Abstract
Animal models have long been developed for cardiovascular research. These animal models have been helpful in understanding disease, discovering potential therapeutics, and predicting efficacy. Despite many efforts, however, translational study has been underestimated. Recently, investigations have identified stem cell treatment as a potentially promising cell therapy for regenerative medicine, largely because of the stem cell's ability to differentiate into many functional cell types. Stem cells promise a new era of cell-based therapy for salvaging the heart. However, stem cells have the potential risk of tumor formation. These properties of stem cells are considered a major concern over the efficacy of cell therapy. The translational/preclinical study of stem cells is essential but only at the beginning stages. What types of heart disease are indicated for stem cell therapy, what type of stem cell, what type of animal model, how do we deliver stem cells, and how do we improve heart function? These may be the key issues that the settlement of which would facilitate the transition of stem cell research from bench to bedside. In this review article, we discuss state-of-the-art technology in stem cell therapies for cardiovascular diseases.
Stem cell therapies hold remarkable promise, particularly in the management of diseases and conditions for which there are currently limited or no treatment options.1) The ability of stem cells to self-renew and differentiate into all somatic cell types offers excellent prospects to regenerative medicine. Like all emerging technologies, however, creating new applications from an evolving knowledge base has been a slow process. In fact, there is a substantial gap between expectations and the reality of clinical stem cell application. Therefore, before clinical applications, a great deal of translational research is necessary to ensure that their therapeutic properties are both effective and safe.
Stem cell biology is highly complex and variable. This creates challenges for regulators developing the testing necessary to ensure the quality of new technologies and therapies. There are valid concerns about the consistency of cell cultures and the genetic stability of cell lines. As an example, both human embryonic stem cells (hESCs) and induced pluripotent stem cells (iPSCs) have been shown to demonstrate genetic and phenotypic drift in long-term in vitro culture. Given that there is the potential for late tumor formation resulting from accidental mutagenesis in the creation of stem cell products, long-term monitoring of animals used in preclinical studies will be required.
The teratoma forming property of stem cells is considered a major obstacle for biomedicine by the U.S. Food and Drug Administration (FDA). The potential for tumor formation represents a concern correlated with the self-renewal of undifferentiated cells, whereas cells at other levels of maturation may also pose a risk. Namely, many stem cell-based therapies will not consist of a pure, homogeneous target cell population, which raises additional questions about risks that non-target cells may present, as well as their physiological role after administration. Additionally, the differentiation of stem cell based products that are allogeneic with respect to the recipient results in increased immunologic incompatibility, due to the expression of foreign non-self antigens. In addition, the death of large proportions of the transplanted cell population, not unique to stem cells, may constitute further risk.2) To determine whether it is reasonable to grant permission for a clinical trial to proceed, the FDA evaluates potential risk based on results derived from the analytical assessment of product characteristics, as well as preclinical proof-of-concept and safety testing, which, collectively, are considered within the context of a proposed clinical study.3)
Small animals (mice, etc.) can provide information regarding the ability of the human cellular product to target the myocardium, as well as offering insight into potential safety issues related to the administered cells. These models can be used to study the potential for cellular products to survive and differentiate in the myocardium following infarction. However, they do not let themselves to use in any sensitive evaluation of overall cardiac function or unfavorable results related to the administration of cellular products, due to restrictions of anatomy and physiology related mainly to body size.
Large animals (swine, etc.) can provide information on the safety and activity of cellular products and delivery systems. As well, these models can be used to address issues such as cell numbers, volume, rate of injection, and the optimal location for product administration. These data cannot be generated using small animal models due to the inability to directly test catheters for clinical use and the limited ability to monitor cardiac function. The use of a sustainable and reproducible large animal model is a crucial step for various researchers to obtain FDA Investigational New Drug approval.
Over the past decade, major advances in stem cell biology have created great promise for regenerative medicine. In vivo transplanted stem cells can proliferate and differentiate into cardiomyocytes, endothelial cells, or smooth muscle cells (Fig. 1). This concept of stem cell transfer for enhancing cardiac repair has raised new therapeutic prospects. Although the underlying mechanisms remain poor, promising results have been reported in early preclinical studies. Despite ongoing controversies, clinical trials have shown a reassuring safety profile and suggest functional benefits. A number of clinical trials of stem cell therapy for cardiac repair have been registered with clinicaltrials.gov. These are listed in Table 1.
The majority of the studies have used intracoronary delivery following successful stenting of the infarct-related artery. Improvements in the left ventricular systolic function and the reduction in the size of scar tissue and cardiac volume have been referred to as the surrogate markers used to assess the efficacy of cell therapy.
Many studies showed improvements in ejection fraction, wall motion abnormalities, volume reduction, and reduced infarction size. In contrast, some trials did not demonstrate any benefits. The reasons for the inconsistent findings remain unclear, but the possibilities comprise differences in the cell numbers, timing, evaluation methods, and follow up periods. Past clinical trials are summarized in Table 2.4-9)
Initially, stem cell implantation was investigated in patients undergoing open-heart surgery. Mostly, the employment of direct intramyocardial injection methods has been used. Most recently, percutaneous transcatheter intramyocardial injection was introduced. Stem cell transplantation has been assessed in regional contralility, New York Heart Association functional class, quality of life, and evidence of reverse ventricular remodeling. Stem Cell Infusion in Patients with Ischemic CardiOmyopathy (SCIPIO) was the first trial using autologous cardiac progenitor cells in heart failure. In this first trial, results demonstrated an improvement in ejection fraction, heart failure score, and reduced infarction size.10) However, some trials did not produce any significant results.11)12)
Swine are superior large animal models for studies of acute myocardial infarction (MI) and ischemic cardiomyopathy (ICM), because the coronary artery anatomy, size, structure, and distribution make them suitable for preclinical cardiovascular research. As with the human anatomy, the porcine left anterior descending artery (LAD) provides approximately half of the blood supply to the left ventricle (LV), and its occlusion creates an MI that is similar in size and distribution to that occurring in humans as a result of LAD occlusion (Fig. 2). This is commonly used as a model of human cardiovascular disease.13-17) Although the left circumflex coronary artery occlusion technique has its benefits, such as lower procedural mortality, LAD occlusion is the preferred model for preclinical studies, considering that this is where the majority of human MI occurs.18) Moreover, the pattern of remodeling as a result of the MI closely resembles that occurring in humans.19) These similarities make the swine model highly appropriate for translational research, as the equipment and procedural techniques used to quantify these variables are analogous to those used in humans.
To establish a reproducible acute MI model, a percutaneous balloon catheter is used to occlude blood flow at the distal part of the first diagonal branch in the LAD. By restoring the blood flow of the first diagonal branch, some territory of the LV muscle can be saved. Accordingly, a portion of survived myocardium shows a border zone to the infracted myocardium.
Occlusion of the coronary artery can provide a predictable and reproducible MI model of anteroapical, lateral, and septal myocardium. The mechanism of the acute MI model results from reperfusion injury. After balloon occlusion, the myocardium is completely reperfused to mimic acute MI. According to protocols, by modifying occlusion time and location, an animal model could be provided.20)21)
To make a chronic model of ICM, stenosis or an occlusion model with significant depression of the LV are required. A chronic ICM model using a plastic occlude on the LAD has developed with low mortality rates and easy reproducibility. A plastic occluder of a fixed diameter and an 18-gauge copper wire were deployed around the proximal segment of the LAD coronary artery and fixed loosely with a cotton umbilical tape. A plastic occlude with a fixed diameter of 1.0 mm, fitted with an 18-gauge copper wire, was placed on the proximal LAD.15) Oral administration of dual anti-platelet agents were followed by the operation for 10 days.
Various stem cell populations exist, derived from both embryonic and adult human tissue sources. The most frequently studied stem cell populations in cardiac disease include embryonic stem cells, bone marrow-derived stem cells, tissue-specific stem cells, and, most recently, iPSCs. Each one has its own particular characteristics. According to current experiments, the findings are summarized in Table 3.22)
The optimal cell type for ischemic heart disease remains a controversial subject of debate. Previous studies have examined various cell types.23-25) Probably, different stem cell types will be considered in different heart disease types. The ideal cell type should meet the following standards: it must be safe, effective, able to regenerate healthy and functional cardiac muscle, easy to harvest, have no immune reaction, and it must not raise ethnical issues.
Currently, the major type of different stem cells used for basic and clinical studies in ischemic heart disease is mesenchymal stem cell, which have tremendous clinical potential due to their high expansion ratio and ability to be used for allogeneic transplantation.26)
A variety of methods have been used to deliver stem cells to the heart. These methods have included local intramyocardial injection, epicardial injection, intravenous injection, and intracoronary injection related to the infarct zone (Fig. 3). There are no definitive answers provided by these methods, but direct cell delivery into the injured heart is considered the most promising method of delivery. In large animal models, several studies have confirmed the safety and feasibility of direct injection methods.27-29)
Stem cells are injected into coronary circulation. In this way, we can deliver a higher concentration of SCs to the heart. This approach was first introduced in 2001 in a feasibility study of stem cell transplantation after primary percutaneous coronary intervention for acute MI. Since that trial, the majority of stem cell studies have been performed using this method.30)
This approach is less invasive, safe, and quite feasible. Because intracoronary infusion must use an opened occluded coronary artery, however, this technique is limited to the acute MI model. Besides, this approach poses the risk of coronary embolism and it is difficult to deliver to poor arterial supply. However, this selective intracoronary infusion approach remains the predominant stem cell delivery route. This intracoronary infusion has been verified as a feasible, safe technique and has demonstrated improvements in cardiac function as well as reductions in infarct size following intracoronary infusion.
By needle, stem cells are directly delivered into the myocardium. This epicardial procedure has been the most direct, precise, and accurate approach for injecting stem cells into an infracted region of the heart. The location can be identified preoperatively using imaging modalities, during surgery, by empirical observation.31) Direct intramyocardial injection can typically be conducted either during open heart surgery like coronary artery bypass graft32) or as a separate procedure performed without cardiac arrest via a lateral minithoracotomy.33) It offers the distinct advantage of targeting localized myocardium without perturbing any surrounding tissue and vasculature. In contrast to the intracoronary approach, myocardial injection enables stem cells to be delivered to an infracted area with a limited blood supply. Thereby, it circumvents the need to address complex issues such as mobilization of the transplanted cells. In addition, stem cells can be injected into the border zone of the infarction. Furthermore, there is no risk of coronary embolism. Therefore, an epicardial injection is performed in conjunction with open-heart surgery or left ventricular assistive device implantation,34)35) even if it was the most widely used method in preclinical research in small animals at first.
This direct injection can be carried out percutaneously and less invasively than an epicardial injection.36) Through the coronary vessel, a percutaneous catheter is entered into the left ventricular cavity. However, specialized catheters are needed for this method. With the introduction of new catheters and imaging modalities, physicians and scientists are now able to apply intramyocardial injections as a potential cellular therapy (Fig. 4). This method was first implemented and validated in a swine model by Fuchs et al.37) Since then, many clinical studies have been published with largely positive indications of its efficacy. No observations of any significant adverse complications have been made so far. However, this approach poses the potential risk of inducing fatal ventricular arrhythmia. To date, the equipment required is expensive and not widely available. With the continuous and rapid development in catheterization techniques and imaging devices, transendocardial injection appears to be a very viable, feasible, and safe approach for further investigation in the future, pending the results of current clinical trials.
Since those initial reports, numerous additional clinical stem cell trials have been conducted in the treatment of cardiac disease. Currently, iPSCs are not being conducted as clinical trials, but a hESCs trial commenced in October 2010 by Geron for spinal cord injuries. Unfortunately, this trial was halted in November 2011 after use in only four trial subjects. As of 2013, clinical trials for cardiovascular disease numbered 236 studies worldwide: 75% targeted the heart and 25% the peripheral vascular system. Almost all of these trials involved messenchymal stem cells, the mobilization of hematopoietic stem cells, other purified bone marrow-derived stem cells, or endothelial progenitor cells for regenerative and supportive purposes (Fig. 5).
In translational research, stem cell study is one of the most actively proceeding field. With the help of these preclinical research efforts, at present, new and exciting stem cell therapies are already being applied in clinical applications, as seen in the SCIPIO, Prospective Randomized Study of Mesenchymal Stem Cell Therapy in Patients Undergoing Cardiac Surgery (PROMETHEUS), the PercutaneOus StEm Cell Injection Delivery Effects on Neomyogenesis pilot study (POSEIDON), dilated cardiomyopathy (POSEIDON-DCM), and Transendocardial Autologous Cells in Ischemic Heart Failure Trial (TACHFT) trials.10)38-41)
The rapid progress of stem cell research has allowed for the almost simultaneous development of preclinical and clinical studies. However, questions still remain unanswered regarding the optimal type, dosing, timing, and delivery of stem cells. To establish convincing stem cell therapy, ongoing efforts in the exchange of knowledge from the bench to the bedside are required.
Figures and Tables
Fig. 1
Potential mechanisms through which cell therapy contributes to cardiovascular repair. Cell therapy may contribute to cardiac and vascular repair depending on the target population.42) EC: endothelial cell, SMC: smooth muscle cell, CSC: cardiac stem cell.
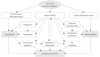
Fig. 2
Swine myocardial infarction model using balloon occlusion. A: swine placed dorsally recumbent under biplane angiography. Vascular access is shown through the right carotid artery and the right external jugular vein. B: left coronary angiogram shows the LAD (arrow 1), left circumflex coronary artery (arrow 2), and the first diagonal branch of the LAD (arrow 3). C: balloon obstructing all distal flows of the LAD past the first diagonal (arrow 4). D: representative electrocardiographic changes during myocardial infarction.43) LAD: left anterior descending artery.
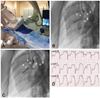
Fig. 3
Potential delivery routes for stem cells. Delivery options for implementing myocardial stem cell transfer. A: epicardial, B: endocardial, C: intracoronary, D: retroperfusion.
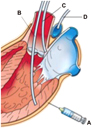
Fig. 4
Devices of transendocardial injection. A: the helical needle catheter is advanced through the Morph Universal Guide Catheter. Cells are injected through the helical tip. A second lumen at the base of the helix is used for contrast injection.39) B: injection catheter advanced into the left ventricle through the aortic valve. The catheter tip is placed against the endocardial surface (insert) with the needle extended into the myocardium, delivering stem cells. C: the NOGA Myostar Injection Catheter (Biosense Webster). D: NOGA 3D mapping.44)
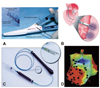
Fig. 5
Global status and trends of stem cell clinical trials. A: currently, 236 clinical trials have been conducting (data from clinicaltrials.gov). B: according to different types of stem cells, clinical trials have been conducted from 1991 to 2010.45)
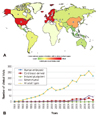
References
1. Weissman IL. Translating stem and progenitor cell biology to the clinic: barriers and opportunities. Science. 2000; 287:1442–1446.
2. Fink DW Jr. FDA regulation of stem cell-based products. Science. 2009; 324:1662–1663.
3. Moos M Jr. Stem-cell-derived products: an FDA update. Trends Pharmacol Sci. 2008; 29:591–593.
4. Britten MB, Abolmaali ND, Assmus B, et al. Infarct remodeling after intracoronary progenitor cell treatment in patients with acute myocardial infarction (TOPCARE-AMI): mechanistic insights from serial contrast-enhanced magnetic resonance imaging. Circulation. 2003; 108:2212–2218.
5. Schächinger V, Erbs S, Elsässer A, et al. Intracoronary bone marrow-derived progenitor cells in acute myocardial infarction. N Engl J Med. 2006; 355:1210–1221.
6. Huikuri HV, Kervinen K, Niemelä M, et al. Effects of intracoronary injection of mononuclear bone marrow cells on left ventricular function, arrhythmia risk profile, and restenosis after thrombolytic therapy of acute myocardial infarction. Eur Heart J. 2008; 29:2723–2732.
7. Lunde K, Solheim S, Aakhus S, et al. Intracoronary injection of mononuclear bone marrow cells in acute myocardial infarction. N Engl J Med. 2006; 355:1199–1209.
8. Tendera M, Wojakowski W, RuzyłłZo W, et al. Intracoronary infusion of bone marrow-derived selected CD34+CXCR4+ cells and non-selected mononuclear cells in patients with acute STEMI and reduced left ventricular ejection fraction: results of randomized, multicentre Myocardial Regeneration by Intracoronary Infusion of Selected Population of Stem Cells in Acute Myocardial Infarction (REGENT) Trial. Eur Heart J. 2009; 30:1313–1321.
9. Traverse JH, Henry TD, Ellis SG, et al. Effect of intracoronary delivery of autologous bone marrow mononuclear cells 2 to 3 weeks following acute myocardial infarction on left ventricular function: the LateTIME randomized trial. JAMA. 2011; 306:2110–2119.
10. Bolli R, Chugh AR, D'Amario D, et al. Cardiac stem cells in patients with ischaemic cardiomyopathy (SCIPIO): initial results of a randomised phase 1 trial. Lancet. 2011; 378:1847–1857.
11. Ang KL, Chin D, Leyva F, et al. Randomized, controlled trial of intramuscular or intracoronary injection of autologous bone marrow cells into scarred myocardium during CABG versus CABG alone. Nat Clin Pract Cardiovasc Med. 2008; 5:663–670.
12. Perin EC, Willerson JT, Pepine CJ, et al. Effect of transendocardial delivery of autologous bone marrow mononuclear cells on functional capacity, left ventricular function, and perfusion in chronic heart failure: the FOCUS-CCTRN trial. JAMA. 2012; 307:1717–1726.
13. Amado LC, Saliaris AP, Schuleri KH, et al. Cardiac repair with intramyocardial injection of allogeneic mesenchymal stem cells after myocardial infarction. Proc Natl Acad Sci U S A. 2005; 102:11474–11479.
14. Hatzistergos KE, Quevedo H, Oskouei BN, et al. Bone marrow mesenchymal stem cells stimulate cardiac stem cell proliferation and differentiation. Circ Res. 2010; 107:913–922.
15. Ishikawa K, Ladage D, Takewa Y, et al. Development of a preclinical model of ischemic cardiomyopathy in swine. Am J Physiol Heart Circ Physiol. 2011; 301:H530–H537.
16. Mewton N, Rapacchi S, Augeul L, et al. Determination of the myocardial area at risk with pre-versus post-reperfusion imaging techniques in the pig model. Basic Res Cardiol. 2011; 106:1247–1257.
17. Schuleri KH, Boyle AJ, Centola M, et al. The adult Göttingen minipig as a model for chronic heart failure after myocardial infarction: focus on cardiovascular imaging and regenerative therapies. Comp Med. 2008; 58:568–579.
18. Zhang J, Wilke N, Wang Y, et al. Functional and bioenergetic consequences of postinfarction left ventricular remodeling in a new porcine model. MRI and 31 P-MRS study. Circulation. 1996; 94:1089–1100.
19. Verdouw PD, van den Doel MA, de Zeeuw S, Duncker DJ. Animal models in the study of myocardial ischaemia and ischaemic syndromes. Cardiovasc Res. 1998; 39:121–135.
20. Pfeffer MA, Braunwald E. Ventricular remodeling after myocardial infarction. Experimental observations and clinical implications. Circulation. 1990; 81:1161–1172.
21. McCall FC, Telukuntla KS, Karantalis V, et al. Myocardial infarction and intramyocardial injection models in swine. Nat Protoc. 2012; 7:1479–1496.
22. Zhang H, Wang H, Li N, Duan CE, Yang YJ. Cardiac progenitor/stem cells on myocardial infarction or ischemic heart disease: what we have known from current research. Heart Fail Rev. 2013; [Epub ahead of print].
23. Mangi AA, Noiseux N, Kong D, et al. Mesenchymal stem cells modified with Akt prevent remodeling and restore performance of infarcted hearts. Nat Med. 2003; 9:1195–1201.
24. Sil AK, Maeda S, Sano Y, Roop DR, Karin M. IkappaB kinase-alpha acts in the epidermis to control skeletal and craniofacial morphogenesis. Nature. 2004; 428:660–664.
25. Kofidis T, Lebl DR, Martinez EC, Hoyt G, Tanaka M, Robbins RC. Novel injectable bioartificial tissue facilitates targeted, less invasive, large-scale tissue restoration on the beating heart after myocardial injury. Circulation. 2005; 112:9 Suppl. I173–I177.
26. Pittenger MF, Mackay AM, Beck SC, et al. Multilineage potential of adult human mesenchymal stem cells. Science. 1999; 284:143–147.
27. Amado LC, Saliaris AP, Schuleri KH, et al. Cardiac repair with intramyocardial injection of allogeneic mesenchymal stem cells after myocardial infarction. Proc Natl Acad Sci U S A. 2005; 102:11474–11479.
28. Hou D, Youssef EA, Brinton TJ, et al. Radiolabeled cell distribution after intramyocardial, intracoronary, and interstitial retrograde coronary venous delivery: implications for current clinical trials. Circulation. 2005; 112:9 Suppl. I150–I156.
29. Freyman T, Polin G, Osman H, et al. A quantitative, randomized study evaluating three methods of mesenchymal stem cell delivery following myocardial infarction. Eur Heart J. 2006; 27:1114–1122.
30. Strauer BE, Brehm M, Zeus T, et al. [Intracoronary, human autologous stem cell transplantation for myocardial regeneration following myocardial infarction]. Dtsch Med Wochenschr. 2001; 126:932–938.
31. Dib N, Menasche P, Bartunek JJ, et al. Recommendations for successful training on methods of delivery of biologics for cardiac regeneration: a report of the International Society for Cardiovascular Translational Research. JACC Cardiovasc Interv. 2010; 3:265–275.
32. Patel AN, Geffner L, Vina RF, et al. Surgical treatment for congestive heart failure with autologous adult stem cell transplantation: a prospective randomized study. J Thorac Cardiovasc Surg. 2005; 130:1631–1638.
33. Pompilio G, Steinhoff G, Liebold A, et al. Direct minimally invasive intramyocardial injection of bone marrow-derived AC133+ stem cells in patients with refractory ischemia: preliminary results. Thorac Cardiovasc Surg. 2008; 56:71–76.
34. Zhao Q, Sun Y, Xia L, Chen A, Wang Z. Randomized study of mononuclear bone marrow cell transplantation in patients with coronary surgery. Ann Thorac Surg. 2008; 86:1833–1840.
35. Dib N, Michler RE, Pagani FD, et al. Safety and feasibility of autologous myoblast transplantation in patients with ischemic cardiomyopathy: four-year follow-up. Circulation. 2005; 112:1748–1755.
36. Losordo DW, Schatz RA, White CJ, et al. Intramyocardial transplantation of autologous CD34+ stem cells for intractable angina: a phase I/IIa double-blind, randomized controlled trial. Circulation. 2007; 115:3165–3172.
37. Fuchs S, Baffour R, Zhou YF, et al. Transendocardial delivery of autologous bone marrow enhances collateral perfusion and regional function in pigs with chronic experimental myocardial ischemia. J Am Coll Cardiol. 2001; 37:1726–1732.
38. Williams AR, Hare JM. Mesenchymal stem cells: biology, pathophysiology, translational findings, and therapeutic implications for cardiac disease. Circ Res. 2011; 109:923–940.
39. Trachtenberg B, Velazquez DL, Williams AR, et al. Rationale and design of the Transendocardial Injection of Autologous Human Cells (bone marrow or mesenchymal) in Chronic Ischemic Left Ventricular Dysfunction and Heart Failure Secondary to Myocardial Infarction (TACHFT) trial: a randomized, double-blind, placebo-controlled study of safety and efficacy. Am Heart J. 2011; 161:487–493.
40. Quevedo HC, Hatzistergos KE, Oskouei BN, et al. Allogeneic mesenchymal stem cells restore cardiac function in chronic ischemic cardiomyopathy via trilineage differentiating capacity. Proc Natl Acad Sci U S A. 2009; 106:14022–14027.
41. Williams AR, Trachtenberg B, Velazquez DL, et al. Intramyocardial stem cell injection in patients with ischemic cardiomyopathy: functional recovery and reverse remodeling. Circ Res. 2011; 108:792–796.
42. Dimmeler S, Burchfield J, Zeiher AM. Cell-based therapy of myocardial infarction. Arterioscler Thromb Vasc Biol. 2008; 28:208–216.
43. McCall FC, Telukuntla KS, Karantalis V, et al. Myocardial infarction and intramyocardial injection models in swine. Nat Protoc. 2012; 7:1479–1496.
44. Perin EC, Dohmann HF, Borojevic R, et al. Transendocardial, autologous bone marrow cell transplantation for severe, chronic ischemic heart failure. Circulation. 2003; 107:2294–2302.
45. Bubela T, Li MD, Hafez M, Bieber M, Atkins H. Is belief larger than fact: expectations, optimism and reality for translational stem cell research. BMC Med. 2012; 10:133.