Abstract
The efficacy and safety of catheter ablation for the management of atrial fibrillation (AF) has been improved in recent years. Radiofrequency (RF) catheter ablation for maintaining sinus rhythm is superior to the current antiarrhythmic drug therapy in selected patients. Pulmonary vein isolation (PVI) is the cornerstone of various catheter ablation strategies. It is well recognized that pulmonary vein (PV) antrum contributes to the AF initiation and/or perpetuation. Since PV stenosis is a complication of ablation within a PV, the ablation site for PVI has shifted to the junction between the left atrium and the PV rather than the ostium of the PV. However, PV reconnection after ablation is the major cause of recurrence of AF. The recovery of PV conduction could be caused by anatomical variations such as the failure to produce complete transmural lesion or gaps at the ablation line due to the transient electrophysiologic effects from the RF ablation. In this review, we discussed several factors to be considered for the achievement of the best PVI, including clinical aspects and technical aspects.
Catheter ablation has been evolving rapidly for the management of atrial fibrillation (AF) with improvements in both efficacy and safety.1) The efficacy of radiofrequency (RF) catheter ablation for maintaining sinus rhythm is superior to the current antiarrhythmic drug therapy in selected patients.2),3),4) The goal of catheter ablation is to eliminate AF. The development of AF requires both a trigger and a susceptible substrate. The most common trigger initiating AF comes from the myocardial sleeve of the left atrium (LA), which extends into the pulmonary veins (PV).5) Unique anatomical and electrophysiological features of the PV and LA-PV junction (LA-PVJ) play major roles in AF. Therefore, PV isolation (PVI) or LA-PVJ ablation is the cornerstone of various catheter ablation strategies.1).
Techniques used for PVI vary widely from center to center. Electrical isolation introduced by Haissaguerre et al.6) involves the sequential identification and ablation of the PV myocardial sleeve close to the earliest site of PV activation. However, PV antrum also contributes to AF initiation and/or perpetuation.7),8),9),10) In addition, PV stenosis could be a complication of ablation within a PV. Therefore, ablation strategies have shifted to target the antrum rather than the PV proper. Although PVI is the most common AF ablation procedure performed to treat various forms of AF, its results varies widely among institutions. There are many possible reasons contributing to different results. Among them, the most important one is the lack of a standardized and systematic method and technique to achieve PVI. In addition, reliable acute predictor during PVI for long-term success is unavailable.
Atrial fibrillation catheter ablation has risks of major complications. International survey and European multinational registry reported a 4.5-7.7% incidence of major complications associated with AF catheter ablation.11)12) Many of these complications could be avoided with careful pre-procedural assessment of patients and detailed planning of the procedure. The majority of the collateral damages as complications of the ablation attributed to individual anatomical variations. Although these complications are not frequent, they have been observed several times even in experienced centers. The current safety and efficacy measures used during the AF ablation have limitations due to the lack of real-time visualization and characterization of the cardiac tissue, especially in lesion formation. There are three major techniques to achieve electrical PVI during AF catheter ablation: 1) Segmental ablation, the earliest PV potential guided segmental and ostial ablation; 2) LA-PVJ ablation, a PVI by circumferential ablation at LA-PVJ; 3) Wide antral circumferential ablation (WACA), an ablation at the LA outside the LA-PVJ.
In this review article, we aimed to characterize each PVI technique so the best PVI result could be achieved in each patient within the given condition of electrophysiology laboratory. It is important to keep in mind that these techniques are not exclusive but rather mutual complementary in some patients. We also included our experiences with a few useful clinical observations to provide better understanding of AF ablation procedures.
Pre-procedure evaluation starts with the first clinical encounter which should include a clear documentation of clinical events to justify the PVI. It is important to assess clinical status of not only cardiac conditions, such as congestive heart failure or history of rheumatic fever, but also other associated clinical conditions that may influence the outcome or may increase the risks during PVI. Clinical condition examples include: 1) Chronic obstructive lung disease, which may require special respiratory support during the sedation; 2) Diabetes mellitus, which may require close monitoring of blood glucose; 3) Renal disease, which may require judicious fluid management as well as hemodynamic monitoring. These clinical conditions may help avoid fluid overload, therefore minimizing the risk for respiratory distresses or exacerbation of renal failure due to hypotension during PVI. It is also important to share and discuss these concerns with the patients.
Physical evaluation should be performed in consideration of AF ablation procedure which should include the exam of the vascular access site. Some patients may have extensive scar over the vascular access site due to pre-existing conditions or previous procedures or surgeries. In patients with pre-existing scar tissues, a generous skin incision after the venous access followed by a good dilatation prior to the insertion of the sheath is recommended. A small skin incision might create a significant resistance during the sheath and catheter manipulations. Generous skin incision is particularly important during trans-septal catheterization which requires delicate sheath manipulation in order to puncture the ideal site within the fossa ovale.
Special clinical circumstances may require additional consideration for PVI. If the patient presents with significant chest deformities such as scoliosis, lordosis, pectus excavatum (Fig. 1A and B), or Marfan syndrome (Fig. 1C and D), they can create difficulties during trans-septal catheterization as well as catheter manipulation to achieve successful PVI. Other clinical conditions such as prior pneumonectomy or hemi-diaphragm paralysis can also change attitudinal cardiac anatomy.
Pre-procedural evaluation of 3-D cardiac imaging has become an essential part in AF ablation. Cardiac CT angiography (cCTA) is the most widely used imaging modality. Modern cCTA provides detailed anatomy, including the relationship of LA, PVs, LA-PVJ, and LA appendage. Cardiac MRI (cMRI) is the other 3-D imaging modality that provides not only anatomical information for cardiac function, but also pre-existing myocardial fibrosis with its delayed enhancement imaging technology.
The objective of 3-D cardiac imaging is to not only evaluate the patient's detailed cardiac anatomy, but also recognize the relationship between LA and its neighboring structures that are subject to collateral damages during PVI, such as esophagus, major proximal arteries, and pulmonary parenchyma. However, some neighboring structures could not be imaged with cCTA or cMRI, such as phrenic and recurrent laryngeal nerves. In addition, they could be injured during PVI despite best efforts.
3-D reconstructed LA image can be used to guide the catheter manipulation during PVI. The potential ablation sites or lines can be pre-selected and planned prior to PVI. The anatomy of LA-PVJ and its relationship with the esophagus or descending aorta may force a change in the ablation strategy to avoid collateral damages. For example, if the left PV posterior wall is in close contact with the esophagus, segmental ostial PVI of the posterior wall combined with the continuous ablation at the remaining part of LA-PVJ or the antrum could be planned (Fig. 2).
From our past experiences, we have learned that CT and MRI may diagnose unsuspected non-cardiac conditions such as lung mass or cancer, large hiatal hernia (Fig. 3), or significant vertebral deformities, which may change the planned ablation strategies or abandon the ablation completely.
Of the three major ablation techniques, the best one is determined by the LA anatomy and its surrounding structures. Understanding the anatomy and the histology of PV and LA-PVJ are very important for effective catheter ablation.
Histological and embryological studies of the human LA revealed complex and wide variations during the development of LA and PVs from primordial LA and venous system, which may explain the wide anatomical variations at the LA-PVJ and PVs observed in patients with AF. These variations may also occur not only at gross anatomical level but also at histological level, resulting in the differences in the length, orientation, and thickness of the atrial myocardial fibers.13) The myocardial muscle fibers with a circumferential and spiral orientation form the thick heterogeneous ostial muscle sleeves, which may progressively become thinner toward the distal PV and end up being organized to parallel along the PV long axis (Fig. 4). It is important to note that these myocardial sleeves are predominantly epicardial structures and that their lengths vary widely between the veins as well as the diameters of the PVs.14) These histological characteristics of myocardial sleeve directly influence the ablation lesion formation and ultimately the long-term PVI. Although it is easy to achieve transmural lesions inside the PV with a small amount of ablation due to the thin and homogenously oriented myocardial fibers, it has a high risk of PV stenosis and collateral damages. In contrast, the WACA technique requires a much larger number of ablation and higher energy to achieve complete isolation because thick atrial myocardial sleeves with multiple muscle layers present in most of the PV antrum, which is less likely to achieve homogenous transmural lesions in the entire circumference with the current available ablation technologies. Therefore, those circumstances favor the use of LA-PVJ ablation technique. LA-PVJ ablation could also eliminate other possible triggers, such as Marshall bundle and posterior LA wall. In addition, LA-PVJ ablation might alter the arrhythmogenic substrate that may generate or perpetuate AF and/or reduce the mass of atrial tissue needed to sustain reentry. Furthermore, neuro-anatomical studies at the LA-PVJ revealed circumferential distribution of a high number of autonomic parasympathetic and sympathetic nerves in LA-PVJ.15) Therefore, LA-PVJ ablation may interrupt the autonomic innervations, the potential triggers for AF.
The single most important technical factor for successful LA-PVJ ablation is a proper trans-septal catheterization. Each patient's LA and PVs have different shapes, orientations, and sizes. Therefore, the trans-septal catheterization site should be adjusted accordingly. During LA-PVJ ablation, the most difficult areas commonly encountered are the upper border of superiorly oriented superior PVs and the lower border of posteriorly oriented right inferior PV (Fig. 5). In general, most centers prefer double trans-septal catheterizations to address these areas with trans-septal puncture sites at the superior-anterior and the posterior-inferior portion of fossa ovale (Fig. 6).
The recovery of PV conduction after PVI is still the most challenging issue due to anatomical reasons such as failure to produce complete transmural lesions,16)17) gaps in the line of ablations,18) or the transient electrophysiologic effects of RF ablation. Ideal ablation lesion formation depends on several factors, including good and constant contact pressure and duration. The following three indicators could be used to suggest good ablation lesion formation: 1) Elimination of target potential within 5 seconds by energy delivery; 2) Impedance drop more than 7 Ohms within 10 seconds of energy delivery; 3) Loss of capture of pacing after ablation. However, it is technically challenging to maintain a constant contact with optimal pressure for a reasonable duration of 30 to 40 seconds in a beating heart. Furthermore, these consistent lesions must be connected continuously to avoid gaps. If PVI is performed only based on the potential reduction, RF ablation duration can be reduced, but the transmurality of the lesion is unlikely. RF lesion reaches maturity after a 20 second exposure of power. The lesion size is determined by integration of exposure duration and power level.19)20) Ablation duration requires 30 to 40 seconds in most of the sites during PVI for a good lesion formation. The most important factor for creating ideal lesion formation is a careful monitoring of the ablation electrogram, which is crucial to confirm proper contact and eliminate target potential with the energy delivery. The ablation energy delivery is continued for an additional 25 to 35 seconds after the initial loss of R wave on the ablation electrogram (Fig. 7) when using open irrigated ablation technology with the power of 20 to 30 Watts and cooling flow of 8 to 30 mL/min. Inability to watch the electrogram during ablation is commonly caused by the contamination of a 60 Hz noise. The most common source of the 60 Hz noise is any AC power source close to the recording system such as the RF generator, defibrillator or infusion pumps. Therefore, it is essential to separate the recording cables from the power cables by bundling as well as ground all electrical equipment. The other common source of contamination is the improper skin preparation before the placement of 12 lead electrocardiography electrodes.
The ideal ablation sites around the LA-PVJ should be: 1) Favorable anatomical location for catheter manipulation to reach the target sites with stable contact throughout the energy delivery, 2) Favorable myocardial thickness for currently available ablation technologies to achieve uniform transmural lesions but with minimum risk of collateral damages.
The LA-PVJ contains the electrophysiological PV ostium (Os) and anatomical PV Os, which is located more proximal to the LA. During AF ablation, the electrophysiological Os can be easily defined by the merger between the local LA potentials and the PV potentials. Generally, the anatomical Os is located 5 mm proximal to the LA (Fig. 8). Once the anatomical Os is identified, a linear ablation line could be drawn over the anatomically merged 3-D LA map to guide PVI (Fig. 9). Even though the contact and duration is good enough to make solid lesion, if the ablation lesion is made by a point-by-point ablation, it is more likely to leave a gap between lesions which may contribute to PV reconnection.21) Therefore, it is preferred to perform the ablation with continuous dragging pattern to minimize gaps.
The ablation line should include the major carina between the superior and inferior PVs because there are crossing myocardial fibers and bridges at the interpulmonary isthmus in -50% of the patients.22) In some instances, it may be necessary to extend the ablation into the sub-carina within the PV.
There are several cardiac and non-cardiac factors that can affect lesion formation. Respiratory movement can directly influence the stability of the catheter during ablation which can be controlled with general anesthesia using a jet ventilation technique. However, this can increase the cost as well as the procedure time. Moderate to deep sedation protocol with Bi-PAP or CPAP support during the ablation can yield favorable outcomes as well as cost effectiveness.
The cardiac rhythm itself can also create catheter instability during ablation. It is well known that irregular AF can create catheter instability during PVI. Therefore, the sinus rhythm is preferred during ablation because it enables easy monitoring of the ablation electrogram as well as stable catheter position (Video in the online-only Data Supplement).
The size and the curve of the ablation catheter can be selected based on the LA dimension or volume and LA shape. The general rule used in our lab is that if the LA volume is greater than 120 mL or LA diameter is larger in horizontal dimension, a large curve ablation catheter should be chosen.
Pulmonary vein isolation is confirmed with a circular catheter once the ablation is completed. Elimination of PV potentials is the primary endpoint for PV ablation procedures in most centers. PVI can be confirmed when the dissociated PV potentials are observed. Some centers routinely perform pacing from inside PV to confirm the exit block after the elimination of the PV potentials.1) High out pacing from the ablation site to confirm the loss of capture has also been proposed as a good and acute predictor.23)
Acute PV reconnection can be observed up to 50% in 30 minutes following completion of PVI. However, it appears to occur continuously throughout 90 days even though majority of them are reconnected during the first 30 days after PVI. There are two pharmacological methods to detect acute PV reconnections. The most commonly used method is a high dose isoproterenol infusion for 10 to 15 minutes and a waiting time of 30 minutes after PVI. The more recent method is a high dose adenosine bolus injection to facilitate the acute reconnections. Regardless of the method used, acute PV reconnection is due to non-transmural lesions or gaps between the ablation lesions. Therefore, reconfirmation of PVI at 20-30 minutes after initial PVI with or without pharmacologic challenging is important to minimize the recurrences.
Two hypothesized mechanisms are involved in post PVI reconduction: 1) Acute reconnection, defined as first 90 days after the PVI, is the main reason of recurrences. This is primarily due to the failure to achieve continuous and complete trans-mural lesions; 2) Delayed recurrences which are observed over several years after the PVI.24) This is most likely due to the longitudinal ultra-structural and electrophysiological propriety changes from the healing mechanisms observed in scar tissues. However, there is no clinical data available to confirm the 2nd hypothesis. Both mechanisms may play roles in a given clinical setting.
In summary, PV reconnection is still the major clinical challenge in any form of AF ablation. Major limitations to successful PVI are: 1) The lack of good acute predictor for the continuous uniform trans-mural lesions; 2) The lack of ablation procedure endpoints to predict long term success of PVI. Technologies that can improve the real time monitoring and the visualizing of the ablation lesion formation will improve the safety as well as the efficacy of AF ablation, which will ultimately improve the long-term outcome of PVI. Further clinical researches are merited to overcome these limitations.
Pulmonary vein isolation can be approached systematically from clinical to procedural aspects. One needs to develop the PVI protocol that is practical and feasible within the given health-care environment so that it has reliable and reproducible results without complications or with very low complications.
Figures and Tables
Fig. 1
Three-dimensional reconstructed images of a left atrium. A and B: the left atrium of a patient with Pectus Excavatum. A shows the relationship between the ascending aorta and the left atrial anterior wall. B shows the indentation of the anterior wall by the aorta and the flattening of the left atrium. C and D: the left atrium of a patient with Marfan syndrome, which shows significant indentation of the posterior wall by the vertebral column.
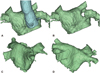
Fig. 2
The proximity of esophagus to the left pulmonary veins. The line on A shows the location of the esophagus during the CT imaging, which was located close to the ablation line (B). Continuous ablation was done in the left lateral ridge, superior, inferior, and carina of the ridge side (C). Afterwards segmental isolation of the pulmonary veins was performed following the earliest activation site of the pulmonary veins to avoid possible esophageal injury (D).
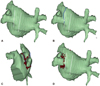
Fig. 3
Images of hiatal hernia of a stomach. A and B: the thoracic portion of the stomach (arrow) has very close contact to the posterior wall of the left atrium on CT images. C and D show 3-dimensional relationship between the herniated stomach (arrow) with the esophagus and the left atrium.
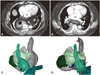
Fig. 4
Anatomical characteristics of the junction of left atrium and pulmonary vein. A: microscopic appearances of myocardial sleeves in a heart specimen. Topography of the myocardial sleeves extends along the pulmonary veins on the adventitial side. The layer of the left atrial myocardium is separated from the muscular media of the pulmonary veins by a plane of fibro-fatty tissue. B: gross appearances of myocardial sleeves around pulmonary veins. The dotted lines indicate the junction between the left atrial myocardium and the pulmonary veins revealing complete continuity. C: three-dimensional reconstruction of a cross-section, perpendicular to the long axis of part of the wall of a myocardial sleeve covering a pulmonary vein, distal to the venoatrial junction (right to left). The direction of the muscle fibers is color coded. The venoatrial junction has heterogeneous orientation of the muscle fibers (Adapted from Saito T, Waki K, Becker AE. J Cardiovasc Electrophysiol 2000;8:888-94).14)
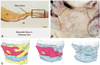
Fig. 5
The different transseptal puncture sites depend on the right inferior pulmonary vein anatomy. A shows typical anatomy of the right inferior pulmonary vein. Bottom picture shows the location of the transseptal puncture sheaths. B shows atypical location of the right inferior pulmonary vein, which projects far posteriorly and very close to the floor of the left atrium. Bottom figure shows a modified transseptal puncture according to the variation of pulmonary vein anatomy.
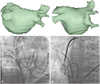
Fig. 6
A shows a right anterior oblique projection. B shows a left anterior oblique projection. It shows an anteroinferior and superoposterior double transseptal puncture. The two well-separated transseptal sheaths can provide various accesses to the pulmonary veins.
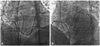
Fig. 7
Changes of electrogram by energy application. The sharp signal registered from the ablation catheter shows a loss of R wave progressively within 2 seconds after energy delivery (arrow), which implies that the catheter contact was good and the lesion formation by energy delivery was effective.
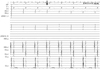
Fig. 8
Left Superior pulmonary vein mapping to identify the pulmonary vein ostium during sinus rhythm. Top picture is a left superior pulmonary venogram. Mid-row shows the location of the catheter ablation from the distal pulmonary vein to the anatomical ostium. Bottom row shows changes of the ablation electrograms according to the location. The signals from distal to mid pulmonary vein (arrows) show small single potential and later activation than the atrial activation. The signal from the electrical ostium shows high voltage multicomponent potentials with similar timing of the atrial activation. The signal of anatomical ostium has higher voltage with single component like a local atrial signal. PV: pulmonary vein, OS: ostium, Abl: ablation, HRA: high right atrium, d: distal, p: proximal.
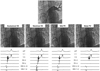
Acknowledgments
We sincerely thank Janette Lancaster and Shannon Logsdon for their assistance and contribution.
References
1. Calkins H, Kuck KH, Cappato R, et al. 2012 HRS/EHRA/ECAS expert consensus statement on catheter and surgical ablation of atrial fibrillation: recommendations for patient selection, procedural techniques, patient management and follow-up, definitions, endpoints, and research trial design: a report of the Heart Rhythm Society (HRS) Task Force on Catheter and Surgical Ablation of Atrial Fibrillation. Developed in partnership with the European Heart Rhythm Association (EHRA), a registered branch of the European Society of Cardiology (ESC) and the European Cardiac Arrhythmia Society (ECAS); and in collaboration with the American College of Cardiology (ACC), American Heart Association (AHA), the Asia Pacific Heart Rhythm Society (APHRS), and the Society of Thoracic Surgeons (STS). Endorsed by the governing bodies of the American College of Cardiology Foundation, the American Heart Association, the European Cardiac Arrhythmia Society, the European Heart Rhythm Association, the Society of Thoracic Surgeons, the Asia Pacific Heart Rhythm Society, and the Heart Rhythm Society. Heart Rhythm. 2012; 9:632–696.
2. Calkins H, Reynolds MR, Spector P, et al. Treatment of atrial fibrillation with antiarrhythmic drugs or radiofrequency ablation: two systematic literature reviews and meta-analyses. Circ Arrhythm Electrophysiol. 2009; 2:349–361.
3. Piccini JP, Lopes RD, Kong MH, Hasselblad V, Jackson K, Al-Khatib SM. Pulmonary vein isolation for the maintenance of sinus rhythm in patients with atrial fibrillation: a meta-analysis of randomized, controlled trials. Circ Arrhythm Electrophysiol. 2009; 2:626–633.
4. Parkash R, Tang AS, Sapp JL, Wells G. Approach to the catheter ablation technique of paroxysmal and persistent atrial fibrillation: a meta-analysis of the randomized controlled trials. J Cardiovasc Electrophysiol. 2011; 22:729–738.
5. Haïssaguerre M, Jaïs P, Shah DC, et al. Spontaneous initiation of atrial fibrillation by ectopic beats originating in the pulmonary veins. N Engl J Med. 1998; 339:659–666.
6. Haïssaguerre M, Jaïs P, Shah DC, et al. Electrophysiological end point for catheter ablation of atrial fibrillation initiated from multiple pulmonary venous foci. Circulation. 2000; 101:1409–1417.
7. Kalifa J, Jalife J, Zaitsev AV, et al. Intra-atrial pressure increases rate and organization of waves emanating from the superior pulmonary veins during atrial fibrillation. Circulation. 2003; 108:668–671.
8. Kumagai K, Ogawa M, Noguchi H, Yasuda T, Nakashima H, Saku K. Electrophysiologic properties of pulmonary veins assessed using a multielectrode basket catheter. J Am Coll Cardiol. 2004; 43:2281–2289.
9. Sanders P, Berenfeld O, Hocini M, et al. Spectral analysis identifies sites of high-frequency activity maintaining atrial fibrillation in humans. Circulation. 2005; 112:789–797.
10. Lin YJ, Tsao HM, Chang SL, et al. Role of high dominant frequency sites in nonparoxysmal atrial fibrillation patients: insights from high-density frequency and fractionation mapping. Heart Rhythm. 2010; 7:1255–1262.
11. Cappato R, Calkins H, Chen SA, et al. Updated worldwide survey on the methods, efficacy, and safety of catheter ablation for human atrial fibrillation. Circ Arrhythm Electrophysiol. 2010; 3:32–38.
12. Arbelo E, Brugada J, Hindricks G, et al. ESC-EURObservational Research Programme: the Atrial Fibrillation Ablation Pilot Study, conducted by the European Heart Rhythm Association. Europace. 2012; 14:1094–1103.
13. Hassink RJ, Aretz HT, Ruskin J, Keane D. Morphology of atrial myocardium in human pulmonary veins: a postmortem analysis in patients with and without atrial fibrillation. J Am Coll Cardiol. 2003; 42:1108–1114.
14. Saito T, Waki K, Becker AE. Left atrial myocardial extension onto pulmonary veins in humans: anatomic observations relevant for atrial arrhythmias. J Cardiovasc Electrophysiol. 2000; 11:888–894.
15. Tan AY, Li H, Wachsmann-Hogiu S, Chen LS, Chen PS, Fishbein MC. Autonomic innervation and segmental muscular disconnections at the human pulmonary vein-atrial junction: implications for catheter ablation of atrial-pulmonary vein junction. J Am Coll Cardiol. 2006; 48:132–143.
16. McGann CJ, Kholmovski EG, Oakes RS, et al. New magnetic resonance imaging-based method for defining the extent of left atrial wall injury after the ablation of atrial fibrillation. J Am Coll Cardiol. 2008; 52:1263–1271.
17. Kowalski M, Grimes MM, Perez FJ, et al. Histopathologic characterization of chronic radiofrequency ablation lesions for pulmonary vein isolation. J Am Coll Cardiol. 2012; 59:930–938.
18. Ranjan R, Kato R, Zviman MM, et al. Gaps in the ablation line as a potential cause of recovery from electrical isolation and their visualization using MRI. Circ Arrhythm Electrophysiol. 2011; 4:279–286.
19. Wittkampf FH, Hauer RN, Robles de Medina EO. Control of radiofrequency lesion size by power regulation. Circulation. 1989; 80:962–968.
20. Jain MK, Wolf PD. Temperature-controlled and constant-power radio-frequency ablation: what affects lesion growth? IEEE Trans Biomed Eng. 1999; 46:1405–1412.
21. Kautzner J, Neuzil P, Peichl P, et al. Contact force, force time integral and lesion continuity are critical to improve durable PV isolation: EFFICAS II results. Heart Rhythm. 2012; 9:S28.
22. Cabrera JA, Ho SY, Climent V, Fuertes B, Murillo M, Sánchez-Quintana D. Morphological evidence of muscular connections between contiguous pulmonary venous orifices: relevance of the interpulmonary isthmus for catheter ablation in atrial fibrillation. Heart Rhythm. 2009; 6:1192–1198.
23. Andrade JG, Pollak SJ, Monir G, et al. Pulmonary vein isolation using a pace-capture-guided versus an adenosine-guided approach: effect on dormant conduction and long-term freedom from recurrent atrial fibrillation--a prospective study. Circ Arrhythm Electrophysiol. 2013; 6:1103–1108.
24. Sotomi Y, Inoue K, Ito N, et al. Cause of very late recurrence of atrial fibrillation or flutter after catheter ablation for atrial fibrillation. Am J Cardiol. 2013; 111:552–556.
Supplementary Materials
The online-only Data Supplement is available with this article at http://dx.doi.org/10.4070/kcj.2014.44.5.291.