Abstract
Background and Objectives
The cardiac autonomic nervous system is an emerging target for therapeutic control of atrial fibrillation (AF). We evaluated the effects of low-intensity autonomic nerve stimulation (LI-ANS) on atrial electrophysiology, AF vulnerability, and neural remodeling.
Subjects and Methods
Fourteen dogs were subjected to 3 hours rapid atrial pacing (RAP, 5 Hz) and concomitant high frequency LI-ANS (20 Hz, at voltages 40% below the threshold) as follows: no autonomic stimulation (control, n=3); or right cervical vagus nerve (RVN, n=6), anterior right ganglionated plexi (ARGP, n=3), and superior left ganglionated plexi (SLGP, n=2) stimulation. Programmed and burst atrial pacing were performed at baseline and at the end of each hour to determine atrial effective refractory period (ERP), window of vulnerability (WOV), and inducibility of sustained AF.
Results
Atrial ERP was significantly shortened by 3 hours RAP (in control group, ΔERP=-47.9±8.9%, p=0.032), and RAP-induced ERP shortening was attenuated by LI-ANS (in LI-ANS group, ΔERP=-15.4±5.9%, p=0.019; vs. control, p=0.035). Neither WOV for AF nor AF inducibility changed significantly during 3 hours RAP with simultaneous LI-ANS. There was no significant difference between the control and LI-ANS group in nerve density and sprouting evaluated by anti-tyrosine hydroxylase and anti-growth associated protein-43 staining. Among the various sites for LI-ANS, the ARGP-stimulation group showed marginally lower ΔWOV (p=0.077) and lower nerve sprouting (p=0.065) compared to the RVN-stimulation group.
Atrial fibrillation (AF) is the most common cardiac arrhythmia, which increases the risk of hemodynamic impairment and thromboembolic events, resulting in significant morbidity and mortality.1) Anti-arrhythmic drugs, electrical cardioversion, and catheter ablation techniques have been used to treat patients with AF. The clinical outcome is however far from satisfactory, and the long-term recurrence rate has been reported to be over 30%.2) The cardiac autonomic nervous system is known to play an important role in initiating and maintaining atrial tachyarrhythmia,3)4)5)6) and emerging evidence on autonomic modulation underscores its use as a novel treatment modality for AF.7)8) In this study, several sites of the cardiac autonomic nervous system were stimulated at an intensity below the threshold, to evaluate effects on atrial electrophysiology and AF inducibility in a canine AF model.
All animal experiments were approved by the Institutional Animal Care and Use Committee of the Seoul National University, and conducted in an AAALAC-approved animal laboratory. Fourteen male mongrel dogs weighing 20 to 25 kg were used in this study. Animals were randomly assigned to control group (no autonomic nerve stimulation, n=3) or low-intensity autonomic nerve stimulation group (LI-ANS, n=11). The sites for autonomic nerve stimulation were right cervical vagus nerve (RVN, n=6), anterior right ganglionated plexi (ARGP, n=3), and superior left ganglionated plexi (SLGP, n=2).
General anesthesia was induced with Zoletil® 5 mg/kg (combination of tiletamine/zolazepam, Virbac S/A, Carros, France) and maintained with isoflurane. Standard electrocardiography and pulse oximeter oxygen saturation were continuously monitored. Median thoracotomy was performed to expose the canine heart. Two bipolar electrodes (Capsurefix® Novus 5076, Medtronic, Fridley, MN, USA) were attached to the free wall of the right and left atria. A custom designed electrode was used to stimulate ganglionated plexi (generous gifts from Cleveland Clinic, Cleveland, OH, USA). The right cervical vagal trunk was exposed by dissection, and a pair of unipolar electrodes (Streamline temporary myocardial pacing lead, Me-dtronic, Fridley, MN, USA) was inserted into the vagal trunk for low-intensity stimulation in the RVN stimulation group. Electrogram was recorded using LabChart 7 (AD Instruments, Colorado Springs, CO, USA) with filter setting of 0.1 to 250 Hz.
The overall study scheme is presented as Fig. 1. To induce acute atrial remodeling, the lateral wall of the left atrium was rapidly paced at 300 beats/min. According to the assigned experimental group, the designated site of autonomic nervous system was simultaneously stimulated by high-frequency electrical stimulation (20 Hz, 0.2 ms duration, square waves) using a S88 dual channel stimulator (Grass Instrument Co., MA, USA). First, ANS threshold was determined as the lowest voltage inducing any reduction in heart rate at each site {3.1±0.6 V for RVN stimulation, 7.8±3.1 V for ganglionated plexi (GP) stimulation}. Then, autonomic nerves were stimulated at a voltage 40% lower than the threshold during the 3 hours experimental protocol for LI-ANS. After each hour rapid atrial pacing (RAP) was temporarily stopped for 5 to 10 minutes to perform electrophysiological evaluation. The effective refractory period (ERP), window of vulnerability (WOV) for AF, and AF inducibility were measured at the lateral free wall of both left and right atria. ERP was determined by programmed stimulation consisting of 8 consecutive stimuli (S1-S1=400 ms) followed by a premature stimulus (S1-S2). WOV for AF was defined as the difference between the longest and shortest S1-S2 interval at which AF of >3 seconds was induced. AF inducibility was tested by counting the episodes of induced sustained AF (>30 seconds) after 5 burst atrial pacings (10 seconds, 600 beats/min).
After the 3 hours electrophysiological study, ventricular fibrillation was induced by 9 V DC current to sacrifice the animals. Tissues were obtained from both atrial free walls. Immunohistochemical staining was performed for tyrosine hydroxylase (anti-TH antibody, Accurate Chemical & Scientific Corporation, Westbury, NY, USA) and growth-associated protein 43 (anti-GAP43 antibody, Chemicon International Inc., Billerica, MA, USA) using the protocols described elsewhere.9) 5 high power fields from each slide, were randomly chosen and nerve density (anti-TH) or nerve sprouting (anti-GAP43) was automatically determined by the computerized detection of the stained brown color (Image-Pro 4.0).
Data were presented as mean±SE. Student's t-tests were used for comparison between the control and the LI-ANS groups. Parameters were compared among the LI-ANS groups using analysis of variance (ANOVA). Paired t-test was used for comparison between the baseline and post-RAP electrophysiological parameters such as ERP and WOV. Values of p<0.05 were considered statistically significant.
In the control group (no autonomic stimulation), atrial ERP was shortened from 101.7±15.8 ms at the baseline state to 80.0±19.1 ms after 1 hour RAP (p=0.006). As the pacing continued, atrial ERP was shortened to 68.3±21.0 ms at the end of the 2nd hour (vs. baseline, p=0.061), and reached 47.5±4.8 ms at 3 hours of RAP (vs. baseline, p=0.032). With concomitant LI-ANS (n=11), RAP progressively shortened atrial ERP from the basal state to the end of 1 hour and 3 hours of RAP: 79.5±5.5 ms, 75.7±4.6 ms (p=0.345), and 65.5±5.7 ms (p=0.019) respectively (Fig. 2A). The percent shortening of atrial ERP induced by RAP was significantly attenuated by concomitant LI-ANS after 3 hours of stimulation (control vs. LI-ANS group, -47.9±8.9% vs. -15.4±5.9%; p=0.035) (Fig. 2B).
During the 3 hours of RAP, WOV for AF did not significantly ch-ange in both control and LI-ANS group (Fig. 3). At 3 hours of RAP with LI-ANS, ΔWOV was 4.8±6.8 ms (vs. baseline, p=0.489) and it was not significantly different from the ΔWOV of the control group (p=0.770). Inducibility of sustained AF >30 seconds did not change significantly during 3 hours of pacing. AF inducibility from baseline to the end of 3 hours RAP was sequentially 0%, 3%, 3%, and 0% in the control group, and 7%, 4%, 4%, and 8% in the LI-ANS group. ΔAF inducibility during the 3 hours stimulation was not significantly different between the control and LI-ANS group (p=0.948).
After 3 hours RAP, the nerve density of the atrium represented as the area positive for TH staining was not significantly different between the control vs. the LI-ANS groups (control vs. LI-ANS group, 6947±1321 µm2/mm2 vs. 6115±538 µm2/mm2; p=0.502). The nerve sprouting defined as the area positive for GAP-43 staining likewise was unchanged in LI-ANS during the 3 hours RAP (control vs. LI-ANS group, 14660±1103 µm2/mm2 vs. 13115±738 µm2/mm2; p=0.313) (Fig. 4). Both the nerve density and the nerve sprouting were significantly higher in the right atrium than the left atrium (nerve density: right vs. left, 8804±804 µm2/mm2 vs. 3783±454 µm2/mm2; p<0.001, nerve sprouting: right vs. left, 17043±901 µm2/mm2 vs. 9849±628 µm2/mm2; p<0.001), regardless of the autonomic stimulation.
Among the various sites for LI-ANS, the degree of atrial ERP percent shortening by 3 hours RAP was in the order of RVN (ΔERP=-19.0±9.2%, vs. baseline, p=0.065), ARGP (ΔERP=-15.0±11.1%, vs. baseline, p=0.234), and SLGP (ΔERP=-5.2±8.3 ms vs. baseline, p=0.576). There was no significant difference in ERP shortening among the LI-ANS subgroups (ANOVA, p=0.172). Low intensity GP stimulation tended to attenuate ERP shortening induced by RAP (ARGP stimulation vs. control group, p=0.067; SLGP stimulation vs. control group, p=0.013). Low-intensity stimulation on ARGP alone resulted in reduction of WOV during 3 hours pacing, albeit not significantly (ΔWOV=-14.2±10.4 ms, vs. control, p=0.236). LI-ANS on ARGP sh-owed lower ΔWOV with borderline significance compared to stimulation on RVN (p=0.077) (Fig. 5).
There was no significant difference in neural remodeling, as measured by nerve density and nerve sprouting among the sites for LI-ANS (ANOVA: p=0.790 for nerve density, and p=0.145 for nerve sp-routing). However, nerve sprouting tended to be lower in the ARGP-stimulation group than in the RVN-stimulation group (p=0.065) (Table 1).
The effect of LI-ANS on atrial electrical physiology and neural remodeling was evaluated. The acute canine model for AF, established by Wijffels et al.10) based on the concept of "AF begets AF", was adopted. Rapid pacing for 3 hours induced electrical remodeling of the canine atria and shortened the atrial ERP, which was significantly attenuated by LI-ANS. Neither WOV nor AF inducibility changed significantly during this 3 hours study protocol, regardless of the concomitant LI-ANS. Neural remodeling represented by sympathetic hyperinnervation or nerve sprouting, are characteristic changes in the chronic canine model for AF.11) Neural remodeling was not significantly different between the control and LI-ANS group in our acute study. Among the sites for LI-ANS, it is notable that the ARGP-stimulation group showed marginally lower ΔWOV and nerve sprouting compared to RVN-stimulation group. These results suggest that ARGP-simulation may be more favorable than RVN-stimulation for the treatment of AF.
As mentioned above, the current treatment strategy for AF has discrete limitations and consequently, there is a growing need for new treatment options. With accumulating evidence on the close relationship between the cardiac autonomic nervous system and AF,3)4)5) autonomic modulation has been explored as a novel treatment modality for AF. In a canine heart failure model, sympathetic blockade using cryoablation of stellate ganglia showed reduction of paroxysmal AF induced by sympathetic discharges.12) In other animal studies, ablation of GP suppressed AF induction.7)13) A few clinical studies also suggested that GP-ablation may improve the effectiveness of conventional catheter-based pulmonary vein isolation therapy.14)15) In addition, Schauerte et al.16) showed that the ablation of post-ganglionic parasympathetic fibers abolished vagally mediated AF. In the current study, autonomic modulation by LI-ANS was tested in the setting of 3 hours RAP. The results provided additional evidence supporting LI-ANS as a plausible therapeutic modality for AF.
In an effort to modulate autonomic nerves, low-level nerve stimulation has been previously explored. Vagal nerve stimulation is known to be arrhythmogenic and was used to induce AF in numerous experimental models.17)18) However, there were indications that the effects of vagal stimulation varied according to the intensity of stimulation,19) Zhang et al.3) demonstrated that the vagal stimulation at moderate intensity (≤40% prolongation of sinus cycle length) did not increase the arrhythmogenic risk. Furthermore, vagal stimulation at an intensity below the threshold was shown to reduce the incidence of paroxysmal AF in ambulatory dogs by suppressing the stellate ganglion nerve activity.8) Another group reported that low-level vagal nerve stimulation might reverse atrial remodeling induced by RAP.20) We also demonstrated in the present study, that sub-threshold GP- and VN-stimulation attenuated RAP-induced ERP shortening during the 3 hours study protocol.
Despite indisputable evidence that pre-ganglionic vagal nerve stimulation induces AF, there have been few attempts to block the vagal trunk with the purpose of treating AF. Consequential extra-cardiac symptoms accompanying vagal blocks owing to its wide innervation, may be a deciding factor. Low-intensity stimulation is no exception, and extra-cardiac symptoms such as nausea, drooling, and coughing were frequently observed in dogs under low-level vagal stimulation.8) Furthermore, two thirds of the vagus nerve is comprised of afferent sensory fibers, which may have inhibitory feedback effects on low intensity stimulation.21)
Ganglionated plexi, on the other hand, constitutes the intrinsic cardiac autonomic nervous system, and is more cardioselective and cholinergic dominant.22) Nerve activities at GPs were correlated with initiation of AF in several studies,23) and the cardiac effects of GP-stimulation (post-ganglionic stimulation) was shown to be similar to those of VN-stimulation (pre-ganglionic stimulation).24) Although GPs have been targeted for autonomic modulation in several studies,7)25) the effects of sub-threshold GP-stimulation have not been clarified. In the current study, low-intensity GP-stimulation attenuated RAP-induced ERP shortening, similar to low-intensity cervical vagus nerve stimulation. Moreover, ARGP-stimulation resulted in marginally lower ΔWOV than RVN-stimulation at the end of 3 hours RAP (p=0.077), perhaps due to the accompanying lower atrial neuronal sprouting in ARGP-stimulation vs. the RVN-stimulation group (p=0.065). These results, along with the cardio-selectivity of GP, suggest that low-intensity ARGP stimulation may be a better option than low-intensity vagal nerve stimulation for the purpose of cardiac autonomic modulation.
Immunohistochemistry staining was performed to analyze neural remodeling during 3 hours RAP and LI-ANS. Previous studies reported that the nerve density and sprouting were higher in the right atrium than in the left atrium, while they were not significantly different among the sites from the ipsilateral atrium.11) Therefore, lateral free wall tissues from both atria were obtained and analyzed in this study. Higher nerve density and sprouting was observed in the right atrium than in the left atrium. Despite no significant difference between the control and LI-ANS groups, GAP-43 positive density tended to be lower in ARGP-stimulation group than in RVN-stimulation group (p=0.065). Although the 3 hours experimental protocol may not be sufficient for actual nerve sprouting, it is possible that these results reflect actual neural remodeling. GAP-43 is a widely accepted marker for sprouting nerves,26) and rapid redistribution of GAP-43 may predict the future axonal grow-th.27) Furthermore, previous studies demonstrated right-side dominance of the canine GP system, both in anatomic and functional aspects.28)29) These factors might have affected the aforementioned favorable outcomes from sub-threshold ARGP-stimulation.
Although LI-ANS was shown to suppress the electrical remodeling induced by RAP during the 3 hours experimental protocol, direct evidence of anti-arrhythmic effect of LI-ANS could not be provided using this acute model for AF. There were considerable differences between the positively stained areas for anti-TH and anti-GAP-43 antibodies, comparable to previous studies.11)30) Different sensitivities to anti-TH and anti-GAP-43 antibodies may have attributed to this discrepancy. Parasympathetic nerve remodeling was not indicated in this study, because immunostaining for anti-choline acetyl transferase antibody was negative in the atrial myocardium. Inconclusive comparative results between ARGP- vs. RVN-stimulation groups also warrant further studies.
In conclusions, LI-ANS significantly attenuated the shortening of atrial ERP caused by 3 hours of RAP in this canine model. ARGP might be a better target among the sites for LI-ANS, including cervical vagus nerve, for suppressing atrial remodeling in AF.
Figures and Tables
Fig. 1
Study protocol. Diagram of the study protocol. Electrophysiologic study (EPS) was performed at baseline and at the end of each hour of rapid atrial pacing with concomitant low-intensity autonomic stimulation. RVN: right cervical vagus nerve, ARGP: anterior right ganglionated plexi, SLGP: superior left ganglionated plexi.
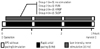
Fig. 2
Change of ERP during 3 hours rapid atrial pacing. RAP significantly shortened atrial ERP, which was attenuated by concomitant LI-ANS. (A) Serial atrial ERP during the study protocol and (B) the percent change of ERP from baseline. *p<0.05, †p<0.10, compared to baseline value. ERP: effective refractory period, LI-ANS: low-intensity autonomic nerve stimulation.
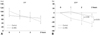
Fig. 3
Change of WOV and inducibility of sustained AF. During 3 hours RAP with LI-ANS, (A) WOV for AF and (B) AF inducibility did not change significantly. WOV: window of vulnerability, AF: atrial fibrillation, LI-ANS: low-intensity autonomic nerve stimulation.
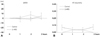
Fig. 4
Neural remodeling after 3 hours RAP. (A) Representative figures of anti-TH staining in the control and (B) LI-ANS group. C: there was no significant difference in sympathetic innervation density. (D) Representative figures of anti-GAP43 staining in the control and (E) LI-ANS group. F: nerve sprouting showed no significant difference between groups. RAP: rapid atrial pacing, TH: tyrosine hydroxylase, LI-ANS: low-intensity-autonomic nerve stimulation, GAP: growth-associated protein.
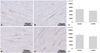
Fig. 5
Changes of ERP and WOV according to the sites for LI-ANS. ΔERP (A) and ΔWOV (B) after 3 hours RAP and LI-ANS according to the sites for LI-ANS. WOV for AF tended to be lower in the ARGP-stimulation group than in RVN-stimulation group at the end of the experimental protocol (p=0.077). ERP: effective refractory period, WOV: window of vulnerability, RAP: rapid atrial pacing, LI-ANS: low-intensity-autonomic nerve stimulation, ARGP: anterior right ganglionated plexi, SLGP: superior left ganglionated plexi.
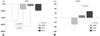
Acknowledgments
This study was supported by grants from the National Research Foundation funded by the Ministry of Education (NRF-2012R1A1A 2006353), and grants from the Seoul National University Hospital Research Fund (0420120350).
References
1. Fuster V, Rydén LE, Cannom DS, et al. 2011 ACCF/AHA/HRS focused updates incorporated into the ACC/AHA/ESC 2006 Guidelines for the management of patients with atrial fibrillation: a report of the American College of Cardiology Foundation/American Heart Association Task Force on Practice Guidelines developed in partnership with the European Society of Cardiology and in collaboration with the European Heart Rhythm Association and the Heart Rhythm Society. J Am Coll Cardiol. 2011; 57:e101–e198.
2. Roy D, Talajic M, Dorian P, et al. Canadian Trial of Atrial Fibrillation Investigators. Amiodarone to prevent recurrence of atrial fibrillation. N Engl J Med. 2000; 342:913–920.
3. Zhang Y, Ilsar I, Sabbah HN, Ben David T, Mazgalev TN. Relationship between right cervical vagus nerve stimulation and atrial fibrillation inducibility: therapeutic intensities do not increase arrhythmogenesis. Heart Rhythm. 2009; 6:244–250.
4. Tai CT, Chiou CW, Chen SA. Interaction between the autonomic nervous system and atrial tachyarrhythmias. J Cardiovasc Electrophysiol. 2002; 13:83–87.
5. Coumel P. Autonomic influences in atrial tachyarrhythmias. J Cardiovasc Electrophysiol. 1996; 7:999–1007.
6. Bettoni M, Zimmermann M. Autonomic tone variations before the onset of paroxysmal atrial fibrillation. Circulation. 2002; 105:2753–2759.
7. Oh S, Zhang Y, Bibevski S, Marrouche NF, Natale A, Mazgalev TN. Vagal denervation and atrial fibrillation inducibility: epicardial fat pad ablation does not have long-term effects. Heart Rhythm. 2006; 3:701–708.
8. Shen MJ, Shinohara T, Park HW, et al. Continuous low-level vagus nerve stimulation reduces stellate ganglion nerve activity and paroxysmal atrial tachyarrhythmias in ambulatory canines. Circulation. 2011; 123:2204–2212.
9. Cao JM, Fishbein MC, Han JB, et al. Relationship between regional cardiac hyperinnervation and ventricular arrhythmia. Circulation. 2000; 101:1960–1969.
10. Wijffels MC, Kirchhof CJ, Dorland R, Power J, Allessie MA. Electrical remodeling due to atrial fibrillation in chronically instrumented conscious goats: roles of neurohumoral changes, ischemia, atrial stretch, and high rate of electrical activation. Circulation. 1997; 96:3710–3720.
11. Chang CM, Wu TJ, Zhou S, et al. Nerve sprouting and sympathetic hyperinnervation in a canine model of atrial fibrillation produced by prolonged right atrial pacing. Circulation. 2001; 103:22–25.
12. Ogawa M, Tan AY, Song J, et al. Cryoablation of stellate ganglia and atrial arrhythmia in ambulatory dogs with pacing-induced heart failure. Heart Rhythm. 2009; 6:1772–1779.
13. Ghias M, Scherlag BJ, Lu Z, et al. The role of ganglionated plexi in apnea-related atrial fibrillation. J Am Coll Cardiol. 2009; 54:2075–2083.
14. Katritsis DG, Giazitzoglou E, Zografos T, Pokushalov E, Po SS, Camm AJ. Rapid pulmonary vein isolation combined with autonomic ganglia modification: a randomized study. Heart Rhythm. 2011; 8:672–678.
15. Pokushalov E, Romanov A, Shugayev P, et al. Selective ganglionated plexi ablation for paroxysmal atrial fibrillation. Heart Rhythm. 2009; 6:1257–1264.
16. Schauerte P, Scherlag BJ, Pitha J, et al. Catheter ablation of cardiac autonomic nerves for prevention of vagal atrial fibrillation. Circulation. 2000; 102:2774–2780.
17. Wakili R, Voigt N, Kääb S, Dobrev D, Nattel S. Recent advances in the molecular pathophysiology of atrial fibrillation. J Clin Invest. 2011; 121:2955–2968.
18. Allessie MA, Boyden PA, Camm AJ, et al. Pathophysiology and prevention of atrial fibrillation. Circulation. 2001; 103:769–777.
19. Oh S. Disease modification by autonomic nerve stimulation. Heart Rhythm. 2012; 9:810–811.
20. Sheng X, Scherlag BJ, Yu L, et al. Prevention and reversal of atrial fibrillation inducibility and autonomic remodeling by low-level vagosympathetic nerve stimulation. J Am Coll Cardiol. 2011; 57:563–571.
21. Prechtl JC, Powley TL. The fiber composition of the abdominal vagus of the rat. Anat Embryol (Berl). 1990; 181:101–115.
22. Hoover DB, Isaacs ER, Jacques F, Hoard JL, Pagé P, Armour JA. Localization of multiple neurotransmitters in surgically derived specimens of human atrial ganglia. Neuroscience. 2009; 164:1170–1179.
23. Choi EK, Shen MJ, Han S, et al. Intrinsic cardiac nerve activity and paroxysmal atrial tachyarrhythmia in ambulatory dogs. Circulation. 2010; 121:2615–2623.
24. Zhang Y, Yamada H, Bibevski S, et al. Chronic atrioventricular nodal vagal stimulation: first evidence for long-term ventricular rate control in canine atrial fibrillation model. Circulation. 2005; 112:2904–2911.
25. Sakamoto S, Schuessler RB, Lee AM, Aziz A, Lall SC, Damiano RJ Jr. Vagal denervation and reinnervation after ablation of ganglionated plexi. J Thorac Cardiovasc Surg. 2010; 139:444–452.
26. Jacobson RD, Virág I, Skene JH. A protein associated with axon growth, GAP-43, is widely distributed and developmentally regulated in rat CNS. J Neurosci. 1986; 6:1843–1855.
27. Goslin K, Banker G. Rapid changes in the distribution of GAP-43 correlate with the expression of neuronal polarity during normal development and under experimental conditions. J Cell Biol. 1990; 110:1319–1331.
28. Yuan BX, Ardell JL, Hopkins DA, Losier AM, Armour JA. Gross and microscopic anatomy of the canine intrinsic cardiac nervous system. Anat Rec. 1994; 239:75–87.
29. Hou Y, Scherlag BJ, Lin J, et al. Ganglionated plexi modulate extrinsic cardiac autonomic nerve input: effects on sinus rate, atrioventricular conduction, refractoriness, and inducibility of atrial fibrillation. J Am Coll Cardiol. 2007; 50:61–68.
30. Tan AY, Zhou S, Ogawa M, et al. Neural mechanisms of paroxysmal atrial fibrillation and paroxysmal atrial tachycardia in ambulatory canines. Circulation. 2008; 118:916–925.