Abstract
Significant improvement in survival of children with congenital cardiac malformations has resulted in an increasing population of adolescent and adult patients with congenital heart disease. Of the long-term cardiac problems, ventricular dysfunction remains an important issue of concern. Despite corrective or palliative repair of congenital heart lesions, the right ventricle, which may be the subpulmonary or systemic ventricular chamber, and the functional single ventricle are particularly vulnerable to functional impairment. Regular assessment of cardiac function constitutes an important aspect in the long-term follow up of patients with congenital heart disease. Echocardiography remains the most useful imaging modality for longitudinal monitoring of cardiac function. Conventional echocardiographic assessment has focused primarily on quantification of changes in ventricular size and blood flow velocities during the cardiac cycles. Advances in echocardiographic technologies including tissue Doppler imaging and speckle tracking echocardiography have enabled direct interrogation of myocardial deformation. In this review, the issues of ventricular dysfunction in congenital heart disease, conventional echocardiographic and novel myocardial deformation imaging techniques, and clinical applications of these techniques in the functional assessment of congenital heart disease are discussed.
Advances in diagnosis, catheter and surgical interventions, and intensive care have significantly improved the survival of children with congenital cardiac malformations. In the present era, 85% or more of children born with congenital heart diseases are expected to survive into adulthood.1) The significantly improved survival has resulted in an increasing population of adolescent and adult patients with congenital heart disease who are confronted with unique cardiac and non-cardiac issues.1)2) Of the long-term cardiac problems, ventricular dysfunction remains an important issue of concern. Despite corrective or palliative repair of congenital heart lesions, the right ventricle, which may be the subpulmonary or systemic ventricular chamber, and the functional single ventricle are particularly vulnerable to functional impairment.
Regular assessment of cardiac function constitutes an important aspect in the long-term follow up of patients with congenital heart disease. Given its non-invasiveness and widespread availability, echocardiography remains the most useful imaging modality for longitudinal monitoring of cardiac function. While conventional echocardiographic assessment of cardiac function has focused on quantification of changes in ventricular size and blood flow velocities during the cardiac cycles, advances in echocardiographic technologies have enabled direct evaluation of myocardial deformation. In the recent few years, novel myocardial deformation imaging has found increasing applications in functional assessment of congenital heart disease. In this review, the issues of ventricular dysfunction in congenital heart disease, conventional echocardiographic and novel myocardial deformation imaging techniques, and clinical applications of these techniques in the functional assessment of congenital heart disease are discussed.
Transannular patch repair is commonly required during surgical repair of tetralogy of Fallot (TOF), as the pulmonary valve annulus is usually small. While initially thought to be a relatively benign condition, chronic pulmonary regurgitation with consequential volume overloading of the subpulmonary right ventricle is now recognized as one of the most important determinants of late outcome.3), 4), 5) Development of right ventricular (RV) systolic and diastolic dysfunction in association with chronic severe pulmonary regurgitation is well documented.6)7) Aneurysmal dilation and akinesia of the RV outflow contribute further to RV dilation and detrimentally affect RV ejection fraction.8) Development of right bundle branch block and QRS prolongation after surgery may potentially lead to RV mechanical dyssynchrony.9) All of these factors may act synergistically and contribute to progressive RV dysfunction late after TOF repair, with the clinical consequences of exercise intolerance, development of cardiac arrhythmias, and congestive heart failure.10), 11), 12)
The phenomenon of restrictive RV physiology occurs both early and late after surgical repair of TOF, which is a manifestation of abnormal relaxation and probably reduced compliance of the subpulmonary right ventricle. During early postoperative period, restrictive RV physiology may account for low cardiac output syndrome.13) On the other hand, persistence of restrictive RV physiology in the long-term has been associated with less progressive dilation of the right ventricle and better exercise capacity.14) Restrictive RV physiology has also been described after biventricular repair of pulmonary atresia with intact ventricular septum (PAIVS) due probably to the relatively small RV cavity and intrinsic myocardial and endocardial abnormalities that reduce RV compliance.15) Limited data also suggest that restrictive RV physiology also predicts better exercise capacity in these patients.15)
In congenitally corrected transposition of the great arteries (cc-TGA) and in patients with complete transposition of the great arteries (TGA) after atrial repair, the right ventricle acts as the systemic pumping chamber.
Systemic RV dysfunction occurs with increasing prevalence with advancing age in cc-TGA. It is estimated that about half of the patients may develop RV dysfunction and congestive heart failure by the fifth decade, even in the absence of significant associated cardiac lesions.16) Occurrence of systemic RV dysfunction in cc-TGA is usually accompanied by progressive RV dilation and worsening of tricuspid regurgitation.17) Notwithstanding the 'chicken and egg' controversy, it appears that the intrinsic architectural design of the tricuspid valve and geometry of the right ventricle may render them more vulnerable to progressive failure.18) Dilation of the systemic right ventricle may set up a vicious cycle by shifting the ventricular septum to the left side, pulling the tricuspid septal leaflet for from the other tricuspid leaflets, which aggravates preexisting tricuspid regurgitation. This hypothesis is supported by the observation of amelioration of tricuspid regurgitation with placement of a pulmonary arterial band,19)20) which increases subpulmonary left ventricular (LV) pressure and restores, albeit partially, the septal geometry. Single-chamber ventricular pacing in patients with cc-TGA and complete heart block may further aggravate systemic RV failure possibly by inducing mechanical dyssynchrony.21)
Substrates other than tricuspid valve design and RV geometry may predispose to development of systemic RV dysfunction in patients after the Senning or Mustard procedure. Myocardial perfusion defects and impaired flow reserve involving the systemic right ventricle have been found in survivors of the Mustard operation,22)23) which may suggest inadequate coronary arterial supply to the hypertrophic RV myocardium to cope with the systemic arterial load. Studies using late gadolinium enhancement (LGE) and equilibrium contrast cardiac magnetic resonance (CMR) have shown areas of fibrosis,24)25) the extent of which has been found to correlate with RV dilation and impairment of systolic function. Reduction of systemic RV ejection fraction and long-axis function has been demonstrated in patients with TGA after atrial repair and may suggest systolic dysfunction.26), 27), 28) Nonetheless, whether this represents intrinsic RV failure or an adaptive response to increased systemic afterload is contentious. On the other hand, accumulating data suggest that impairment of atrioventricular coupling due to restrictive atrial baffling pathways may limit the augmentation of stroke volume during exercise and other forms of stress, and constitute a unique form of diastolic dysfunction of the systemic right ventricle.29)
The function of the right and left ventricles is closely linked given the enclosure of the two ventricles within the same pericardial cavity, sharing of ventricular septum, and coursing of superficial epicardial myocardial fibres through two ventricles. These anatomic substrates provide the basis of adverse ventricular-ventricular interaction in patients with congenital heart disease with abnormal RV geometry and function.
In repaired TOF patients, impaired LV function has been related to abnormal RV function and shown to be a significant independent risk factor for long-term adverse outcomes. Suboptimal RV-LV diastolic interaction secondary to RV dilation and septal shift with consequential reduction of LV compliance and diastolic filling has been described.30)31) With interrogation of LV mechanics by deformation imaging techniques, abnormal systolic ventricular-ventricular interaction after TOF repair is also being increasingly recognized. Additionally, limited data suggest the presence of adverse systemic RVsubpulmonary LV interaction in patients after atrial repair. These unfavourable ventricular-ventricular interactions are discussed below.
Ventricular dysfunction remains one of the most important longterm complications in patients with functionally univentricular hearts palliated by Fontan-type procedures.32) Intrinsic abnormalities of myocardial architecture are found in functional single ventricles.33)34) Pressure and volume loading of the systemic ventricle occurs as a result of the parallel supply of the systemic and pulmonary circulation prior to univentricular repair. The volume overload may be further aggravated by atrioventricular valvar insufficiency, which may be related to dysplasia of the common atrioventricular valve or annular dilation secondary to ventricular dilation. Conversion from parallel systemic and pulmonary circulation to the in-series Fontan circulation is associated with changes in ventricular geometry, acquired ventricular hypertrophy, and preload reduction. The consequences of incoordinate ventricular relaxation, diastolic ventricular dysfunction, and probably progressive reduction in ventricular compliance have been recognized.35)36) Furthermore, increased systemic afterload with abnormal ventriculo-arterial interaction found in post Fontan patients may have further negative impact on diastolic function of the functional single ventricle.37)
Conventional echocardiographic assessment of cardiac function in patients with congenital heart disease has focused on quantification of changes in ventricular dimensions and chamber volumes for assessment of systolic function, and Doppler-derived blood flow velocities across atrioventricular valves for evaluation of diastolic function.
M-mode has conventionally been used to derive LV shortening fraction for assessing global LV systolic function. Shortening fraction is affected by loading conditions, regional myocardial abnormalities, and alteration of LV geometry. Existence of paradoxical septal motion in RV volume- and pressure-loaded conditions invalidates the interpretation of this LV functional parameter. Furthermore, this geometric parameter is of little use in the assessment of RV and single ventricular systolic function.
Fractional area change of the right ventricle determined by two-dimensional (2D) planimetry has also been used as an index of RV systolic function in adults,38) although this has not been validated in children. To assess RV longitudinal shortening as a measure of RV systolic function, placement of the M-mode curser through the tricuspid annulus in the four-chamber view enables the derivation of the so-called tricuspid annular plane systolic excursion (TAPSE). TAPSE has been used to evaluate subpulmonary and systemic RV systolic function, although conclusions on its usefulness are mixed.39), 40), 41), 42) Normal reference values of TAPSE in children are available to facilitate the interpretation of patient data.43)
Cardiac magnetic resonance is regarded as the gold standard in the measurement of ventricular volumes and quantification of ejection fraction. Avoidance of the need for geometric assumption is of particular importance in the evaluation of right ventricles, functional single ventricles, and distorted left ventricles due to septal shift in patients with congenital heart disease.
Different 2D echocardiographic methods including the Simpson algorithms, area-length method, and bullet method have been used to estimate LV volumes.44) Nonetheless, violation of geometric assumptions is not uncommon in patients with congenital heart disease, which limits their accuracy and usefulness in the clinical setting. Although different formulae have also been proposed to estimate RV volume by 2D echocardiography, there are little or no data regarding their utility and accuracy in children.44)
The introduction of real-time three-dimensional (3D) echocardiography has shown great promise in evaluating ventricular volumes regardless of ventricular geometry (Fig. 1). Its initial applications in children are encouraging. Importantly, LV and RV volumes and volumes of functional single ventricles obtained by real-time 3D echocardiography compare favorably with those obtained using CMR.45)46) Hence, real-time 3D echocardiography has an important advantage in evaluating volume-based functional parameters in patients with congenital heart disease having ventricles of different geometries.
Pulsed-wave Doppler analysis of mitral early (E) and late (A) diastolic inflow velocities and E-deceleration time is commonly used to assess LV diastolic function. In children with fast heart rates, the utility of these indices may however by limited due to frequent fusion of the E and A waves. Isovolumic relaxation time, another parameter of LV diastolic function, can be measured by simultaneous continuous-wave Doppler interrogation of LV inflow and outflow.
Respiratory variations of tricuspid valve inflow velocities render their measurement difficult in children. Reduction of RV compliance in certain congenital heart lesions further confounds the interpretation of the tricuspid inflow velocities. A good surrogate pulsed-Doppler measure of impaired RV diastolic compliance is the antegrade diastolic flow in the pulmonary artery coincident with atrial systole (Fig. 2).13)
The spectral Doppler-derived myocardial performance index (MPI) can be used to assess combined systolic and diastolic function of the left and right ventricles.47) It is calculated as the sum of isovolumic relaxation time and isovolumic contraction time divided by ejection time. The MPI has increasingly been utilized in the assessment of global RV function in patients with different types of congenital heart lesions.44)
Velocities of myocardial tissue motion can be determined using tissue Doppler imaging.48) Nonetheless, tissue Doppler imaging allows only one-dimensional assessment of the tissue regional velocity vector along the beam of the ultrasound. Hence, commonly measured myocardial velocities are those at the base and mid-levels of the LV lateral wall, ventricular septum, and RV free wall determined from the four-chamber view. Increasingly, tissue Doppler evaluation of the mitral and tricuspid annular junction is utilized to assess respective LV and RV function in children with congenital heart diseases.
From the spectral or colour tissue Doppler tracings, the peak systolic and two diastolic peak velocities can be measured (Fig. 3). The positive systolic peak represents annular motion towards the apex and reflects ventricular systolic function. The early negative diastolic annular velocity (e') reflects ventricular recoil from the contracted state. The late negative diastolic peak represents annular motion during atrial contraction and is affected by ventricular diastolic and atrial systolic function.
An additional peak velocity is seen during isovolumic contraction. A relatively-load independent index, isovolumic acceleration during myocardial acceleration (IVA), can be calculated as the difference between peak and baseline myocardial velocities divided by the time interval from onset of the wave during isovolumic contraction at zero-crossing to the time at peak velocity.49)
The E/e' ratios on the respective sides may provide an estimation of LV and RV filling pressures,50)51) although pediatric validation is lacking. The MPI can also be determined by measuring the time intervals on spectral tissue Doppler tracings, which correlates well with that derived from conventional Doppler interrogation.52)
Conventional echocardiographic assessment of cardiac function based on evaluation of changes in cardiac dimensions and blood flow can perhaps be regarded only as an indirect assessment of cardiac function consequential upon shortening and lengthening of myocardium throughout the cardiac cycle. Techniques that allow direct interrogation of myocardial mechanics may be more sensitive in the detection of subtle preclinical functional impairment.
The heart undergoes complex systolic and diastolic deformation in three dimensions in relation to the architectural design of the myocardium. The different aspects of myocardial deformation can broadly be described in terms of linear deformation, torsional deformation, and synchronicity of deformation.
Linear deformation of the myocardium can be quantified by strain. Strain is defined as the percentage change in the length of myocardial segment relative to the reference length taken usually at end-diastole. Linear deformation of the left ventricle is usually described in terms of strain along its three principle geometrical coordinates: longitudinal, circumferential, and radial. As the RV myocardial fibres are arranged longitudinally, longitudinal strain is usually used to describe RV deformation.
The speed of myocardial deformation, on the other hand is quantified by strain rate. Peak systolic strain rate, compared with systolic strain, might better reflect the rate of myocardial force generation and hence cardiac contractility.53)54) Diastolic strain rates have been used to assess ventricular diastolic function.55)
The helical arrangement of myocardial fibres accounts for the torsional deformation of the left ventricle.56) Anti-clockwise rotation of the left heart, as viewed from the cardiac apex, occurs during isovolumic contraction. During ventricular ejection, the apex continues its anti-clockwise rotation while the base rotates in the clockwise direction.57) Untwisting with clockwise rotation of the apex occurs during relaxation to generate a suction force that draws blood into the LV from the left atrium.58) Torsional deformation of the left ventricle contributes significantly to LV diastolic recoil and function.59) Recent data further suggest the possibility of ventricular torsion as an aid to ejection.60) But, the phenomenon of RV torsion is poorly understood.
Dyssynchrony of myocardial deformation refers to the incoordinate contraction of regional myocardial segments. This may be related to electrical conduction delay, abnormalities in excitation-contraction coupling, or abnormal myocardial tissue leading to regional delay in the onset of myocardial shortening.61) Incoordinate contraction of the early- and late-activated segments leads to energy loss, delays the rise of intra-ventricular cavity pressure, and reduces cardiac output. In congenital heart diseases, pathological and electrical substrates for development of dyssynchrony may potentially be present. Although data in congenital heart disease literature on the aetiology and prevalence are lacking, there is increasing interest in the application of cardiac resynchronization therapy in the management of patients with congenital heart disease complicated by ventricular failure.62)63)
Whereas spectral Doppler acquisition enables only direct measurement of peak velocities and time intervals as aforementioned, color Doppler data acquired at a frame rate of 100 frames per second or more can be used to derive regional myocardial deformation parameters.64)65) Strain rate is numerically identical to the spatial gradient tissue velocity, while strain can be derived as the temporal integral of strain rate.
While a major strength of tissue Doppler strain imaging is its ability to quantify regional myocardial mechanics, this technique suffers from several major limitations. These include angle dependency, noisy signals with resultant poor quality strain and strain rate curves, and assessment of limited number of myocardial segments that are parallel to the direction of ultrasound beam. These limitations negate the global assessment of cardiac function.
Two-dimensional speckle tracking echocardiography is a relatively new and largely angle-independent technique for imaging myocardial deformation66)67) and has increasingly been applied to assess cardiac function in patients with congenital heart disease. The principle of this technique is utilization of a template matching algorithm to track the motion of clusters of speckles in 2D grey scale images frame by frame. Speckles are natural acoustic markers generated from constructive and destructive interference of ultrasound backscattered from tissues. Acquisition of grey scale images at a frame rate of 40 to 80 per second provides spatial and temporal information for quantification of strain, strain rate, and ventricular twist and torsion.
Deformation imaging of both the left and right ventricles is feasible with 2D speckle tracking echocardiography (Fig. 4). Hence, global and regional LV and RV longitudinal deformation can be determined from the apical four-chamber view, while LV circumferential and radial deformation can be determined from the mid parasternal short axis view, and torsional mechanics from the apical and basal short axis planes.68)69)70)71)
Although 2D speckle tracking echocardiography has overcome some of the important limitations of tissue Doppler imaging, shortcomings inherent to its 2D nature are inevitable. Speckles that move out of the 2D plane cannot be tracked. Furthermore, separate basal and apical acquisitions for assessment for torsional mechanics.
The recently developed 3D speckle tracking echocardiography tracks the 3D motion of speckles within the acquired 3D dataset. This new deformational imaging modality enables the assessment of truly 3D global and regional strain (Fig. 5).72) An additional innovative feature is the ability to track the endocardial surface area change, so-called area strain, throughout the cardiac cycle.73)74) This can be regarded as a composite parameter that integrates the regional consequence of deformation in the longitudinal, circumferential, and radial dimensions. Furthermore, this modality allows more efficient data acquisition and simultaneous analysis of all the LV segments. A limitation, however, is the relatively low volume frame rate at 20 to 30 volumes per second. Nonetheless, a recent study showed that a frame rate of 18 or 25 volumes per second does not appear to compromise the assessment of myocardial deformation.75) Indeed, this new technology has found increasing applications in a variety of clinical scenarios for assessment of global LV performance, quantification of regional function, and evaluation of cardiac resynchronization. Recently, 3D speckle tracking echocardiography has been utilized to evaluate RV mechanics in adults with pulmonary hypertension.76)
Several echocardiographic approaches may be used to assess ventricular mechanical dyssynchrony.62)63)69)77) M-mode can be used to assess LV dyssynchrony by measuring the septal-to-posterior wall motion delay. Other modalities allow the assessment of LV and RV dyssynchrony. Pulsed-wave and color-coded tissue Doppler imaging can provide myocardial velocity tracings for determination of difference in timing of peak systolic velocities among different myocardial segments within the same or different ventricles for calculation of intra- and inter-ventricular dyssynchrony.
Myocardial strain imaging by tissue Doppler imaging or 2D/3D speckle tracking echocardiography can also be used to determine differences in timing of peak systolic strain among ventricular segments (Fig. 6).72)78) Real-time 3D echocardiography can also be used to derive a LV systolic dyssynchrony index based on the standard deviation of time taken to reach minimum regional volume for each of the LV segments (Fig. 1).79)
Assessment of RV volumes and ejection fraction is important for determining the timing of pulmonary valve replacement in patients with repaired TOF. The European Society of Cardiology,2) American College of Cardiology/American Heart Association,1) and Canadian Cardiovascular Society80) guidelines recommend that pulmonary valve replacement should be considered even in asymptomatic patients with moderate-to-severe degree of RV dilation, evidence of progressive RV dilation, and RV systolic dysfunction.
Using tissue Doppler imaging, Weidemann et al.81) found significant reduction of myocardial velocities, systolic strain, and systolic strain rate of RV free wall, LV lateral wall, and ventricular septum. They further found that abnormalities in RV deformation were more marked in patients with a transannular patch than those with an infundibular patch. Frigiola et al.6) further reported that a lower RV IVA in children and adults with repaired TOF was associated with more severe pulmonary regurgitation and longer QRS duration, suggesting that reduced RV contractile function is related to the degree of pulmonary regurgitation and that RV IVA may be an early sensitive index for selecting patients for pulmonary valve replacement. Decreased RV systolic strain and strain rate have been associated with worse RV mechanical dyssynchrony and decreased exercise capacity.82) Intra-RV and interventricular mechanical dyssynchrony has further been documented in these patients, which becomes worse during exercise.83)
The clinical relevance of restrictive RV physiology in TOF patients has been alluded to earlier. Tissue Doppler imaging studies have also provided evidence of RV and LV diastolic dysfunction.84) With the coexistence of systolic and diastolic RV dysfunction, the documented increase in RV MPI in repaired TOF patients is perhaps expected.85)86)87) Importantly, RV MPI correlates negatively with RV ejection fraction and exercise capacity in these patients.86)
Deformation imaging has shed light on the understanding of adverse systolic ventricular-ventricular interaction in patients after TOF repair. Reduced LV systolic longitudinal, radial, and circumferential strain and diastolic circumferential and radial strain rate has been reported.70)84) Additionally, the magnitude of RV dilation has been correlated negatively with LV systolic circumferential strain and strain rate, and LV mechanical dyssynchrony.70) The role of altered septal geometry secondary to RV volume overload in adverse RV-LV interaction in repaired TOF patients is increasingly recognized. Eccentricity of the left ventricle due to septal shift correlates negatively with LV peak systolic torsion, systolic twisting velocity, and diastolic untwisting velocity.88)89) A recent 3D speckle tracking echocardiographic study showed that reduced septal curvature is related to impairment of LV 3D systolic strain, mechanical dyssynchrony, and reduced ventricular torsion in repaired TOF patients with and without pulmonary valve replacement.90) The new 3D technique has enabled comprehensive on stop-shop evaluation of LV global performance by taking simultaneously into account the different aspects of deformation.74)90)
Limited data exist on the impact of pulmonary valve replacement on ventricular performance. Acutely after transcatheter pulmonary valve implantation in a cohort of children including those with repaired TOF, improvement in RV free wall strain has been noted.91) Transcatheter pulmonary valve replacement in patients with repaired TOF and other congenital heart diseases has also resulted in improvement of LV circumferential and longitudinal strain and longitudinal dyssynchrony.92)
Recently, Diller et al.93) described the potential prognostic value of deformation imaging in repaired TOF patients. In 413 adults patients studied at a mean of 36 years and followed up for a median of 2.9 years, mitral annular plane systolic excursion and LV global longitudinal 2D strain were related to the composite endpoint of sudden cardiac death or life-threatening ventricular arrhythmias independent of QRS duration.
The understanding of RV mechanics after biventricular repair of PAIVS is limited. A tissue Doppler echocardiographic study has shown regional systolic and diastolic longitudinal myocardial dysfunction involving both the right and left ventricles in adolescent patients after biventricular repair in infancy.94) A subsequent study using 2D speckle tracking imaging confirmed the impairment of RV systolic and diastolic deformation.15) LGE CMR showed evidence of RV myocardial fibrosis. About 80% of these patients have a restrictive RV physiology, which is related to more severe RV fibrosis and better exercise capacity in the long-term.15)
The consistently reported reduction of systemic RV ejection fraction in patients with TGA after atrial repair and the controversy regarding its interpretation has been discussed previously. Nonetheless, accumulating functional data based on deformation imaging suggest the possibility of impaired systemic RV mechanics, which may have prognostic implications in these patients.
Tissue Doppler imaging revealed significantly lower regional systolic strain and strain rate of RV free wall, septum, and LV lateral wall in patients after the Senning procedure.95)96) Significant correlation was found between regional longitudinal RV systolic strain parameters and RV ejection fraction. Vogel et al.97) validated the use of IVA in assessing systemic RV contractile function. Abnormal wall motion in these patients after atrial repair was associated with reduced IVA. Furthermore, reduced contractile reserve of the systemic right ventricle as assessed by changes in IVA with dobutamine infusion was associated with increased brain natriuretic peptide levels.98) Indeed, plasma brain natriuretic peptide has been positively correlated with systemic RV MPI and negatively correlated with systemic RV free wall and septal annular myocardial velocities.99)
Chow et al.69) demonstrated the reproducibility and potential usefulness of 2D speckle tracking echocardiography in assessing systemic RV function. Global systemic RV longitudinal strain and strain rate has been correlated with CMR-derived systemic RV ejection fraction. These strain parameters were also found to correlate with systemic RV IVA and MPI. Altered systemic RV contraction and deformation pattern has further been unveiled by strain imaging. Using CMR, Pettersen et al.100) found predominant circumferential over longitudinal free wall shortening in the systemic right ventricle as in the normal left ventricle, which is opposite from the findings in the normal subpulmonary right ventricle. However, unlike the normal LV, the systemic RV did not exhibit torsional deformation.
Cardiac resynchronization therapy improves systemic RV function in patients after atrial repair.62)63) Strain imaging has helped define intra-systemic RV and inter-ventricular mechanical delay in these patients. Using tissue Doppler strain imaging, Chow et al.101) reported a prevalence of 32% and 57% for intra-systemic RV dyssynchrony and inter-ventricular dyssynchrony, respectively. Importantly, the intra- and inter-ventricular mechanical delays negatively affect the systemic RV ejection fraction and exercise capacity. Although the exact cause is unknown, non-uniform alteration of RV myocardium due to ischaemia and fibrotic process might contribute to asynchronous myocardial deformation.22)23)24)25)
Studies on ventricular-ventricular interaction in patients after atrial repair are limited. Unfavorable diastolic ventricular interaction was suggested by a 2D specking tracking study. In both the systemic right ventricle and subpulmonary left ventricle, early and late diastolic strain rates were significantly reduced. Subpulmonary LV eccentricity index, a reflection of septal shift (Fig. 7), correlated negatively with LV early and late diastolic strain rates.102) For systolic interaction, Diller et al.103) reported correlations between systemic RV and subpulmonary LV 2D longitudinal strain and CMR-derived ejection fraction in patients after atrial repair and those with cc-TGA. Furthermore, they103) and others41) showed that systemic RV global longitudinal strain predicts adverse clinical outcomes in these patients in terms of symptomatic progression towards worse functional class, development of cardiac arrhythmias, and death.
Similar to patients after atrial switch operation, patients with cc-TGA have reduced systemic RV peak systolic strain and strain rate.104) A direct piece of evidence of ventricular-ventricular interaction in this setting can be appreciated in these patients and in those with TGA post atrial switch with failing systemic right ventricle undergoing pulmonary arterial banding.19)20) The consequent increase in subpulmonary LV load may partially reverse the septal shift and improve systemic RV geometry with reduction in tricuspid regurgitation.
The placement of the pulmonary arterial band may, however, cause acute reduction in global subpulmonary LV strain and ejection fraction. Even after anatomic repair with LV retraining, the systemic left ventricle has been shown to demonstrate further reduction in the global LV strain,105) implying the need for long-term evaluation of myocardial mechanics in these patients. Whether deformation assessment may be used to stratify patients with a systemic right ventricle eligible for anatomical repair remain to be clarified.
Complete heart block may complicate cc-TGA. Pacemaker therapy has been identified as an important risk factor for development of systemic RV dysfunction. Limited tissue Doppler imaging data suggest the possibility of pacing-induced systemic RV dyssynchrony being the culprit, which may be rectified by biventricular pacing.21)
Although previous studies have reported on normal resting LV systolic function as assessed by M-mode echocardiography in patients with TGA after arterial switch operation,106)107) Pettersen et al.108) showed, using 2D speckle tracking echocardiography, reduction of LV global longitudinal strain and torsion. In children studied about 9 years after arterial switch operation, Hui et al.109) demonstrated dobutamine stress induced abnormalities of LV segmental wall motion, whose location corresponded to regions of impaired myocardial perfusion. A recent study further showed impaired LV contractile reserve as evidenced by flattening of the change of LV IVA with increase in heart rate, which was worse in patients with variant coronary arterial anatomy and might have implications on their exercise capacity.110) Additionally, reduction of diastolic mitral annular velocities suggestive of LV diastolic dysfunction was found in this study. Hence, continued monitoring of systemic LV function after arterial switch operation is warranted.
Systolic and diastolic ventricular dysfunction in patients with a functional single ventricle post Fontan-type procedures has been documented.32) In Fontan patients with tricuspid atresia, Ho et al.111) demonstrated using 2D speckle tracking echocardiography reduction of global systemic LV longitudinal, circumferential, and radial strain. Furthermore, the findings of reduced systolic and diastolic strain rates reflect subclinical systolic and diastolic ventricular dysfunction, which can be attributed to chronic volume and pressure overload before the Fontan procedure, and reduced preload, acquired wall thickening, uncoordinated ventricular relaxation, and increase afterload after the operation. The speckle tracking algorithm as applied to CMR has revealed altered torsional mechanics in patients with functional single ventricles of LV or indeterminate morphologies after cavopulmonary connection.112)
Potential differences in single RV and LV function have also been investigated at different stages of univentricular repair by deformation imaging. Kaneko et al.113) showed that single right ventricle had the worst systolic performance in before cavopulmonary anastomosis, although parity with single LV function was regained after Fontan-type procedures. Indeed, Cheung et al.114) have shown that while functional single ventricles exhibit reduced ventricular contractile reserve as assessed by tissue Doppler-derived myocardial force-frequency relationship, no differences in contractile function was found between functional single ventricles with RV and those with LV morphology. Adaption of RV deformation in hypoplastic left heart syndrome with reduction of RV longitudinal to circumferential strain ratio before cavopulmonary anastomosis is associated with better contractile dyssychrony.115)
Limited data suggest dyssynchronous myocardial deformation of functional single ventricles. In children with hypoplastic left heart syndrome, Friedberg et al.116) demonstrated mechanical dyssynchrony of the systemic right ventricle using velocity vector imaging based on the principle of 2D speckle tracking. Earlier CMR studies showed regional LV wall motion abnormalities in patients with tricuspid atresia after the Fontan procedure.117)118) Based on 3D segmental volume assessment of the systemic left ventricle, Ho et al.111) documented a 55% prevalence of mechanical dyssynchrony in Fontan patients with tricuspid atresia. This study also demonstrated a positive correlation between severity of mechanical dyssynchrony and calibrated integrated backscatter. This finding together with the reported association between LGE and regional wall motion abnormality and dyskinesia in Fontan patients119) suggest that myocardial fibrosis may play a role in the origin of asynchronous myocardial deformation in function single ventricles.
Limitations of the various techniques have to be taken into account when translating these modalities into clinical use. The angle dependency of Doppler-based techniques, the varying load dependency of different parameters, and the relatively low temporal resolution of current 3D techniques are of particular relevance when assessing cardiac function in congenital heart disease. To facilitate the clinical applications of the novel techniques, establishment of normal references for different parameters in different age groups is necessary.120)121)122) Discordance in deformation measurements among manufactures has to be resolved.123)124)125)
Functional assessment of cardiac function in congenital heart diseases, in particular RV function and function of a single ventricle, has been facilitated by the introduction of novel echocardiographic techniques that enable the quantification of ventricular volumes independent of ventricular geometry and direct evaluation of myocardial global and regional motion and deformation. These new techniques show great potential in the comprehensive functional evaluation of patients with congenital heart disease at risk of late ventricular dysfunction and cardiac failure. Furthermore, the functional impact of medical, device, and surgical interventions can be ascertained more objectively. The ability to directly interrogate myocardial function may allow early sensitive detection of subclinical myocardial dysfunction, better risk stratification, and timely institution of interventions. Large scale longitudinal studies are nonetheless required to substantiate the clinical values of these novel functional parameters in the management, risk stratification, and prognostication of different congenital heart diseases.
Figures and Tables
Fig. 1
Real-time three-dimensional echocardiographic analysis of left ventricular (LV) and right ventricular (RV) volumes. The regional time-volume curves of the 16 LV segments enable the assessment of LV mechanical dyssynchrony.
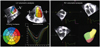
Fig. 2
Pulsed-Doppler echocardiography demonstrating antegrade diastolic flow in the main pulmonary artery during atrial systole.
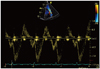
Fig. 3
Tissue Doppler imaging with the curser at the right ventricular free wall-tricuspid annular junction. a: late diastolic myocardial velocity, e: early diastolic myocardial velocity, IVA: isovolumic acceleration, s: systolic myocardial velocity, Δt: time difference, Δv: velocity change.
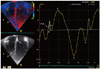
Fig. 4
Two-dimensional speckle tracking echocardiography demonstrating the assessment of global left ventricular (LV) and right ventricular (RV) longitudinal strain from the four-chamber view, and LV circumferential and radial strain from the mid-LV short axis.
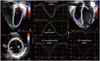
Fig. 5
Quantification of three-dimensional (3D) strain based on 3D speckle tracking echocardiographic assessment of the full volume 3D dataset acquired from the cardiac apex.
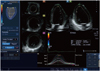
Fig. 6
Tissue Doppler strain imaging showing systemic right ventricular dyssynchrony in a patient after atrial switch operation for transposition of the great arteries as evidenced by differences of time to peak systolic strain right ventricular free wall and septal segments (A) compared to the similar timing in a healthy subject (B).
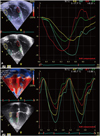
Fig. 7
Left panel: normal septal geometry in the healthy subject and distortion of septal geometry with compression of the subpulmonary left ventricle in a patient after atrial repair for complete transposition of the great arteries. Right panel: two-dimensional speckle tracking echocardiography enables deformation imaging of the subpulmonary left ventricle in patient as compared to the systemic left ventricle in a normal subject. TGA: transposition of the great arteries.
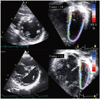
References
1. Warnes CA, Williams RG, Bashore TM, et al. ACC/AHA 2008 guidelines for the management of adults with congenital heart disease: a report of the American College of Cardiology/American Heart Association Task Force on Practice Guidelines (Writing Committee to Develop Guidelines on the Management of Adults With Congenital Heart Disease). Developed in Collaboration With the American Society of Echocardiography, Heart Rhythm Society, International Society for Adult Congenital Heart Disease, Society for Cardiovascular Angiography and Interventions, and Society of Thoracic Surgeons. J Am Coll Cardiol. 2008; 52:e143–e263.
2. Baumgartner H, Bonhoeffer P, De Groot NM, et al. ESC Guidelines for the management of grown-up congenital heart disease (new version 2010). Eur Heart J. 2010; 31:2915–2957.
3. Bouzas B, Kilner PJ, Gatzoulis MA. Pulmonary regurgitation: not a benign lesion. Eur Heart J. 2005; 26:433–439.
4. Knauth AL, Gauvreau K, Powell AJ, et al. Ventricular size and function assessed by cardiac MRI predict major adverse clinical outcomes late after tetralogy of Fallot repair. Heart. 2008; 94:211–216.
5. Redington AN. Determinants and assessment of pulmonary regurgitation in tetralogy of Fallot: practice and pitfalls. Cardiol Clin. 2006; 24:631–639. vii
6. Frigiola A, Redington AN, Cullen S, Vogel M. Pulmonary regurgitation is an important determinant of right ventricular contractile dysfunction in patients with surgically repaired tetralogy of Fallot. Circulation. 2004; 110:11 Suppl 1. II153–II157.
7. Helbing WA, Niezen RA, Le Cessie S, et al. Right ventricular diastolic function in children with pulmonary regurgitation after repair of tetralogy of Fallot: volumetric evaluation by magnetic resonance velocity mapping. J Am Coll Cardiol. 1996; 28:1827–1835.
8. Davlouros PA, Kilner PJ, Hornung TS, et al. Right ventricular function in adults with repaired tetralogy of Fallot assessed with cardiovascular magnetic resonance imaging: detrimental role of right ventricular outflow aneurysms or akinesia and adverse right-to-left ventricular interaction. J Am Coll Cardiol. 2002; 40:2044–2052.
9. Mueller M, Rentzsch A, Hoetzer K, et al. Assessment of interventricular and right-intraventricular dyssynchrony in patients with surgically repaired tetralogy of Fallot by two-dimensional speckle tracking. Eur J Echocardiogr. 2010; 11:786–792.
10. Geva T, Sandweiss BM, Gauvreau K, Lock JE, Powell AJ. Factors associated with impaired clinical status in long-term survivors of tetralogy of Fallot repair evaluated by magnetic resonance imaging. J Am Coll Cardiol. 2004; 43:1068–1074.
11. Wessel HU, Cunningham WJ, Paul MH, Bastanier CK, Muster AJ, Idriss FS. Exercise performance in tetralogy of Fallot after intracardiac repair. J Thorac Cardiovasc Surg. 1980; 80:582–593.
12. Gatzoulis MA, Balaji S, Webber SA, et al. Risk factors for arrhythmia and sudden cardiac death late after repair of tetralogy of Fallot: a multicentre study. Lancet. 2000; 356:975–981.
13. Cullen S, Shore D, Redington A. Characterization of right ventricular diastolic performance after complete repair of tetralogy of Fallot. Restrictive physiology predicts slow postoperative recovery. Circulation. 1995; 91:1782–1789.
14. Gatzoulis MA, Clark AL, Cullen S, Newman CG, Redington AN. Right ventricular diastolic function 15 to 35 years after repair of tetralogy of Fallot. Restrictive physiology predicts superior exercise performance. Circulation. 1995; 91:1775–1781.
15. Liang XC, Lam WW, Cheung EW, Wu AK, Wong SJ, Cheung YF. Restrictive right ventricular physiology and right ventricular fibrosis as assessed by cardiac magnetic resonance and exercise capacity after biventricular repair of pulmonary atresia and intact ventricular septum. Clin Cardiol. 2010; 33:104–110.
16. Graham TP Jr, Bernard YD, Mellen BG, et al. Long-term outcome in congenitally corrected transposition of the great arteries: a multi-institutional study. J Am Coll Cardiol. 2000; 36:255–261.
17. Prieto LR, Hordof AJ, Secic M, Rosenbaum MS, Gersony WM. Progressive tricuspid valve disease in patients with congenitally corrected transposition of the great arteries. Circulation. 1998; 98:997–1005.
18. Warnes CA. Transposition of the great arteries. Circulation. 2006; 114:2699–2709.
19. Metton O, Gaudin R, Ou P, et al. Early prophylactic pulmonary artery banding in isolated congenitally corrected transposition of the great arteries. Eur J Cardiothorac Surg. 2010; 38:728–734.
20. Winlaw DS, McGuirk SP, Balmer C, et al. Intention-to-treat analysis of pulmonary artery banding in conditions with a morphological right ventricle in the systemic circulation with a view to anatomic biventricular repair. Circulation. 2005; 111:405–411.
21. Van de Veire NR, Yu CM, Ajmone-Marsan N, et al. Triplane tissue Doppler imaging: a novel three-dimensional imaging modality that predicts reverse left ventricular remodelling after cardiac resynchronisation therapy. Heart. 2008; 94:e9.
22. Lubiszewska B, Gosiewska E, Hoffman P, et al. Myocardial perfusion and function of the systemic right ventricle in patients after atrial switch procedure for complete transposition: long-term follow-up. J Am Coll Cardiol. 2000; 36:1365–1370.
23. Singh TP, Humes RA, Muzik O, et al. Myocardial flow reserve in patients with a systemic right ventricle after atrial switch repair. J Am Coll Cardiol. 2001; 37:2120–2125.
24. Babu-Narayan SV, Goktekin O, Moon JC, et al. Late gadolinium enhancement cardiovascular magnetic resonance of the systemic right ventricle in adults with previous atrial redirection surgery for transposition of the great arteries. Circulation. 2005; 111:2091–2098.
25. Plymen CM, Sado DM, Taylor AM, et al. Diffuse myocardial fibrosis in the systemic right ventricle of patients late after Mustard or Senning surgery: an equilibrium contrast cardiovascular magnetic resonance study. Eur Heart J Cardiovasc Imaging. 2013; 14:963–968.
26. Dos L, Teruel L, Ferreira IJ, et al. Late outcome of Senning and Mustard procedures for correction of transposition of the great arteries. Heart. 2005; 91:652–656.
27. Wilson NJ, Clarkson PM, Barratt-Boyes BG, et al. Long-term outcome after the mustard repair for simple transposition of the great arteries. 28-year follow-up. J Am Coll Cardiol. 1998; 32:758–765.
28. Roos-Hesselink JW, Meijboom FJ, Spitaels SE, et al. Decline in ventricular function and clinical condition after Mustard repair for transposition of the great arteries (a prospective study of 22-29 years). Eur Heart J. 2004; 25:1264–1270.
29. Derrick GP, Narang I, White PA, et al. Failure of stroke volume augmentation during exercise and dobutamine stress is unrelated to loadindependent indexes of right ventricular performance after the Mustard operation. Circulation. 2000; 102:19 Suppl 3. III154–III159.
30. Colan SD. Ventricular function in volume overload in ventricular function and blood flow. In : Fogel MA, editor. Congenital Heart Disease. New York: Blackwell Futura;2005. p. 217–220.
31. Kelly DT, Spotnitz HM, Beiser GD, Pierce JE, Epstein SE. Effects of chronic right ventricular volume and pressure loading on left ventricular performance. Circulation. 1971; 44:403–412.
32. Gewillig M. The Fontan circulation. Heart. 2005; 91:839–846.
33. Sanchez-Quintana D, Climent V, Ho SY, Anderson RH. Myoarchitecture and connective tissue in hearts with tricuspid atresia. Heart. 1999; 81:182–191.
34. Ho SY, Jackson M, Kilpatrick L, Smith A, Gerlis LM. Fibrous matrix of ventricular myocardium in tricuspid atresia compared with normal heart. A quantitative analysis. Circulation. 1996; 94:1642–1646.
35. Penny DJ, Redington AN. Diastolic ventricular function after the Fontan operation. Am J Cardiol. 1992; 69:974–975.
36. Cheung YF, Penny DJ, Redington AN. Serial assessment of left ventricular diastolic function after Fontan procedure. Heart. 2000; 83:420–424.
37. Senzaki H, Masutani S, Kobayashi J, et al. Ventricular afterload and ventricular work in fontan circulation: comparison with normal twoventricle circulation and single-ventricle circulation with blalocktaussig shunts. Circulation. 2002; 105:2885–2892.
38. Jiang L, Wiegers SE, Weyman AE. Right ventricle. In : Weyman AE, editor. Principles and Practice of Echocardiography. 2nd ed. Philadelphia: Lea & Febiger;1994. p. 901–921.
39. Koestenberger M, Nagel B, Ravekes W, et al. Systolic right ventricular function in pediatric and adolescent patients with tetralogy of Fallot: echocardiography versus magnetic resonance imaging. J Am Soc Echocardiogr. 2011; 24:45–52.
40. Mercer-Rosa L, Parnell A, Forfia PR, Yang W, Goldmuntz E, Kawut SM. Tricuspid annular plane systolic excursion in the assessment of right ventricular function in children and adolescents after repair of tetralogy of Fallot. J Am Soc Echocardiogr. 2013; 26:1322–1329.
41. Kalogeropoulos AP, Deka A, Border W, et al. Right ventricular function with standard and speckle-tracking echocardiography and clinical events in adults with D-transposition of the great arteries post atrial switch. J Am Soc Echocardiogr. 2012; 25:304–312.
42. De Caro E, Bondanza S, Calevo MG, et al. Tricuspid Annular Plane Systolic Excursion for the Assessment of Ventricular Function in Adults Operated on with Mustard Procedure for Complete Transposition of the Great Arteries. Congenit Heart Dis. 2013; [Epub ahead of print].
43. Koestenberger M, Ravekes W, Everett AD, et al. Right ventricular function in infants, children and adolescents: reference values of the tricuspid annular plane systolic excursion (TAPSE) in 640 healthy patients and calculation of z score values. J Am Soc Echocardiogr. 2009; 22:715–719.
44. Lopez L, Colan SD, Frommelt PC, et al. Recommendations for quantification methods during the performance of a pediatric echocardiogram: a report from the Pediatric Measurements Writing Group of the American Society of Echocardiography Pediatric and Congenital Heart Disease Council. J Am Soc Echocardiogr. 2010; 23:465–495. quiz 576-7.
45. Hung J, Lang R, Flachskampf F, et al. 3D echocardiography: a review of the current status and future directions. J Am Soc Echocardiogr. 2007; 20:213–233.
46. Soriano BD, Hoch M, Ithuralde A, et al. Matrix-array 3-dimensional echocardiographic assessment of volumes, mass, and ejection fraction in young pediatric patients with a functional single ventricle: a comparison study with cardiac magnetic resonance. Circulation. 2008; 117:1842–1848.
47. Tei C, Ling LH, Hodge DO, et al. New index of combined systolic and diastolic myocardial performance: a simple and reproducible measure of cardiac function--a study in normals and dilated cardiomyopathy. J Cardiol. 1995; 26:357–366.
48. Sutherland GR, Stewart MJ, Groundstroem KW, et al. Color Doppler myocardial imaging: a new technique for the assessment of myocardial function. J Am Soc Echocardiogr. 1994; 7:441–458.
49. Vogel M, Schmidt MR, Kristiansen SB, et al. Validation of myocardial acceleration during isovolumic contraction as a novel noninvasive index of right ventricular contractility: comparison with ventricular pressure-volume relations in an animal model. Circulation. 2002; 105:1693–1699.
50. Hillis GS, Møller JE, Pellikka PA, et al. Noninvasive estimation of left ventricular filling pressure by E/e' is a powerful predictor of survival after acute myocardial infarction. J Am Coll Cardiol. 2004; 43:360–367.
51. Abbas A, Lester S, Moreno FC, Srivathsan K, Fortuin D, Appleton C. Noninvasive assessment of right atrial pressure using Doppler tissue imaging. J Am Soc Echocardiogr. 2004; 17:1155–1160.
52. Tekten T, Onbasili AO, Ceyhan C, Unal S, Discigil B. Novel approach to measure myocardial performance index: pulsed-wave tissue Doppler echocardiography. Echocardiography. 2003; 20:503–510.
53. Abraham TP, Nishimura RA. Myocardial strain: can we finally measure contractility? J Am Coll Cardiol. 2001; 37:731–734.
54. Greenberg NL, Firstenberg MS, Castro PL, et al. Doppler-derived myocardial systolic strain rate is a strong index of left ventricular contractility. Circulation. 2002; 105:99–105.
55. Nagueh SF, Appleton CP, Gillebert TC, et al. Recommendations for the evaluation of left ventricular diastolic function by echocardiography. J Am Soc Echocardiogr. 2009; 22:107–133.
56. Vendelin M, Bovendeerd PHM, Engelbrecht J, Arts T. Optimizing ventricular fibers: uniform strain or stress, but not ATP consumption, leads to high efficiency. Am J Physiol Heart Circ Physiol. 2002; 283:H1072–H1081.
57. Lorenz CH, Pastorek JS, Bundy JM. Delineation of normal human left ventricular twist throughout systole by tagged cine magnetic resonance imaging. J Cardiovasc Magn Reson. 2000; 2:97–108.
58. Burns AT, La Gerche A, Prior DL, Macisaac AI. Left ventricular untwisting is an important determinant of early diastolic function. JACC Cardiovasc Imaging. 2009; 2:709–716.
59. Rüssel IK, Götte MJ, Bronzwaer JG, Knaapen P, Paulus WJ, van Rossum AC. Left ventricular torsion: an expanding role in the analysis of myocardial dysfunction. JACC Cardiovasc Imaging. 2009; 2:648–655.
60. Young AA. Ventricular torsion: an aid to ejection? JACC Cardiovasc Imaging. 2012; 5:282–284.
61. Cheng A, Helm RH, Abraham TP. Pathophysiological mechanisms underlying ventricular dyssynchrony. Europace. 2009; 11:Suppl 5. v10–v14.
62. van der Hulst AE, Delgado V, Blom NA, et al. Cardiac resynchronization therapy in paediatric and congenital heart disease patients. Eur Heart J. 2011; 32:2236–2246.
63. Janousek J. Cardiac resynchronisation in congenital heart disease. Heart. 2009; 95:940–947.
64. Heimdal A, Støylen A, Torp H, Skjaerpe T. Real-time strain rate imaging of the left ventricle by ultrasound. J Am Soc Echocardiogr. 1998; 11:1013–1019.
65. Urheim S, Edvardsen T, Torp H, Angelsen B, Smiseth OA. Myocardial strain by Doppler echocardiography. Validation of a new method to quantify regional myocardial function. Circulation. 2005; 102:1158–1164.
66. Leitman M, Lysyansky P, Sidenko S, et al. Two-dimensional strain-a novel software for real-time quantitative echocardiographic assessment of myocardial function. J Am Soc Echocardiogr. 2004; 17:1021–1029.
67. Amundsen BH, Helle-Valle T, Edvardsen T, et al. Noninvasive myocardial strain measurement by speckle tracking echocardiography: validation against sonomicrometry and tagged magnetic resonance imaging. J Am Coll Cardiol. 2006; 47:789–793.
68. Reisner SA, Lysyansky P, Agmon Y, Mutlak D, Lessick J, Friedman Z. Global longitudinal strain: a novel index of left ventricular systolic function. J Am Soc Echocardiogr. 2004; 17:630–633.
69. Chow PC, Liang XC, Cheung EW, Lam WW, Cheung YF. New two-dimensional global longitudinal strain and strain rate imaging for assessment of systemic right ventricular function. Heart. 2008; 94:855–859.
70. Cheung EW, Liang XC, Lam WW, Cheung YF. Impact of right ventricular dilation on left ventricular myocardial deformation in patients after surgical repair of tetralogy of fallot. Am J Cardiol. 2009; 104:1264–1270.
71. Notomi Y, Lysyansky P, Setser RM, et al. Measurement of ventricular torsion by two-dimensional ultrasound speckle tracking imaging. J Am Coll Cardiol. 2005; 45:2034–2041.
72. Cheung YF. The role of 3D wall motion tracking in heart failure. Nat Rev Cardiol. 2012; 9:644–657.
73. Seo Y, Ishizu T, Enomoto Y, Sugimori H, Aonuma K. Endocardial surface area tracking for assessment of regional LV wall deformation with 3D speckle tracking imaging. JACC Cardiovasc Imaging. 2011; 4:358–365.
74. Li SN, Wong SJ, Cheung YF. Novel area strain based on three-dimensional wall motion analysis for assessment of global left ventricular performance after repair of tetralogy of Fallot. J Am Soc Echocardiogr. 2011; 24:819–825.
75. Yodwut C, Weinert L, Klas B, Lang RM, Mor-Avi V. Effects of frame rate on three-dimensional speckle-tracking-based measurements of myocardial deformation. J Am Soc Echocardiogr. 2012; 25:978–985.
76. Onishi T, Onishi T, Tanaka T, Haberman SC, Champion H, Gorcsan J. Three dimensional speckle tracking strain evaluation of right heart function and hemodynamics in patients with pulmonary hypertension. Circulation. 2012; 126:A14407.
77. Smiseth OA, Russell K, Skulstad H. The role of echocardiography in quantification of left ventricular dyssynchrony: state of the art and future directions. Eur Heart J Cardiovasc Imaging. 2012; 13:61–68.
78. Nesser HJ, Winter S. Speckle tracking in the evaluation of left ventricular dyssynchrony. Echocardiography. 2009; 26:324–336.
79. Kapetanakis S, Kearney MT, Siva A, Gall N, Cooklin M, Monaghan MJ. Real-time three-dimensional echocardiography: a novel technique to quantify global left ventricular mechanical dyssynchrony. Circulation. 2005; 112:992–1000.
80. Silversides CK, Salehian O, Oechslin E, et al. Canadian Cardiovascular Society 2009 Consensus Conference on the management of adults with congenital heart disease: complex congenital cardiac lesions. Can J Cardiol. 2010; 26:e98–e117.
81. Weidemann F, Eyskens B, Mertens L, et al. Quantification of regional right and left ventricular function by ultrasonic strain rate and strain indexes after surgical repair of tetralogy of Fallot. Am J Cardiol. 2002; 90:133–138.
82. Friedberg MK, Fernandes FP, Roche SL, et al. Relation of right ventricular mechanics to exercise tolerance in children after tetralogy of Fallot repair. Am Heart J. 2013; 165:551–557.
83. Roche SL, Grosse-Wortmann L, Redington AN, et al. Exercise induces biventricular mechanical dyssynchrony in children with repaired tetralogy of Fallot. Heart. 2010; 96:2010–2015.
84. Friedberg MK, Fernandes FP, Roche SL, et al. Impaired right and left ventricular diastolic myocardial mechanics and filling in asymptomatic children and adolescents after repair of tetralogy of Fallot. Eur Heart J Cardiovasc Imaging. 2012; 13:905–913.
85. Abd El Rahman MY, Abdul-Khaliq H, Vogel M, et al. Value of the new Doppler-derived myocardial performance index for the evaluation of right and left ventricular function following repair of tetralogy of fallot. Pediatr Cardiol. 2002; 23:502–507.
86. Cheung EW, Lam WW, Cheung SC, Cheung YF. Functional implications of the right ventricular myocardial performance index in patients after surgical repair of tetralogy of Fallot. Heart Vessels. 2008; 23:112–117.
87. Mercer-Rosa L, Yang W, Kutty S, Rychik J, Fogel M, Goldmuntz E. Quantifying pulmonary regurgitation and right ventricular function in surgically repaired tetralogy of Fallot: a comparative analysis of echocardiography and magnetic resonance imaging. Circ Cardiovasc Imaging. 2012; 5:637–643.
88. Takayasu H, Takahashi K, Takigiku K, et al. Left ventricular torsion and strain in patients with repaired tetralogy of Fallot assessed by speckle tracking imaging. Echocardiography. 2011; 28:720–729.
89. Cheung YF, Wong SJ, Liang XC, Cheung EW. Torsional mechanics of the left ventricle in patients after surgical repair of tetralogy of Fallot. Circ J. 2011; 75:1735–1741.
90. Li SN, Yu W, Lai CT, Wong SJ, Cheung YF. Left ventricular mechanics in repaired tetralogy of Fallot with and without pulmonary valve replacement: analysis by three-dimensional speckle tracking echocardiography. PLoS One. 2013; 8:e78826.
91. Moiduddin N, Asoh K, Slorach C, Benson LN, Friedberg MK. Effect of transcatheter pulmonary valve implantation on short-term right ventricular function as determined by two-dimensional speckle tracking strain and strain rate imaging. Am J Cardiol. 2009; 104:862–867.
92. Harrild DM, Marcus E, Hasan B, et al. Impact of transcatheter pulmonary valve replacement on biventricular strain and synchrony assessed by cardiac magnetic resonance feature tracking. Circ Cardiovasc Interv. 2013; 6:680–687.
93. Diller GP, Kempny A, Liodakis E, et al. Left ventricular longitudinal function predicts life-threatening ventricular arrhythmia and death in adults with repaired tetralogy of fallot. Circulation. 2012; 125:2440–2446.
94. Mi YP, Cheung YF. Assessment of right and left ventricular function by tissue Doppler echocardiography in patients after biventricular repair of pulmonary atresia with intact ventricular septum. Int J Cardiol. 2006; 109:329–334.
95. Eyskens B, Weidemann F, Kowalski M, et al. Regional right and left ventricular function after the Senning operation: an ultrasonic study of strain rate and strain. Cardiol Young. 2004; 14:255–264.
96. Rentzsch A, Abd El, Hui W, et al. Assessment of myocardial function of the systemic right ventricle in patients with D-transposition of the great arteries after atrial switch operation by tissue Doppler echocardiography. Z Kardiol. 2005; 94:524–531.
97. Vogel M, Derrick G, White PA, et al. Systemic ventricular function in patients with transposition of the great arteries after atrial repair: a tissue Doppler and conductance catheter study. J Am Coll Cardiol. 2004; 43:100–106.
98. Vogt M, Kühn A, Wiese J, Eicken A, Hess J, Vogel M. Reduced contractile reserve of the systemic right ventricle under Dobutamine stress is associated with increased brain natriuretic peptide levels in patients with complete transposition after atrial repair. Eur J Echocardiogr. 2009; 10:691–694.
99. Chow PC, Cheung EW, Chong CY, et al. Brain natriuretic peptide as a biomarker of systemic right ventricular function in patients with transposition of great arteries after atrial switch operation. Int J Cardiol. 2008; 127:192–197.
100. Pettersen E, Helle-Valle T, Edvardsen T, et al. Contraction pattern of the systemic right ventricle shift from longitudinal to circumferential shortening and absent global ventricular torsion. J Am Coll Cardiol. 2007; 49:2450–2456.
101. Chow PC, Liang XC, Lam WW, Cheung EW, Wong KT, Cheung YF. Mechanical right ventricular dyssynchrony in patients after atrial switch operation for transposition of the great arteries. Am J Cardiol. 2008; 101:874–881.
102. Chow PC, Liang XC, Cheung YF. Diastolic ventricular interaction in patients after atrial switch for transposition of the great arteries: a speckle tracking echocardiographic study. Int J Cardiol. 2011; 152:28–34.
103. Diller GP, Radojevic J, Kempny A, et al. Systemic right ventricular longitudinal strain is reduced in adults with transposition of the great arteries, relates to subpulmonary ventricular function, and predicts adverse clinical outcome. Am Heart J. 2012; 163:859–866.
104. Bos JM, Hagler DJ, Silvilairat S, et al. Right ventricular function in asymptomatic individuals with a systemic right ventricle. J Am Soc Echocardiogr. 2006; 19:1033–1037.
105. Sun HY, Behzadian F, Punn R, Tacy TA. Decremental left ventricular deformation after pulmonary artery band training and subsequent repair in ventriculoarterial discordance. J Am Soc Echocardiogr. 2013; 26:765–774.
106. Losay J, Touchot A, Serraf A, et al. Late outcome after arterial switch operation for transposition of the great arteries. Circulation. 2001; 104:12 Suppl 1. I121–I126.
107. Wernovsky G, Hougen TJ, Walsh EP, et al. Midterm results after the arterial switch operation for transposition of the great arteries with intact ventricular septum: clinical, hemodynamic, echocardiographic, and electrophysiologic data. Circulation. 1988; 77:1333–1344.
108. Pettersen E, Fredriksen PM, Urheim S, et al. Ventricular function in patients with transposition of the great arteries operated with arterial switch. Am J Cardiol. 2009; 104:583–589.
109. Hui L, Chau AK, Leung MP, Chiu CS, Cheung YF. Assessment of left ventricular function long term after arterial switch operation for transposition of the great arteries by dobutamine stress echocardiography. Heart. 2005; 91:68–72.
110. Chen RH, Wong SJ, Wong WH, Cheung YF. Left ventricular contractile reserve after arterial switch operation for complete transposition of the great arteries: an exercise echocardiographic study. Eur Heart J Cardiovasc Imaging. 2013; 14:480–486.
111. Ho PK, Lai CT, Wong SJ, Cheung YF. Three-dimensional mechanical dyssynchrony and myocardial deformation of the left ventricle in patients with tricuspid atresia after Fontan procedure. J Am Soc Echocardiogr. 2012; 25:393–400.
112. Truong UT, Li X, Broberg CS, et al. Significance of mechanical alterations in single ventricle patients on twisting and circumferential strain as determined by analysis of strain from gradient cine magnetic resonance imaging sequences. Am J Cardiol. 2010; 105:1465–1469.
113. Kaneko S, Khoo NS, Smallhorn JF, Tham EB. Single right ventricles have impaired systolic and diastolic function compared to those of left ventricular morphology. J Am Soc Echocardiogr. 2012; 25:1222–1230.
114. Cheung MM, Smallhorn JF, McCrindle BW, Van Arsdell GS, Redington AN. Non-invasive assessment of ventricular force-frequency relations in the univentricular circulation by tissue Doppler echocardiography: a novel method of assessing myocardial performance in congenital heart disease. Heart. 2005; 91:1338–1342.
115. Khoo NS, Smallhorn JF, Kaneko S, Myers K, Kutty S, Tham EB. Novel insights into RV adaptation and function in hypoplastic left heart syndrome between the first 2 stages of surgical palliation. JACC Cardiovasc Imaging. 2011; 4:128–137.
116. Friedberg MK, Silverman NH, Dubin AM, Rosenthal DN. Right ventricular mechanical dyssynchrony in children with hypoplastic left heart syndrome. J Am Soc Echocardiogr. 2007; 20:1073–1079.
117. Akagi T, Benson LN, Williams WG, Freedom RM. Regional ventricular wall motion abnormalities in tricuspid atresia after the Fontan procedure. J Am Coll Cardiol. 1993; 22:1182–1188.
118. Fogel MA, Gupta KB, Weinberg PM, Hoffman EA. Regional wall motion and strain analysis across stages of Fontan reconstruction by magnetic resonance tagging. Am J Physiol. 1995; 269(3 Pt 2):H1132–H1152.
119. Rathod RH, Prakash A, Powell AJ, Geva T. Myocardial fibrosis identified by cardiac magnetic resonance late gadolinium enhancement is associated with adverse ventricular mechanics and ventricular tachycardia late after Fontan operation. J Am Coll Cardiol. 2010; 55:1721–1728.
120. Lorch SM, Ludomirsky A, Singh GK. Maturational and growth-related changes in left ventricular longitudinal strain and strain rate measured by two-dimensional speckle tracking echocardiography in healthy pediatric population. J Am Soc Echocardiogr. 2008; 21:1207–1215.
121. Marcus KA, Mavinkurve-Groothuis AM, Barends M, et al. Reference values for myocardial two-dimensional strain echocardiography in a healthy pediatric and young adult cohort. J Am Soc Echocardiogr. 2011; 24:625–636.
122. Marcus KA, Janousek J, Barends ME, Weijers G, de Korte CL, Kapusta L. Synchronicity of systolic deformation in healthy pediatric and young adult subjects: a two-dimensional strain echocardiography study. Am J Physiol Heart Circ Physiol. 2012; 302:H196–H205.
123. Manovel A, Dawson D, Smith B, Nihoyannopoulos P. Assessment of left ventricular function by different speckle-tracking software. Eur J Echocardiogr. 2010; 11:417–421.
124. Gayat E, Ahmad H, Weinert L, Lang RM, Mor-Avi V. Reproducibility and inter-vendor variability of left ventricular deformation measurements by three-dimensional speckle-tracking echocardiography. J Am Soc Echocardiogr. 2011; 24:878–885.
125. Yuda S, Sato Y, Mina M. Inter-vendor variability of left ventricular volumes and strains determined by three-dimensional speckle tracking echocardiography. Echocardiography. 2013; [Epub ahead of print].