Abstract
Background and Objectives
We evaluated the utility of two-dimensional (2D) and three-dimensional (3D) left ventricular (LV) global myocardial deformity parameters for assessing LV diastolic function by comparing invasive measures of LV performance.
Subjects and Methods
Echocardiography and LV pressure were assessed in 39 patients. Myocardial LV longitudinal, circumferential, and radial deformations, as well as area strain, were evaluated utilizing 2D and 3D speckle tracking software. The 2D early diastolic strain rate (2D-SRe) was measured from the 3 apical and 3 short axis views. The 3D diastolic index (3D-DI) was calculated by the % change of global strain during the first one-third of the diastolic period. LV end diastolic pressure (LVEDP) and the rate of LV pressure change (dP/dt) were collected using a pressure-conducted catheter and tau was calculated.
Results
dP/dtmin were related to early mitral annular velocity (e'), 2D-SRelong, 2D-SReradial, as well as 3D-DIlong, and 3D-DIas. Additionally, LVEDP was associated with the ratio of mitral early diastolic velocity (E) to 2D-SRelong, 2D-SRecirc, 2D-SReradial, 3D-DIlong, 3D-DIcirc, and 3D-DIas. E/2D-SRelong, E/2D-SReradial, E/3D-DIlong, and E/3D-DIas were comparable with E/e' in predicting patients with elevated LVEDP. Among those patients with E/e' of 8 to 15, E/3D-DIlong provided incremental value in identifying those with LVEDP ≥15 mm Hg.
Conclusion
2D-SRelong, 2D-SReradial, 3D-DIlong, and 3D-DIas were related to LV relaxation, and the ratios of E to those parameters were associated with LVEDP. In addition, among patients with indeterminate E/e', E/3D-DIlong offered incremental value in predicting elevated LVEDP, suggesting it may provide supplementary information in the evaluation of LV diastolic function.
Left ventricular (LV) diastolic function plays an important role in evaluating patients with various cardiac diseases, and provides a predictive value for potential future cardiovascular outcomes.1-4) The assessment of LV diastolic function is necessary in a routine echocardiographic exam, and Doppler echocardiography has been widely used to assess LV diastolic function non-invasively.5)6) However, there are several drawbacks that limit the use of these conventional methods in clinical settings. Newly-developed speckle tracking methods enable the assessment of regional and global LV function in more detail, while the utility of these myocardial deformity measurements in assessing diastolic function is yet to be fully established.7)8) We hypothesized that myocardial deformity measurements can provide additional information for the assessment of LV diastolic function. To test this hypothesis, we compared myocardial deformity measurements with invasively measured LV pressure and LV pressure-derived diastolic parameters using pressure wire.
Thirty seven consecutive patients who had undergone cardiac catheterization were enrolled. Hemodynamic data were additionally obtained using the pressure wire in those patients who had undergone a coronary angiogram with a variety of clinical indications and who had provided informed consent. Echocardiography was performed within 24 hours of catheterization. Those patients with atrial fibrillation or other significant arrhythmia, suboptimal echocardiographic window, or pressure recording were excluded. This study was approved by an institutional review committee of the Inha University Hospital.
Baseline transthoracic echocardiograms were performed with a frame rate of 40-60 Hz. Patients with insufficient two-dimensional (2D) image quality for the following analyses were excluded at this stage. Standard echocardiographic parameters, including LV volumes and ejection fraction (EF), peak early (E) and late (A) mitral inflow velocities, deceleration time of early mitral flow velocity (DT), and early mitral annular velocity (e') at the septal annular site were analyzed at an offline analysis workstation. 2D and 3D speckle tracking (Artida TM®, Tokyo, Toshiba) were utilized to measure LV myocardial longitudinal, circumferential, and radial deformation, as well as area strain. For 2D speckle tracking measurements, the software automatically tracked the motion through the cardiac cycle after tracing the subendocardium of the LV manually. Tracking quality was verified for each segment with manual adjustment as required. 2D LV global early diastolic longitudinal strain rate (2D-SRelong) was averaged from the apical 4 chamber, 2 chamber, and long axis views, and circumferential (2D-SRecirc) and radial strain rates (2D-SReradial) were averaged from the 3 parasternal short axis views at the level of the mitral valve, papillary muscle, and apex. In addition, three-dimensional (3D) full volume images were acquired with a volume rate of 20-30 volumes/second and 3D LV global longitudinal, circumferential, radial, and area strains were assessed through 3D wall motion tracking. After adjusting the orientation of 2D planes and tracing LV endocardial and epicardial borders on long axis images manually, the software algorithm tracked the contour from frame to frame throughout the cardiac cycle, and strain data were displayed. A 3D diastolic index (3D-DI) was calculated by % change of global strain during the first one-third of the diastolic period, and global 3D-DI of longitudinal (3D-DIlong), circumferential (3D-DIcirc), radial (3D-DIradial), and area strain (3D-DIas) were calculated by averaging them from 16 LV segments.9)
Thity seven patients were analyzable by 2D speckle tracking for 2D-SRelong and 36 patients for 2D-SRecirc and 2D-SReradial. 34 patients were found to be analyzable by 3D speckle tracking. The intraobserver variability was assessed in 5 randomly selected patients. The coefficients of variability were 8.2% for 2D-SRelong, 9.0% for 2D-SRecirc, 13.4% for 2D-SReradial, 11.3% for 3D-DIlong, 12.1% for 3D-DIecirc, 15.1% for 3D-DIradial, and 11.5% for 3D-DIas, respectively.
Hemodynamic data were collected using a pressure wire catheter. A 0.014" sensor-tipped pressure guide wire (PressureWire®, RADI medical system, Uppsala, Sweden) was introduced into the LV to measure LV end-diastolic pressure and end-systolic pressure and maximal and minimal rates of LV pressure change (dP/dtmax and dP/dtmin). LV pressure and dP/dt were recorded for at least one respiratory cycle, calculated electronically for every beat and averaged over the period of measurement, after excluding any poor recording values.
Continuous variables were expressed as mean±standard deviation and categorical variables were expressed as numbers and percentages. We categorized the patients in tertiles according to dP/dtmin level and applied a Wilcoxon rank sum test for trends across the ordered groups. Correlation analysis was used to compare echocardiographic measurements and invasively measured parameters. Receiver operating characteristic curves were constructed and confidence intervals for areas under the curves (AUC) were calculated to compare the predictive values of the ratios of E to various echocardiographic measurements. For all tests, a p<0.05 was considered to be significant.
Table 1 illustrates the baseline characteristics. The mean age of the 39 patients was 60±12 years (21 men). Among them, 14 patients were smokers, 11 patients had diabetes, 13 patients had hypertension, and 8 patients had dilated cardiomyopathy. Mean indexed LV end-diastolic volume was 85±39 mL/m2 and mean left ventricular ejection fraction (LVEF) was 46±16%. 19 patients had EF ≥50% and 13 patients had regional wall motion abnormalities.
Left ventricular end diastolic pressure (LVEDP) had a significant correlation with E/e' as well as E/2D-SRelong, E/2D-SRecirc, E/2D-SReradial, E/3D-DIlong, E/3D-DIcirc, and E/3D-DIas (Fig. 2). Fig. 3 showed the comparable predictive values of the ratios of E to 2D-SRelong, 2D-SReradial, 3D-DIlong, and 3D-DIas, which had acceptable correlations with the marker of LV relaxation, as compared with E/e'. Although E/3D-DIlong had the highest AUC among the variety of parameters, it did not offer any incremental value in addition to E/e', which is commonly used to estimate LV filling pressure, in terms of identifying patients with LVEDP ≥15 mm Hg (p=0.31). However, in the 21 patients who had E/e' of 8 to 15, E/3D-DIlong provided some incremental value for predicting those patients with increased LVEDP (p=0.04) (Fig. 4). E/2D-SRelong showed increased AUC to predict LVEDP in this population, despite not being statistically significant. Those patients with E/e' of 8 to 15 had more preserved LVEF than those with E/e' <8 or >15 (37.2±18.7% vs. 52.3±14.6%, p=0.007). 14 of 21 patients (67%) had LVEF ≥50% among patients with indeterminate E/e'.
Left ventricular diastolic dysfunction can be a sensitive marker for cardiovascular disease and provides prognostic information in a variety of patients.4)10-12) Determining LV filling pressure can prove cr-ucial in the diagnosis and treatment of patients with suspicious heart failure. Furthermore, abnormal LV diastolic filling pattern and elevated LV filling pressure predict worsened cardiovascular outcomes and the degree to which pressures can be reduced may predict survival.13)14) Over the past two decades, echocardiography has provided an important role in evaluating LV diastolic function. Traditionally, Doppler echocardiography, including tissue Doppler imaging, has become widely used to assess LV diastolic function and diastolic filling pressure. Mitral inflow pattern is helpful in identifying patients with abnormal LV relaxation and predicting diastolic filling pressure. E' is also commonly used to assess LV relaxation and filling pressure, since it is less dependent on preload. Prior studies have shown that E/e' is a useful parameter in estimating LV diastolic pressure non-invasively.15)16) However, E/e' is less sensitive to increased filling pressure, especially when EF is preserved. E' from septal annulus, lateral annulus, or both has a limitation in terms of reflecting LV global relaxation, especially among patients with regional wall motion abnormalities. In addition, it cannot specifically represent intrinsic LV diastolic properties.
Recently, new technological advances in echocardiography, speckle tracking echocardiography, enable a detailed assessment of LV systolic and diastolic function, including measuring myocardial deformation in radial, circumferential, and longitudinal directions. While these deformation measurements have advantages in angle-independency and relative load-independency as compared with conventional Doppler measurements, the utility of strain and strain rate in assessing LV diastolic function remains uncertain and subject to further investigation. Prior animal studies presented the relationship between longitudinal early diastolic strain rate and myocardial stiffness and LVEDP.17)18) Wang et al.19) has shown that the ratio of mitral early diastolic strain rate during the isovolumic relaxation period was predictive of LV filling pressure with reasonable accuracy, particularly for patients with normal EF and in those with regional abnormalities. More recently, 3D speckle tracking has developed further to overcome the intrinsic limitations of 2D speckle tracking. While 2D speckle tracking suffers the inherent limitations of 2D imaging, such as the use of foreshortened images and geometric remodeling, and has a problem in tracking out-of-motion adequately, 3D speckle tracking has a theoretical advantage in tracking the speckles in and out of the imaging planes.20-22) When considering heart movements in the 3D space, 3D speckle tracking may be a promising technique for evaluating myocardial mechanics in a more accurate and complete way. In our study, we evaluated the utility of the measurements by 2D and 3D speckle tracking for assessing diastolic function, and found that diastolic longitudinal strain and strain rate by 2D and 3D speckle tracking and the diastolic index of area strain were associated with the indices of LV relaxation and the ratios of mitral E to these parameters related to LVEDP. Furthermore, the ratio of mitral E to 3D-DIlong provided additional information in predicting elevated LVEDP among those patients with E/e' of 8 to 15, who were characterized as having more preserved LVEF. Deformation parameters may be beneficial in assessing diastolic function by differentiating active myocardial movement from passive translational or tethering motion and reflecting LV global relaxation, unlike tissue Doppler measurements. Although the ratio of E to 2D-SRelong also led to increased AUC in addition to E/e' in this population, it did not reach the level of statistical significance in our data. This may derive from the theoretical advantages of 3D speckle tracking in measuring more real deformation data from the 3D space through the acquisition of full volumetric dataset. However, the sample size was too small to draw conclusive statements in the present study. In addition, 3D speckle tracking has limitations in terms of its relatively low temporal and spatial resolution, although these limitations are expected to improve with technological advances in the near future. Low temporal resolution, in particular, would make the analysis of diastolic events more challenging.
Several limitations in our study should be noted. First, the study population was small, as previously mentioned. Second, we excluded patients with a poor echo window in the screening stage, and the testing may be difficult to apply to the general population. Additionally, we enrolled patients who had undergone cardiac catheterization for a variety of clinical indications, potentially leading to another selection bias. Third, we performed echocardiography within 24 hours of pressure measurement. Since patients usually fast before cardiac catheterization, preload may not be the same when echocardiography was performed. Finally, the frame rate may be somewhat low for the accurate assessment of strain rate in our study.
In summary, 2D-SRelong, 3D-DIlong, and 3D-DIas were related to LV relaxation with an acceptable correlation coefficient. The ratios of E to those parameters could reflect LVEDP, suggesting that they may provide additional information in evaluating LV diastolic function. In addition, E/3D-DIlong may be helpful in detecting elevated LVEDP, especially among a subset of patients with E/e' 8 to 15.
Figures and Tables
Fig. 1
Correlations between the echocardiographic parameters and the minimal rate of left ventricular filling.
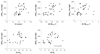
Fig. 2
Correlations between the ratio of E to various echocardiographic measures and left ventricular end diastolic pressure (LVEDP).
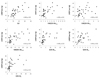
Fig. 3
Receiver operating characteristics curves of the ratio of E to various echo measures in identifying patients with left ventricular end diastolic pressure ≥15 mm Hg.
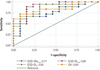
Fig. 4
Receiver operating characteristics curves of the ratio of E to various echo measures in identifying patients with left ventricular end diastolic pressure ≥15 mm Hg among those patients with E/Em 8 to 15.
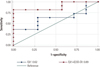
Acknowledgments
This study was supported by a grant (2010) from the Korean Society of Cardiology and an Inha University Research Grant (INHA40899-01).
References
1. Moller JE, Hillis GS, Oh JK, et al. Left atrial volume: a powerful predictor of survival after acute myocardial infarction. Circulation. 2003; 107:2207–2212.
2. Pritchett AM, Mahoney DW, Jacobsen SJ, Rodeheffer RJ, Karon BL, Redfield MM. Diastolic dysfunction and left atrial volume: a population-based study. J Am Coll Cardiol. 2005; 45:87–92.
3. Anavekar NS, Mirza A, Skali H, et al. Risk assessment in patients with depressed left ventricular function after myocardial infarction using the myocardial performance index--Survival and Ventricular Enlargement (SAVE) experience. J Am Soc Echocardiogr. 2006; 19:28–33.
4. Meta-Analysis Research Group in Echocardiography (MeRGE) AMI Collaborators. Møller JE, Whalley GA, et al. Independent prognostic importance of a restrictive left ventricular filling pattern after myocardial infarction: an individual patient meta-analysis: Meta-Analysis Research Group in Echocardiography acute myocardial infarction. Circulation. 2008; 117:2591–2598.
5. Sohn DW, Chai IH, Lee DJ, et al. Assessment of mitral annulus velocity by Doppler tissue imaging in the evaluation of left ventricular diastolic function. J Am Coll Cardiol. 1997; 30:474–480.
6. Nagueh SF, Appleton CP, Gillebert TC, et al. Recommendations for the evaluation of left ventricular diastolic function by echocardiography. J Am Soc Echocardiogr. 2009; 22:107–133.
7. Marwick TH. Measurement of strain and strain rate by echocardiography: ready for prime time? J Am Coll Cardiol. 2006; 47:1313–1327.
8. Oh JK, Park SJ, Nagueh SF. Established and novel clinical applications of diastolic function assessment by echocardiography. Circ Cardiovasc Imaging. 2011; 4:444–455.
9. Ishii K, Suyama T, Imai M, et al. Abnormal regional left ventricular systolic and diastolic function in patients with coronary artery disease undergoing percutaneous coronary intervention: clinical significance of post-ischemic diastolic stunning. J Am Coll Cardiol. 2009; 54:1589–1597.
10. Owan TE, Hodge DO, Herges RM, Jacobsen SJ, Roger VL, Redfield MM. Trends in prevalence and outcome of heart failure with preserved ejection fraction. N Engl J Med. 2006; 355:251–259.
11. Bhatia RS, Tu JV, Lee DS, et al. Outcome of heart failure with preserved ejection fraction in a population-based study. N Engl J Med. 2006; 355:260–269.
12. Penicka M, Bartunek J, Trakalova H, et al. Heart failure with preserved ejection fraction in outpatients with unexplained dyspnea: a pressure-volume loop analysis. J Am Coll Cardiol. 2010; 55:1701–1710.
13. Hillis GS, Møller JE, Pellikka PA, et al. Noninvasive estimation of left ventricular filling pressure by E/e' is a powerful predictor of survival after acute myocardial infarction. J Am Coll Cardiol. 2004; 43:360–367.
14. Stevenson LW, Tillisch JH, Hamilton M, et al. Importance of hemodynamic response to therapy in predicting survival with ejection fraction less than or equal to 20% secondary to ischemic or nonischemic dilated cardiomyopathy. Am J Cardiol. 1990; 66:1348–1354.
15. Ommen SR, Nishimura RA, Appleton CP, et al. Clinical utility of Doppler echocardiography and tissue Doppler imaging in the estimation of left ventricular filling pressures: a comparative simultaneous Doppler-catheterization study. Circulation. 2000; 102:1788–1794.
16. Nagueh SF, Middleton KJ, Kopelen HA, Zoghbi WA, Quiñones MA. Doppler tissue imaging: a noninvasive technique for evaluation of left ventricular relaxation and estimation of filling pressures. J Am Coll Cardiol. 1997; 30:1527–1533.
17. Park TH, Nagueh SF, Khoury DS, et al. Impact of myocardial structure and function postinfarction on diastolic strain measurements: implications for assessment of myocardial viability. Am J Physiol Heart Circ Physiol. 2006; 290:H724–H731.
18. Pislaru C, Bruce CJ, Anagnostopoulos PC, et al. Ultrasound strain imaging of altered myocardial stiffness: stunned versus infarcted reperfused myocardium. Circulation. 2004; 109:2905–2910.
19. Wang J, Khoury DS, Thohan V, Torre-Amione G, Nagueh SF. Global diastolic strain rate for the assessment of left ventricular relaxation and filling pressures. Circulation. 2007; 115:1376–1383.
20. Seo Y, Ishizu T, Enomoto Y, et al. Validation of 3-dimensional speckle tracking imaging to quantify regional myocardial deformation. Circ Cardiovasc Imaging. 2009; 2:451–459.
21. Mor-Avi V, Lang RM, Badano LP, et al. Current and evolving echocardiographic techniques for the quantitative evaluation of cardiac mechanics: ASE/EAE consensus statement on methodology and indications endorsed by the Japanese Society of Echocardiography. J Am Soc Echocardiogr. 2011; 24:277–313.
22. Urbano-Moral JA, Patel AR, Maron MS, Arias-Godinez JA, Pandian NG. Three-dimensional speckle-tracking echocardiography: methodological aspects and clinical potential. Echocardiography. 2012; 29:997–1010.
23. Urbano-Moral JA, Arias-Godinez JA, Ahmad R, et al. Evaluation of myocardial mechanics with three-dimensional speckle tracking echocardiography in heart transplant recipients: comparison with two-dimensional speckle tracking and relationship with clinical variables. Eur Heart J Cardiovasc Imaging. 2013; [Epub ahead of print].