their membrane breaks down, so that their intracellular enzymes are not released. Apoptosis is triggered and modulated by two pathways. The intrinsic pathway involves the mitochondria and is activated by reactive oxygen species, whereas the extrinsic pathway is activated when ligands bind to their receptors, for instance tumor necrosis factor-alpha (TNF-α) to TNF receptors and Fas-ligand to Fas.5) Activation is rapid for the intrinsic pathway, but may take up to several hours for the extrinsic pathway.4)
Recent studies in idiopathic PAH have implicated a failure of vascular smooth muscle apoptosis in the disease pathogenesis.6)7) However, data about endothelial cell apoptosis and proliferation are rare in idiopathic PAH.8) Other recent studies have suggested a role for periarterial inflammatory cell mediators in the pathogenesis of PAH.9) The idea that endothelial cell (EC) apoptosis may play a central role in the initiation and progression of PAH suggests that therapeutic strategies aimed at endothelial repair and regeneration of ECs may be uniquely effective in the treatment.2)
Monocrotaline (MCT) is a selective endothelial toxin and increases vasoconstrictor responsiveness in the lungs of rats,10) alveolar capillary membrane permeability,11) inflammation12) and EC injury.13) The presence of MCT-induced degenerative changes in the pulmonary endothelium in vivo and the evidence of cross-linking of DNA in MCT treatment to cultured ECs14) suggests that endothelial injury and endothelial apoptosis may be a triggering event in MCT-induced PAH.
Bosentan is an orally active endothelin (ET) receptor antagonist having non-selective, dual action against ETA and ETB.15) Bosentan significantly reduces PAH, pulmonary vascular hypertrophy and right ventricular hypertrophy in rats treated with MCT.16)
We studied the expressions of Bcl (B cell leukemia/lymphoma)-2, caspase-3, complement component (C)-6, vascular endothelial growth factor (VEGF), interleukin (IL)-6 and TNF-α genes in a pulmonary hypertensive rat model. Finally, we investigated the effects of bosentan on apoptosis and inflammation in this rat model.
Six-week-old male Sprague-Dawley rats weighing between 200-250 g were housed in climate-controlled conditions with a 12 hours light : 12 hours dark cycle and free access to chow and water. PAH was induced by subcutaneous (sc) injection of 60 mg/kg MCT (Sigma-Aldrich, St. Louis, MO, USA). The rats were grouped into a control (C) group (n=36) that received sc injection of normal saline (0.1 mL/kg); M group (n=36) that received sc injection of MCT and B group (n=36) that received sc injection of MCT +20 mg/day bosentan (Actelion Pharmaceuticals, Allischwil, Switzerland) by gavage twice a day. The rats were sacrificed after 1, 5, 7, 14, and 28 days. Lung tissues were removed and immediately frozen at -70℃ for enzyme analysis, post-fixed in 10% formalin and processed routinely for paraffin embedding. The animal studies were performed after receiving approval of the Institutional Animal Care and Use Committee (IACUC) in Ewha Womans University (IACUC approval No. ESM-2010-0141).
Total ribonucleic acid (RNA) was extracted by using TRIzol reagent (Invitrogen, Carlsbad, CA, USA) as described,16) and resuspended in diethyl pyrocarbonate water. Final RNA amount was spectrophotometrically determined at 260/280 nm. Quality was assessed as the absence of smear of 18s rRNA or gapdh bands in examination using a Bio analyzer 2100 (Agilent, Sunnyvale, CA, USA). RNA samples were stored at -70℃ until used. Complementary deoxyribonucleic acids (cDNAs) were synthesized by 1 µg of total RNA, according to the manufacture's protocol16) (High Capacity RNA-to-cDNA kit; Applied Biosystems, Foster City, CA, USA).
Real-time quantitative polymerase chain reaction (PCR) was performed in triplicate using the Prism 7900 Sequence Detection System (Applied Biosystems, Foster City, CA, USA) in white-colored 384-well plates (ABgene, Hamburg, Germany) according to the manufacturer's instructions16) for intensification of the fluorescent signals by a factor of three. The system operated using a thermal cycler and a laser that was directed via fiber optics to each sample well. The fluorescence emission from each sample was collected by a charge-coupled device-camera and the quantitative data were analyzed using the Sequence Detection System version 2.0 software (Applied Biosystems, Foster City, CA, USA). Reaction mixtures contained 10 pmol/µL of each primer and 2X SYBR Green PCR Master Mix (Toyob, Osaka, Japan), which included the HotStarTaqt DNA-Polymerase in an optimized buffer, dNTP mix (with dUTP additive), SYBR Green I fluorescent dye and ROX dye as a passive reference. Each of the 384-well real-time quantitative PCR plates included serial dilutions (1, 1/2, and 1/4) of cDNA, which were used to generate relative standard curves for genes.
The resulting first-strand of cDNA was normalized to the glyceraldehyde 3-phosphate dehydrogenase (GAPDH) gene. The normalized cDNA was used for the PCR procedure as a template. The specific primers for each gene are shown in Table 1. All primers were amplified using the same conditions. Thermal cycling conditions 50℃ for 2 minutes and 95℃ for 10 minutes followed by 40 cycles of 95℃ for 30 seconds and 60℃ for 30 seconds, and 72℃ for 30 seconds. To exclude the presence of unspecific products, a melting curve analysis of products was performed routinely after finishing amplification by a high-resolution data collection during an incremental temperature increase from 60℃ to 95℃ with a ramp rate of 0.21℃/second. Real-time PCR cycle numbers were converted to gene amounts (ng) on the basis of the equation. The real-time PCR analysis was performed on an Applied Biosystems Prism 7900 Sequence Detection System (Fig. 1).
Tissue was homogenized in 10 mM Tris HCl buffer, pH 7.4, containing 0.5 mM ethylenediaminetetraacetic acid, pH 8.0, 0.25 M sucrose, 1 mM phenylmethylsulfonyl fluoride, 1 mM Na4VO3 and a protease inhibitor cocktail (Roche-Boehringer-Mannheim, Mannheim, Germany). After centrifugation, the supernatant containing 25 µg of protein was subjected to 10% sodium dodecyl sulfate polyacrylamide gel electrophoresis (SDS-PAGE). The proteins on the acrylamide gel were transferred to a polyvinylidene difluoride membrane (Millipore, Bedford, MA, USA) at 400 mA in a transfer buffer containing 25 mM Tris and 192 mM glycine, pH 8.4. The membrane was blocked in Tris-buffered saline with 5% non-fat dry milk and 0.1% Tween-20 at room temperature for 1 hour, and incubated with the appropriate primary antibody to Bcl-2, caspase-3, C-6, VEGF, IL-6, TNF-α (Cell Signaling Technology, Danvers, MA, USA) or GAPDH (Santa Cruz Biotechnology, Santa Cruz, CA, USA), at 4℃ overnight. The membrane was incubated with horseradish peroxidase-conjugated secondary antibody (Cell Signaling Technology) for 1 hour at room temperature. After washing, the membrane was visualized by a chemiluminescent reaction using an enhanced chemiluminescence detection kit (GE Healthcare, Piscataway, NJ, USA). The cell lysate from the solubilized tissue was mixed with BCA reagents (Pierce, Rockford, IL, USA) and incubated for 30 minutes. The protein content was quantified with an ELISA reader (Molecular Devices, Sunnyvale, CA, USA) at 562 nm based on a bovine serum albumin (BSA) standard curve.
The terminal deoxynucleotidyl transferase dUTP nick end labeling (TUNEL) assay was performed following the manufacturer's instructions (Roche, Basel, Switzerland). Paraffin sections of lung were deparaffinized twice for 5 minutes each and rehydrated in a series of serially diluted ethanol solutions (100%, 95%, 70%, and H2O). Each section was treated with proteinase K (final concentration 10 µg/mL in 10 mM Tris-HCl, pH 7.4-7.8) for 10 minutes at room temperature. A labeling reaction was prepared by mixing 45 µl of TUNEL-label solution with 5 µL of TUNEL enzyme, adding the mixture to the tissue sections, and incubating for 60 minutes at 37℃ in a humidified chamber in the dark. A negative control was prepared without TUNEL enzyme. Tissue sections were washed three times with phosphate buffered saline (PBS) and analyzed using confocal microscopy (Carl Zeiss, Jena, Germany).
Results are expressed as the mean±standard deviation. Differences between all other parameters for the three groups were evaluated by ANOVA followed by multiple-group comparisons with the Bonferroni correction. Statistical analysis was performed using the Statistical Package for Social Science version 13.0 software (SPSS, Chicago, IL, USA) and a p<0.05 was considered statistically significant.
Expressions of several mRNAs in rat lung tissues were examined. The expression of Bcl-2 mRNA was significantly increased in the M and B groups compared with the C group in week 4 (4.41±0.49 vs. 1±0.30, p=0.023) (Fig. 2A). The gene expression of Bcl-2 mRNA was not significantly different after bosentan treatment in week 4 (Fig. 2A). The expression of caspase-3 mRNA was significantly increased in the M group compared with the C group in week 4 (7.77±2.29 vs. 1±0.52, p=0.011) (Fig. 2B). The expression of caspase-3 mRNA was significantly decreased after bosentan treatment in week 4 (7.77±2.29 vs. 4.79±1.84, p=0.042) (Fig. 2B). The expression of C-6 mRNA was significantly increased in the M group compared with the C group in week 4 (1006.11±174.54 vs. 1±0.62, p=0.001) (Fig. 3A). The gene expression of C-6 mRNA was significantly decreased after bosentan treatment in week 4 (1006.11±174.54 vs. 218.06±172.43, p=0.035) (Fig. 3A). The expression of VEGF mRNA was significantly increased in the M group compared with the C group in week 4 (45.48±1.7 vs. 1±0.49, p=0.001) (Fig. 3B). The expression of VEGF mRNA was significantly decreased after bosentan treatment in week 4 (45.48±1.7 vs. 12.85±2.8, p=0.043) (Fig. 3B). Expression of IL-6 mRNA was significantly increased in weeks 1, 2, and 4 in the M group compared with the C group. mRNA expression of IL-6 was significantly decreased in weeks 1, 2, and 4 in the B group compared with the M group (p<0.05) (Fig. 4A). mRNA expression of TNF-α was significantly increased on day 5 in the M group compared with the C group (p<0.05) (Fig. 4B). mRNA expression of TNF-α was significantly decreased on day 5 and weeks 1 and 2 in the B group compared with the M group (p<0.05) (Fig. 4B).
In western blot analyses, significant decreases in protein production were evident in the B group compared with the M group at week 4 in C-6 (p=0.041) (Fig. 5A), VEGF (p=0.045) (Fig. 5B), IL-6 (p=0.023) (Fig. 6A) and TNF-α (p=0.029) (Fig. 6B). The TUNEL assay revealed enhanced cell death was observed in the M group compared with the C group. In contrast, apoptotic cell death in the B group was ameliorated compared with the M group (Fig. 7).
This study provides evidence that MCT increases apoptosis and inflammation, and that bosentan improves apoptosis and inflammation in a rat model of PAH. The expressions of Bcl-2, caspase-3 and VEGF mRNA were significantly increased in the M group compared with the C group in week 4. Apoptosis was more pronounced in the M group compared to the C group. Expression of IL-6 mRNA was significantly increased in weeks 1, 2, and 4 in the M group compared with the C group. Expression of TNF-α mRNA was significantly increased on day 5 in the M group compared with the C group. The expressions of caspase and VEGF mRNA were significantly decreased in week 4 after bosentan treatment, and expression of TNF-α mRNA was significantly decreased on day 5 and in weeks 1 and 2 after bosentan treatment, while expression of IL-6 mRNA was significantly decreased in weeks 1, 2, and 4 after bosentan treatment. Apoptotic cell death was ameliorated after bosentan treatment.
In the present and previous our studies, increased gene expression of endothelin-1 (ET-1),15) matrix metalloproteinase-2,16) TNF-α and IL-6 were evident as early as 5-7 days in the course of MCT injection, suggesting their contribution to the progression of cardiopulmonary pathology in MCT-induced pulmonary hypertensive rats. Up-regulation of endothelial nitric oxide synthase,16) Bcl-2, caspase-3, C-6, and VEGF genes were significant in week 4 because they are involved in the compensation mechanism.
These data suggest that prevention of EC death and inflammation occurs after bosentan treatment, implicating this treatment in the prevention of the development of PAH. Increased EC death is the likely explanation for the endothelial function impairment, most notably the deficient nitric oxide production as documented in our previous study.16) We confirmed that MCT-induced PAH.
Apoptosis is the process of normal programmed cell death that allows normal cell turnover and remodeling in the vasculature.17) Other recent studies have implicated resistance to apoptosis as being important in the pathogenesis of vascular proliferation in experimental and cell culture studies.18)19) EC apoptosis has been implicated as an initiating event in experimental PAH, leading either directly to the degeneration of pre-capillary arterioles or to the selection of hyperproliferative, apoptosis-resistant ECs that may contribute to angioproliferative plexiform lesions.2) Excessive EC injury and apoptosis can contribute to increased pulmonary vascular resistance by three complementary pathways: directly by EC drop out and precapillary microvascular drop out; by leading to endothelial dysfunction which together with loss of inhibitory regulation of smooth muscle cell (SMC) growth can lead to arteriolar remodeling; and by creating the conditions that favor the emergence of abnormal, hyperproliferative ECs.7) Irreversible PAH is strongly associated with impaired EC apoptosis and antiapoptotic signaling from perivascular inflammatory cells.7) These changes are associated with intimal proliferation and vessel narrowing, may contribute to clinical outcomes associated with PAH.7)
The role of proapoptotic and antiapoptotic proteins in PAH has been suggested, both as an initiating mechanism in the pathogenesis of PAH and as a potential mechanism for the survival of proliferative ECs and compensatory angiogenesis in irreversible PAH. The triggering events that are responsible for the imbalance between vasoconstrictors and vasodilators and endothelial dysfunction remain unknown.2) Both the MCT and chronic hypoxia models of PAH have been shown to be associated with reduced levels of VEGF transcripts. 20) VEGF is not only a potent EC trophic and pro-angiogenic factor, but also a potent survival factor protecting ECs from apoptosis mediated through the extrinsic pathway.21) Compensatory upregulation of VEGF in response to occluded vessels has also been found in systemic arteriopathies, such as diabetic retinopathy and age-related macular degeneration.22)
Our model was also characterized by marked endothelial apoptosis as evidenced by activated expressions of caspase-3 and Bcl-2 genes and TUNEL assay. An aberration in the normal balance between proliferation and apoptosis of SMCs contributes to vascular remodeling in PAH.23)24) These findings led us to hypothesize that ET-1 may modulate remodeling by inhibiting apoptosis of SMCs.23)
Endothelin-1 also prevented up-regulation of pro-apoptotic Bax and cleaved activated caspase-3. ET-1 has anti-apoptotic effects on vascular cells derived from adult animals, including ECs, fibroblasts and systemic SMCs.23) The mechanisms by which ET-1 modulates apoptosis in SMCs are unclear. ET-1 prevents many cell types from apoptosis-inducing stimuli including paclitaxel, nitric oxide, or serum deprivation.23)
In addition to being the most potent vasoconstrictor, ET-1 has been reported to directly modulate growth in vascular SMCs derived from adult humans and rats by acting as an autocrine/paracrine mitogen. 24) ET expression is increased in the lungs of patients with PAH25) and plasma levels of ET-1 are elevated in all cases of PAH.15) Both ETA-selective and combined ETA, ETB antagonists markedly attenuate the development of PAH in rat models and decrease pulmonary artery pressure.26) Other studies suggest that ET-1 is an important survival and anti-apoptotic factor for rat pulmonary arterial smooth muscle, which may at least partly account for the reported efficacy of ET receptor antagonists in the prevention and treatment of pulmonary vascular remodeling in newborn animals.27) Studies utilizing various ET receptor antagonists have confirmed that ET-1 is critical to the pathogenesis of vascular remodeling in both adult and newborn27) rodent models of chronic PAH.
Recent results from studies using the dual ET receptor antagonist, bosentan, as well as studies of a more selective ETA receptor antagonist, demonstrated the alleviation of symptoms and slowing in the progression of disease in some patients.28) Bosentan blocks the apoptotic cascade before the development of PAH. Although the exact pathophysiology remains unknown, there is increasing evidence to suggest an important role for inflammation in PAH. There is substantial evidence supporting a role for inflammatory cytokines in the development of idiopathic PAH.29) Animal models also support the role of inflammatory cytokines in the initiation and progression of PAH. IL-6 is consistently elevated in animal models of experimental PAH.30)
In our study, expression of IL-6 mRNA was significantly increased in weeks 1, 2, and 4 in the M group. mRNA expression of IL-6 was significantly decreased 1 week after bosentan treatment. mRNA expression of TNF-α was significantly increased on day 5 in the M group. mRNA expression of TNF-α was significantly decreased on day 5, in weeks 1 and 2 after bosetan treatment.
In conclusion, increased EC apoptosis and inflammatory cells are the pathogenesis of PAH. Administration of bosentan reduced apoptosis and inflammation. Further studies would be required to better understand the actual pathophysiological sequence of events and basic mechanisms.
Figures and Tables
Fig. 1
Representative RT-PCR product of each gene. The RT-PCR products from the transcripts of Bcl-2, caspase-3, C-6, VEGF, IL-6, and TNF-α were 177 bp, 100 bp, 145 bp, 97 bp, 161 bp, and 113 bp. RT-PCR: reverse transscription-polymerase chain reaction, Bcl: B cell leukemia/lymphoma, C: complement component, VEGF: vascular endothelial growth factor, IL: interleukin, TNF: tumor necrosis factor.
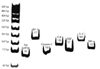
Fig. 2
Gene expressions of Bcl-2 (A), caspase-3 (B) in rat lung tissues after bosentan treatment. The gene expression of Bcl-2 mRNA was not significantly different after bosentan treatment (A). The expression of caspase-3 mRNA was significantly decreased after bosentan treatment in week 4 (B). *p<0.05 significantly different from C group, †p<0.05 significantly different from M group. Bcl: B cell leukemia/lymphoma, C: control group, M: monocrotaline group, B: bosentan group.
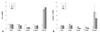
Fig. 3
Gene expressions of C-6 (A) and VEGF (B) in rat lung tissues after bosentan treatment. Expressions of C-6 (A) and VEGF mRNA (B) were significantly decreased after bosentan treatment in week 4. *p<0.05 significantly different from C group, †p<0.05 significantly different from M group. C-6: complement component, VEGF: vascular endothelial growth factor, C: control group, M: monocrotaline group, B: bosentan group.
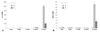
Fig. 4
Gene expressions of IL-6 and TNF-α in rat lung tissues after bosentan treatment. mRNA expression of IL-6 was significantly decreased in weeks 1, 2, and 4 after bosentan treatment (A). mRNA expressions of TNF-α had significantly decreased on day 5, in weeks 1 and 2 after bosentan treatment (B). *p<0.05 significantly different from C group, †p<0.05 significantly different from M group. mRNA: messenger ribonucleic acid, IL: interleukin, TNF: tumor necrosis factor, C: control group, M: monocrotaline group, B: bosentan group.
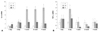
Fig. 5
Protein contents of C-6 (A) and VEGF (B) in rat lung tissues after bosentan treatment. C-6 and VEGF protein contents significantly decreased in the B group in week 4. *p<0.05 significantly different from C group, †p<0.05 significantly different from M group. C: complement component, VEGF: vascular endothelial growth factor, C: control group, M: monocrotaline group, B: bosentan group.
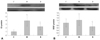
Fig. 6
Protein contents of IL-6 (A) and TNF-α (B) in rat lung tissues after bosentan treatment. IL-6 and TNF-α protein contents were significantly decreased in the B group in week 4. *p<0.05 significantly different from C group, †p<0.05 significantly different from M group. IL: interleukin, TNF: tumor necrosis factor, C: control group, M: MCT group, B: bosentan group.
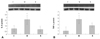
Fig. 7
TUNEL assay results in lung tissues. There were no TUNEL positive cells (green) in the C group. TUNEL positivity was progressively increased by MCT treatment. Copious cell death was evident in the M group compared with the C group. In contrast, apoptotic cell death in the B group was ameliorated than the M group. TUNEL: terminal deoxynucleotidyl transferase dUTP nick end labeling, C: control group, M: monocrotaline group, B: bosentan group.
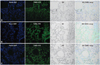
Table 1
Gene sequence of primer of reverse transcription polymerase chain reaction
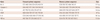
Acknowledgments
This work was supported by a National Research Foundation of Korea Grant (KRF-2009-0064784).
References
1. Gaine S. Pulmonary hypertension. JAMA. 2000; 284:3160–3168.
2. Jurasz P, Courtman D, Babaie S, Stewart DJ. Role of apoptosis in pulmonary hypertension: from experimental models to clinical trials. Pharmacol Ther. 2010; 126:1–8.
3. Denecker G, Vercammen D, Declercq W, Vandenabeele P. Apoptotic and necrotic cell death induced by death domain receptors. Cell Mol Life Sci. 2001; 58:356–370.
4. Kuwano K, Hara N. Signal transduction pathways of apoptosis and inflammation induced by the tumor necrosis factor receptor family. Am J Respir Cell Mol Biol. 2000; 22:147–149.
5. Sage E, Mercier O, Van den Eyden F, et al. Endothelial cell apoptosis in chronically obstructed and reperfused pulmonary artery. Respir Res. 2008; 9:19.
6. Budhiraja R, Tuder RM, Hassoun PM. Endothelial dysfunction in pulmonary hypertension. Circulation. 2004; 109:159–165.
7. Lévy M, Maurey C, Celermajer DS, et al. Impaired apoptosis of pulmonary endothelial cells is associated with intimal proliferation and irreversibility of pulmonary hypertension in congenital heart disease. J Am Coll Cardiol. 2007; 49:803–810.
8. Takahashi H, Goto N, Kojima Y, et al. Downregulation of type II bone morphogenetic protein receptor in hypoxic pulmonary hypertension. Am J Physiol Lung Cell Mol Physiol. 2006; 290:L450–L458.
9. Jamieson SW, Kapelanski DP, Sakakibara N, et al. Pulmonary endarterectomy: experience and lessons learned in 1,500 cases. Ann Thorac Surg. 2003; 76:1457–1462.
10. Hilliker KS, Roth RA. Increased vascular responsiveness in lungs of rats with pulmonary hypertension induced by monocrotaline pyrrole. Am Rev Respir Dis. 1985; 131:46–50.
11. Kido M, Hirose T, Tanaka K, Kurozumi T, Shoyama Y. Increased alveolarcapillary membrane permeability by monocrotaline. Jpn J Med. 1981; 20:170–179.
12. Sugita T, Stenmark KR, Wagner WW Jr, et al. Abnormal alveolar cells in monocrotaline induced pulmonary hypertension. Exp Lung Res. 1983; 5:201–215.
13. Rosenberg HC, Rabinovitch M. Endothelial injury and vascular reactivity in monocrotaline pulmonary hypertension. Am J Physiol. 1988; 255:H1484–H1491.
14. Wilson DW, Lamé MW, Dunston SK, Segall HJ. DNA damage cell checkpoint activities are altered in monocrotaline pyrrole-induced cell cycle arrest in human pulmonary artery endothelial cells. Toxicol Appl Pharmacol. 2000; 166:69–80.
15. Lim KA, Kim KC, Cho MS, Lee BE, Kim HS, Hong YM. Gene expression of endothelin-1 and endothelin receptor a on monocrotaline-induced pulmonary hypertension in rats after bosentan treatment. Korean Circ J. 2010; 40:459–464.
16. Koo HS, Kim KC, Hong YM. Gene expressions of nitric oxide synthase and matrix metalloproteinase-2 in monocrotaline-induced pulmonary hypertension in rats after bosentan treatment. Korean Circ J. 2011; 41:83–90.
17. Stier S, Totzke G, Gruewald E, et al. Identification of p54nrb and the 14-3-3 Protein HS1 as TNF-alpha-inducible genes related to cell cycle control and apoptosis in human arterial endothelial cells. J Biochem Mol Biol. 2005; 38:447–456.
18. Sakao S, Taraseviciene-Stewart L, Lee JD, Wood K, Cool CD, Voelkel NF. Initial apoptosis is followed by increased proliferation of apoptosis-resistant endothelial cells. FASEB J. 2005; 19:1178–1180.
19. Matter CM, Chadjichristos CE, Meier P, et al. Role of endogenous Fas (CD95/Apo-1) ligand in balloon-induced apoptosis, inflammation, and neointima formation. Circulation. 2006; 113:1879–1887.
20. Partovian C, Adnot S, Eddahibi S, et al. Heart and lung VEGF mRNA expression in rats with monocrotaline- or hypoxia-induced pulmonary hypertension. Am J Physiol. 1998; 275:H1948–H1956.
21. Alavi A, Hood JD, Frausto R, Stupack DG, Cheresh DA. Role of Raf in vascular protection from distinct apoptotic stimuli. Science. 2003; 301:94–96.
22. VEGF Inhibition Study in Ocular Neovascularization (V.I.S.I.O.N.) Clinical Trial Group. D'Amico DJ, Masonson HN, et al. Pegaptanib sodium for neovascular age-related macular degeneration: two-year safety results of the two prospective, multicenter, controlled clinical trials. Ophthalmology. 2006; 113:992–1001.
23. Jankov RP, Kantores C, Belcastro R, Yi M, Tanswell AK. Endothelin-1 inhibits apoptosis of pulmonary arterial smooth muscle in the neonatal rat. Pediatr Res. 2006; 60:245–251.
24. Hahn AW, Resink TJ, Scott-Burden T, Powell J, Dohi Y, Bühler FR. Stimulation of endothelin mRNA and secretion in rat vascular smooth muscle cells: a novel autocrine function. Cell Regul. 1990; 1:649–659.
25. Giaid A, Yanagisawa M, Langleben D, et al. Expression of endothelin-1 in the lungs of patients with pulmonary hypertension. N Engl J Med. 1993; 328:1732–1739.
26. Jasmin JF, Lucas M, Cernacek P, Dupuis J. Effectiveness of a nonselective ET(A/B) and a selective ET(A) antagonist in rats with monocrotaline-induced pulmonary hypertension. Circulation. 2001; 103:314–318.
27. Jankov RP, Luo X, Belcastro R, et al. Gadolinium chloride inhibits pulmonary macrophage influx and prevents O2-induced pulmonary hypertension in the neonatal rat. Pediatr Res. 2001; 50:172–183.
28. Rabinovitch M. Molecular pathogenesis of pulmonary arterial hypertension. J Clin Invest. 2008; 118:2372–2379.
29. Soon E, Holmes AM, Treacy CM, et al. Elevated levels of inflammatory cytokines predict survival in idiopathic and familial pulmonary arterial hypertension. Circulation. 2010; 122:920–927.
30. Price LC, Wort SJ, Perros F, et al. Inflammation in pulmonary arterial hypertension. Chest. 2012; 141:210–221.