Abstract
Along with the development of innovative stent designs, preclinical trials in animal models are essential. Many animal models have been used and appear to yield comparable results to clinical trials despite substantial criticisms about their validity. Among the animal models, porcine coronary artery models have been the standard models for the preclinical evaluation of endovascular devices. However, rapid growth rate, high body weight potential, and the propensity to develop granulomatous inflammatory reactions are major limitations of the porcine coronary artery model. Compared with porcine coronary artery models, the comparative rabbit iliac artery model has the advantages of being small and easy to handle and relatively inexpensive. Furthermore, the rabbit model has been known to reliably reflect human restenosis histopathologically and have major advantages such as pairwise comparison, which makes each animal serve as its own control subject, therefore, maximizing its statistical power for comparative testing. However, despite the widespread use of this model, a systematic description of the procedure and harvest protocols has never been published. This article describes the surgical procedure, stent implantation procedure, method for tissue harvesting, and how measurements are performed. Although the results of animal models may not perfectly extrapolate to humans, the comparative rabbit iliac artery model may be a useful tool for assessing and comparing the efficacy of new coronary stents with conventional stent systems. This thorough description of the techniques required for vascular access, stent implantation, tissue preparation, and measurement, should aid investigators wishing to begin using the comparative rabbit iliac artery model.
Percutaneous coronary intervention (PCI) for the treatment of coronary artery disease from stable angina to acute coronary syndrome is commonly accompanied with stent implantation. Currently, as of this year, drug-eluting stents (DES) are estimated to have been implanted five million times worldwide. PCI with stent implantation is the most frequently performed medical intervention.1) Coronary stents have proven their value in the management of coronary artery disease, as a supplement to balloon angioplasty, countering the elastic recoil and constriction of the artery.2)3) However, significant neointimal proliferation reactions, leading to in-stent restenosis, allergic and inflammatory reactions to the polymers, and incomplete endothelialization over the stent struts, lead to lethal complications like stent thrombosis, which have become an Achilles heel for DES.4-6) Along with the clinical comorbidities of the patient, well-known risk factors including restenosis are also attributable to the stent itself, for example, stent design, stent materials, stent surface condition, and drug delivery devices, including coated polymers.5-7) In addition, the inherent characteristics of coronary stents are also known to play a role in the development of stent thrombosis.8) Although newer generations of coronary stents with biocompatible or bioabsorbable polymers have been introduced and evaluated, none of these technologies have completely overcome the weakness of DES.9)10) This problem has persistently encouraged the development of coronary stents using innovative technologies such as antibody-coated cell capturing stents, biodegradable stents, or dual polymer coated stents.11) In order to assess the actual potential of new coronary stents with innovative technologies, preclinical animal studies need to be as rigorous as possible and eliminate as many confounding factors as possible, so as to isolate the feature of the stent alone for comparison.12)13) To date, injured normal animal models (rat, pig, mouse, dog, rabbit, primate) have become standard for understanding neointima and vascular remodeling, and they rapidly evolved into a new role, that of testing novel restenosis therapies.14) Among these animal models, the hypercholesterolemic comparative rabbit iliac artery model has become known to reliably reflect human restenosis histopathologically and also have major advantages such as pairwise comparison, which makes each animal serve as its own control subject, thereby maximizing statistical power for comparative testing.13)14) However, despite the widespread use of this model, a systematic description of the procedure and harvest protocols has never been published. The literature only provides a brief description of the model and a detailed methodological description of the model has not been developed to date.15-17) This article seeks to present a detailed description of the procedure with comprehensive instructions for generating the model.
For the comparative rabbit iliac artery model to evaluate coronary stents, Male New Zealand White rabbits (3.0 to 3.5 kg; Yonam Laboratory Animals, Cheonan, Korea) were fed a 1% cholesterol diet (Oriental Yeast Co. Ltd., Tokyo, Japan) and oral aspirin (2 mg/kg) for 2 weeks prior to stent implantation. The efficacy of the 1% cholesterol diet for 2 to 5 weeks on the induction of hypercholesterolemia has been well validated.14) Cho et al.18) showed serum cholesterol levels of 824±165 mg/dL after 2 weeks on a 1% cholesterol diet. In addition, Van Dyck et al.19) reported that the mean total blood cholesterol level at baseline was 48±10 mg/dL, and peaked at 2087±624 mg/dL at 5 weeks after initiation of the 1% cholesterol diet. The body weight of the animals should be 3.0 to 3.5 kg, since an adequate diameter of the carotid artery is necessary to insert the 4-5 Fr femoral sheath without tearing the artery. All animals were cared for under constant temperature (22℃) and humidity (65%) conditions. The animal experiments were approved and performed according to the Institutional Animal Care and Use Committee (IACUC) of the Clinical Research Institute at the Seoul National University Hospital and complied with the National Research Council's Guidelines for the Care and Use of Laboratory Animals.
Several drugs may be used for general anesthesia, and their detailed dosages are presented in Table 1.17)20) Tiletamine, components of the Zoletil, have been known to be potential nephrotoxic agents with dosages of 32-64 mg/kg intramuscular route, and intermediate dose of 15 mg/kg were used. The intranasal route can also be used to avoid possible nephrotoxicity.17)21) The lateral side of the thigh was preferred for the intramuscular injection of these drugs. In order to prevent peri-procedural bacteremia, cefazolin (25 mg/kg) was injected intramuscularly. During general anesthesia and the procedure, the maintenance of the body temperature at 37℃ using a heating blanket is crucial for the survival of the animals. For local anesthesia, lidocaine solution (2%) was administered subcutaneously at the incision site. Continuous electrocardiographic monitoring and supplemental oxygen using an oxygen mask were necessary.
After fasting for 8 hours, the animals were anesthetized with medication, as described above (Table 1) and then fixed on the operating table in the supine position. Topical 10% povidone-iodine was used to sterilize the surgical field. For local anesthesia, a 2% lidocaine solution was injected subcutaneously. Since even a small amount of lidocaine can be lethal if the solution is unintentionally infused into the blood circulation, regurgitation was essential prior to injection (Fig. 1A and B). A lateral skin incision, approximately 5 cm in length, was performed 0.5-1 cm from the midline and meticulous bleeding control was performed with an electronic coagulator or manual compression (Fig. 1C). After dissection of subcutaneous tissues, the internal jugular vein was visible 1-1.5 cm on the lateral side from the tracheal ring (Fig. 1D and E). Extreme caution was required not to injure the internal jugular vein. In our experience, an animal with an injured internal jugular vein resulted in a poor outcome after the procedure. In order to expose the carotid artery, an incision was made in the space between the tracheal ring and the internal jugular vein (Fig. 1E and F). If a sufficient incision is made in the space between the tracheal ring and internal jugular vein, the carotid artery along with the vagus nerve are exposed (Fig. 1F). The perivascular tissue should be sufficiently dissected; otherwise, the insertion of the 4 Fr introducer might be precluded due to the presence of perivascular tissue (Fig. 2A and B). After sufficient dissection and isolation of the carotid artery, 3-0 black silk was tied at the most distal (cephalic) part of the carotid artery in order to dilate the carotid artery, thereby making it easy to puncture (Fig. 2C and D). Then, two more 3-0 black silks were temporarily anchored at the most proximal (caudal) part of the carotid artery. These two black silks will be used to ligate the carotid artery after removal of the introducer sheath (Fig. 2E and F). In addition, the pulling up of these black silks will prevent hemorrhage during the change of the 22 G angiosheath to 4 Fr introducer sheath. The 22 G angiosheath was preferred for the puncture of the carotid artery. A 24 G angiosheath is too small and a 4 Fr introducer sheath is difficult to advance through the punctured hole, while a 20 G angiosheath is too large and laceration or perforation of the carotid artery is common, resulting in lethal consequences. The bevel of the needle should be faced downward to prevent any puncture of the posterior wall of the carotid artery (Fig. 3A). The most common mistake, resulting in a puncture, is facing the bevel in the upward direction. In the bevel upward case, blood usually spurts out after the puncture and visualization of the field is difficult. All of these factors raise the risk of posterior puncture. After the successful anterior puncture of the arterial wall was made, angiosheath was advanced gently and a 0.014 inch coronary guide wire (Runthrough NS™, Terumo, Tokyo, Japan) was advanced through the lumen of the angiosheath and carotid artery (Fig. 3B). When removing the angiosheath, the pulling up of the distally anchored 3-0 black silk will reduce hemorrhage from the punctured arterial wall (Fig. 3C). A 4 Fr introducer sheath (short femoral sheath is preferable; Pinnacle TIF Tip™, Terumo, Tokyo, Japan) was gently advanced through the 0.014 inch coronary guide wire into the punctured arterial wall (Fig. 3D and E). This is the most crucial step, since perivascular tissues may become entangled and subsequently prevent the advance of the introducer sheath. The sufficient dissection of perivascular tissues and careful inspection of the puncture hole in the anterior arterial wall when advancing the sheath are the most important steps. Approximately 3-4 cm of an introducer sheath was advanced and sutured to the skin to prevent the unintentional removal of the sheath (Fig. 3F). Any side of the carotid artery could be used for the initial angiogram and implantation of stents. The surgical exposure of the carotid artery and stent implantation was performed under sterile conditions.
Prior to the insertion of the guide wire, heparin (100 IU/kg) was injected intra-arterially through the introducer sheath to prevent thrombus formation. Under fluoroscopic guiding (Philips, BV-Pulsera, Eindhoven, the Netherlands), the 0.014 inch coronary guide wire was advanced from the carotid artery into the distal descending aorta (Fig. 4A). When the right carotid artery was used as the access route, the tip of the wire should be shaped as per the alphabet letter "J" to cross the aortic arch and advance into the descending aorta. The guide wire was then advanced to the proximal portion of the femoral artery (Fig. 4B). There are two important details that enhance the success rate of the procedure, which should be highlighted. First, the gentle advance of the guide wire is crucial, since even minimal trauma to the aortic wall from the guide wire is able to cause aortic dissection, pseudoaneurysm, or a rupture of the wall. Second, the advance of the guide wire above the level of the distal femur should be avoided to prevent any rupture of the artery. In the next step of the procedure, the stent delivery system was advanced to the distal portion of the iliac artery, just beyond the bifurcation of the abdominal aorta, and the stents were deployed with a pressure of 8-12 atm, according to the reference vessel diameter and stent size (Fig. 4B and C). The final angiograms confirmed successful stent implantation (Fig. 4D). Two distally anchored black silks were tied upon the removal of the introducer sheath. The muscle fascia and dermal layer were then sutured with a 3-0 black silk.
During the entire post-procedural period, the hypercholesterolemia diet and oral aspirin (2 mg/kg) was given. At prespecified time points according to the evaluation modalities {scanning electron microscopy (SEM) or histologic evaluation}, follow-up angiography was performed through the opposite side of the carotid artery. Generally, the procedure with the opposite side of the artery was the same as for the initial procedure.
If the left carotid artery was used for the follow-up procedure, a 4 Fr introducer sheath was advanced into the descending aorta to allow for easier advance of the guide wire into the common iliac artery.22)
According to the literature available, a variety of evaluations regarding the efficacy and safety of experimental stents have been reported. Ultimately, the most important part of the evaluation is the assessment of early re-endothelialization and delayed neointimal hyperplasia, regardless of the evaluation modality. We used SEM and percent covered stent surface for the assessment of early re-endothelialization. Conversely, resin fixation of harvested samples and histologic examinations were used for the assessment of delayed neointimal hyperplasia. After a follow-up angiography was performed, the animal was sacrificed with 20 mL of potassium chloride through direct intracardiac injection. The inferior vena cava was punctured with 16 G angiosheath and continuous phlebotomy was performed along with the infusion of normal saline through a carotid sheath. Meticulous retroperitoneal dissection and exposure of both iliac arteries were necessary prior to the cutting off of the arteries. The evaluation of re-endothelialization was performed with SEM after 1 hour, 24 hours, and 3 days. Harvested stents were fixed in 2.5% glutaraldehyde and processed for SEM. To prevent detachment of the stent struts from the arterial wall and to achieve sufficient visualization of the luminal surface of harvested vessels, one quarter of the stent circumference was removed longitudinally. As a result, we were able to look inside through this window (Fig. 5A-D) and see the luminal surface and the cellular coverage of the entire stent struts, so they could be evaluated qualitatively or quantitatively. Quantitative analysis of covered stent struts was performed by the measurement of the covered surface using a digital image-analysis system (Image-Pro Plus version 4.5, MediaCybernetics, MD, USA) (Fig. 5C). Samples for the assessment of neointimal hyperplasia were harvested 6 weeks after stent implantation. After the animal was sacrificed with a potassium-chloride solution, a 4% neutral-buffered formalin solution was infused through a carotid introducer sheath at 120 mm Hg for 10-30 minutes. Simultaneously, phlebotomy with a puncture of the inferior vena cava was performed. Except for the use of perfusion fixation with a 4% neutral-buffered formalin solution in the delayed harvest protocol, the surgical procedure for early harvest is the same as for the delayed harvest protocol. In the early harvest protocol, pressure fixation might remove endothelial layers that are minimally attached. Therefore, perfusion fixation should be avoided in the early harvest procedure. The specimens were embedded in cold-polymerizing methylmethacrylate to preserve stent and vessel integrity. Cross-sectional panes were cut from the proximal, middle, and distal in-stent sections with a low-speed tungsten blade, leaving the stent struts intact and minimizing arterial deformation.20)22-24) The samples were stained with hematoxylin and eosin for histomorphological analysis (Fig. 5E). Cross-sectional images of stented rabbit iliac arteries were loaded onto Image-Pro Plus version 4.5 (MediaCybernetics), and the neointimal area was measured by a blinded observer. The luminal area, defined as the area circumscribed by the endothelial layer and the internal elastic membrane area, defined as the area circumscribed by the internal elastic membrane, were determined to calculate the neointimal area.20) The neointima area=internal elastic membrane area-luminal area (Fig. 5F).23) In order to assess late luminal loss for both stents groups, quantitative coronary angiography was used, as previously reported.20)
We have thoroughly described in detail the method of generating the comparative rabbit iliac artery model for the comparison of experimental stents with conventional stents. Since there has not been a detailed field manual published for the rabbit model, we have described every step of the procedure in as much detail as possible in order for beginners to be able to follow the steps. Research in human coronary atherosclerosis and the evaluation of coronary stents are limited by the inability to control experiments, by slow temporal lesion development, and by the monetary cost required to conduct randomized controlled trials. Many animal models have been used and appear to yield comparable results to clinical trials despite substantial criticisms about their validity.12) The purpose of animal models can be classified into three categories; the arterial response to injury model (pathogenesis model), safety studies to evaluate the suitability of new medical devices to humans, and efficacy studies to evaluate the performance of new medical devices. Among these models, the comparative rabbit iliac artery model is appropriate for efficacy studies to evaluate the performance of new medical devices. Safety studies require a closer alignment with human stenting and none of the animal models to date have been validated for this purpose. The comparative rabbit iliac artery model has major advantages for the evaluation of new coronary stents in comparison with conventional coronary stents.13) First, arterial dilatation and arterial wall aggregation can be controlled simultaneously. The operator can observe the other side of the artery during the implantation of the stent on one side of the artery; therefore, the stent-to-arterial ratio can be equalized by visual estimation, quantitative coronary angiography, or intravascular ultrasound. Second, all of the animals provide paired parameters and this means each animal serves as its own control subject. This removes the possibility of inter-subject variations which can causes bias in a comparison, particularly where the samples being compared are small in size.13) In addition, intra-subject variations are known to be far fewer compared to inter-subject variations. Third, the statistical power of pairwise. comparison is much higher compared to the comparison of two independent groups. Lastly, rabbit iliac arteries have similar dimensions compared with human coronary arteries,25) and flow dynamics of rabbit iliac arteries are similar to porcine coronary arteries,26) which have been the standard model for the preclinical evaluation of endovascular devices.27) Conversely, the major shortcomings of rabbit stent implantation model should be considered. Foremost is the inability to directly assess cardiac events following stent implantation. Second, although arterial responses are similar, the susceptibility of the elastic-natured aorta and iliac artery to injury is less than muscular coronary artery.28) These discrepancies are able to affect the degree of neointimal hyperplasia, thrombus formation, and inflammatory response, each of which might be less in the rabbit model compared with the porcine coronary artery model.27) Compared with the rabbit model, the porcine coronary artery model has a similar cardiovascular anatomy to humans with regard to size, morphology, collateral circulation, and well-developed vasa vasorum. In addition, this model also possesses similar general physiology and coagulation systems with humans.29) Furthermore, local flow dynamics of porcine coronary arteries closely parallels that of humans, and the neointimal response shows similar histology to that in stenotic human coronary arteries. However, rapid growth rate, high body weight potential, and the propensity to develop granulomatous inflammatory reactions, to date considered unique to this species, are major limitation of the porcine coronary artery model.27) Although the results of animal models may not perfectly extrapolate to humans, the comparative rabbit iliac artery model may be a useful tool for assessing and comparing the efficacy of new coronary stents with conventional stent systems. Appropriate model selection, understanding of model limitations, and appropriate interpretation of the study results in light of model limitations are carefully considered to derive the maximum benefit from preclinical investigations into the safety and efficacy of endovascular devices.
We have described in detail the method for generating the rabbit coronary stent implantation model and the preparation of samples for the appropriate measurement of early re-endothelialization and neointimal hyperplasia. This thorough description of the techniques required for vascular access, stent implantation, tissue preparation, and measurement, should aid investigators wishing to begin using the comparative rabbit iliac artery model, particularly if they have little prior experience doing so.
Figures and Tables
Fig. 1
Dissection of the right carotid artery for vascular access. Before the entire procedure commences, the midline of the rabbit should be marked (A). After local anesthesia with lidocaine solution (B), a lateral incision was made 0.5-1 cm from the midline (C). The internal jugular vein was exposed after the subcutaneous fascia was dissected (D and E, arrowhead). The arrow indicates the imaginary dissection plane to expose the carotid artery (E). The right carotid artery and vagus nerve were exposed through a dissection of the medial side of the internal jugular vein (F, arrowhead).
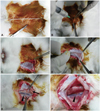
Fig. 2
Mobilization of the carotid artery. The carotid artery was mobilized using blunt dissection is (A and B). The distal vessel was ligated to dilate the carotid artery and make for an easy puncture (C and D). Two loose sutures were placed around the proximal vessel (E and F).
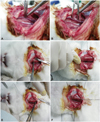
Fig. 3
Puncture technique and insertion of the introducer sheath. The carotid artery was punctured with 22 G angiosheath with the bevel facing downward (A). During the removal of the angiosheath, the proximal suture was pulled upward to prevent arterial bleeding (C, arrowhead). The 4 Fr introducer sheath was gently advanced through the 0.014 inch coronary guide wire into the punctured arterial wall (D and E), and subsequently secured with a tagging suture (F).
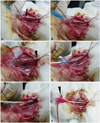
Fig. 4
Stent implantation and angiograms. Once the introducer sheath was inserted, heparin was injected intra-arterially through the introducer sheath to prevent thrombus formation. The 0.014 inch coronary guide wire was advanced from the carotid artery into the distal descending aorta (A). The guide wire never advanced above the level of the distal femur, to prevent perforation of the distal iliac artery (B and C). The final angiogram shows the successful implantation of stents in both iliac arteries with a stent-to-artery-size ratio range of 1.1-1.2 (D).
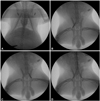
Fig. 5
Assessment of the early re-endothelialization and neointimal area. Scanning the electron microscopic image of a 72-hour harvest sample of anti VE-cadherin antibody coated stents (A). The surface of the bare metal stent and anti VE-cadherin antibody coated stents are shown 3 days after implantation (B and D, respectively). The covered stent surface was measured and quantitatively analyzed (C). A representative hematoxylin and eosin stained sample harvested 6 weeks after implantation (E). The neointimal area was measured by subtracting area 2 (luminal area) from area 1 (internal elastic membrane area) (F).
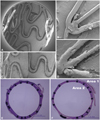
Acknowledgments
This research was supported by the Bio & Medical Technology Development Program of the National Research Foundation (NRF) funded by the Korean government (2010-0020258).
References
1. Simsek C, Serruys PW. Developments in coronary artery stenting: primum non nocere. Panminerva Med. 2011; 53:19–30.
2. Fischman DL, Leon MB, Baim DS, et al. Stent Restenosis Study Investigators. A randomized comparison of coronary-stent placement and balloon angioplasty in the treatment of coronary artery disease. N Engl J Med. 1994; 331:496–501.
3. Serruys PW, van Hout B, Bonnier H, et al. Randomised comparison of implantation of heparin-coated stents with balloon angioplasty in selected patients with coronary artery disease (benestent II). Lancet. 1998; 352:673–681.
4. Cook S, Ladich E, Nakazawa G, et al. Correlation of intravascular ultrasound findings with histopathological analysis of thrombus aspirates in patients with very late drug-eluting stent thrombosis. Circulation. 2009; 120:391–399.
5. Finn AV, Kolodgie FD, Harnek J, et al. Differential response of delayed healing and persistent inflammation at sites of overlapping sirolimusor paclitaxel-eluting stents. Circulation. 2005; 112:270–278.
6. Mauri L, Hsieh WH, Massaro JM, Ho KK, D'Agostino R, Cutlip DE. Stent thrombosis in randomized clinical trials of drug-eluting stents. N Engl J Med. 2007; 356:1020–1029.
7. Rogers C, Edelman ER. Endovascular stent design dictates experimental restenosis and thrombosis. Circulation. 1995; 91:2995–3001.
8. Carter AJ, Aggarwal M, Kopia GA, et al. Long-term effects of polymer-based, slow-release, sirolimus-eluting stents in a porcine coronary model. Cardiovasc Res. 2004; 63:617–624.
9. Serruys PW, Kutryk MJ, Ong AT. Coronary-artery stents. N Engl J Med. 2006; 354:483–495.
10. Akin I, Schneider H, Ince H, et al. Second- and third-generation drug-eluting coronary stents: progress and safety. Herz. 2011; 36:190–196.
11. Garg S, Serruys PW. Coronary stents: looking forward. J Am Coll Cardiol. 2010; 56:10 suppl. S43–S78.
12. Touchard AG, Schwartz RS. Preclinical restenosis models: challenges and successes. Toxicol Pathol. 2006; 34:11–18.
13. Robert R, Rioufol G, Finet G, et al. Experimental assessment of new stent technologies: validation of a comparative paired rabbit iliac artery study model. J Biomed Mater Res B Appl Biomater. 2004; 70:303–310.
14. Schwartz RS, Chronos NA, Virmani R. Preclinical restenosis models and drug-eluting stents: still important, still much to learn. J Am Coll Cardiol. 2004; 44:1373–1385.
15. Nakamura T, Winsor-Hines D, Yin X, et al. Vasomotor function and re-endothelialisation after implantation of biodegradable abluminal polymer coated paclitaxel-eluting stents in rabbit iliac arteries: a time-course study. EuroIntervention. 2012; 8:493–500.
16. Nakazawa G, Nakano M, Otsuka F, et al. Evaluation of polymer-based comparator drug-eluting stents using a rabbit model of iliac artery atherosclerosis. Circ Cardiovasc Interv. 2011; 4:38–46.
17. Doerning BJ, Brammer DW, Chrisp CE, Rush HG. Nephrotoxicity of tiletamine in New Zealand white rabbits. Lab Anim Sci. 1992; 42:267–269.
18. Cho HJ, Kim HS, Lee MM, et al. Mobilized endothelial progenitor cells by granulocyte-macrophage colony-stimulating factor accelerate reendothelialization and reduce vascular inflammation after intravascular radiation. Circulation. 2003; 108:2918–2925.
19. Van Dyck CJ, Hoymans VY, Bult H, et al. Resolute and Xience V polymer-based drug-eluting stents compared in an atherosclerotic rabbit double injury model. Catheter Cardiovasc Interv. 2013; 81:E259–E268.
20. Sommer CM, Grenacher L, Stampfl U, et al. Impact of stent design on in-stent stenosis in a rabbit iliac artery model. Cardiovasc Intervent Radiol. 2010; 33:565–575.
21. Robertson SA, Eberhart S. Efficacy of the intranasal route for administration of anesthetic agents to adult rabbits. Lab Anim Sci. 1994; 44:159–165.
22. Che HL, Bae IH, Lim KS, et al. Suppression of post-angioplasty restenosis with an Akt1 siRNA-embedded coronary stent in a rabbit model. Biomaterials. 2012; 33:8548–8556.
23. Lim WH, Seo WW, Choe W, et al. Stent coated with antibody against vascular endothelial-cadherin captures endothelial progenitor cells, accelerates re-endothelialization, and reduces neointimal formation. Arterioscler Thromb Vasc Biol. 2011; 31:2798–2805.
24. Lee JM, Choe W, Kim BK, et al. Comparison of endothelialization and neointimal formation with stents coated with antibodies against CD34 and vascular endothelial-cadherin. Biomaterials. 2012; 33:8917–8927.
25. Van Belle E, Tio FO, Couffinhal T, Maillard L, Passeri J, Isner JM. Stent endothelialization. Time course, impact of local catheter delivery, feasibility of recombinant protein administration, and response to cytokine expedition. Circulation. 1997; 95:438–448.
26. Bayes-Genis A, Kantor B, Keelan PC, et al. Restenosis and hyperplasia: Animal Models. Curr Interv Cardiol Rep. 2000; 2:303–308.
27. Perkins LE. Preclinical models of restenosis and their application in the evaluation of drug-eluting stent systems. Vet Pathol. 2010; 47:58–76.
28. Asada Y, Kisanuki A, Tsuneyoshi A, Marutsuka K, Hatakeyama K, Sumiyoshi A. Effects of inflation pressure of balloon catheter on vascular injuries and subsequent development of intimal hyperplasia in rabbit aorta. Atherosclerosis. 1996; 121:45–53.
29. Lowe HC, Schwartz RS, Mac Neill BD, et al. The porcine coronary model of in-stent restenosis: current status in the era of drug-eluting stents. Catheter Cardiovasc Interv. 2003; 60:515–523.