Abstract
Background and Objectives
It is unclear which plaque component is related with long-term clinical outcomes in patients with coronary artery occlusive disease (CAOD). We assessed the relationship between plaque compositions and long-term clinical outcomes in those patients.
Subjects and Methods
The study subjects consisted of 339 consecutive patients (mean 61.7±12.2 years old, 239 males) who underwent coronary angiogram and a virtual histology-intravascular ultrasound examination. Major adverse cardiac and cerebrovascular events (MACCE), including all-cause death, non-fatal myocardial infarction, cerebrovascular events, and target vessel revascularization were evaluated during a mean 28-month follow-up period.
Results
Patients with high fibrofatty volume (FFV, >8.90 mm3, n=169) had a higher incidence of MACCE (25.4% vs. 14.7%, p=0.015), male sex (75.7% vs. 65.3%, p=0.043), acute coronary syndrome (53.3% vs. 35.9%, p=0.002), multivessel disease (62.7% vs. 41.8%, p<0.001) and post-stent slow flow (10.7% vs. 2.4%, p=0.002) than those with low FFV (FFV≤8.90 mm3, n=170). Other plaque composition factors such as fibrous area/volume, dense calcified area/volume, and necrotic core area/volume did not show any impact on MACCE. Cardiogenic shock {hazard ratio (HR)=8.44; 95% confidence interval (CI)=3.00-23.79; p<0.001} and FFV (HR=1.85; 95% CI=1.12-3.07; p=0.016) were the independent predictors of MACCE by Cox regression analysis. Thin-cap fibroatheroma, necrotic core area, and necrotic core volume were not associated with MACCE.
Atherosclerotic plaque responsible for coronary heart disease is heterogeneous in their composition, containing a variable amount of lipid, scar tissue, calcium, neovessels, inflammatory cells, and thrombotic material.1) Various imaging techniques are currently under investigation for the tissue characterization of coronary plaque. Virtual histology-intravascular ultrasound (VH-IVUS) is one of the most promising techniques available. Indeed, VH-IVUS has been shown to have a 93-97% ex vivo and 87-92% in vivo accuracy rate for the characterization of four different types of atherosclerotic plaque (fibrous, fibrofatty, dense calcium, and necrotic core).2-8)
Until now, there have been few reports demonstrating an association between plaque composition and long-term clinical outcomes. Recently, there was a report that thin-cap fibroatheroma (TCFA), high plaque burden (PB), and small luminal area were responsible for long-term major adverse cardiac events (MACE) in non-culprit lesions with acute coronary syndrome (ACS).9) Other reports have shown only necrotic core and calcium were significantly greater in the non-culprit lesions of patients with a future MACE.10)
However, these reports evaluated the plaque composition of non-culprit lesions in ACS. Therefore, we sought to evaluate the impact of in vivo plaque composition of culprit lesions using VH-IVUS on long-term clinical outcomes in patients who had coronary artery occlusive disease (CAOD) and had undergone percutaneous coronary intervention (PCI).
All consecutive patients were prospectively enrolled in the Konyang University Hospital VH-IVUS registry (n=339). They had undergone successful PCI and VH-IVUS study between July 2006 and July 2008. They were followed up for a mean 28 months for major adverse cardiac and cerebrovascular events (MACCE). Exclusion criteria for VH-IVUS were severe vessel tortuousness or severe luminal narrowing with calcification precluding the insertion of an IVUS catheter. Stent selection and the use of a glycoprotein IIb/IIIa inhibitor were all up to the operator and physicians' discretion. Patient demographics and laboratory data were obtained before the PCI & VH-IVUS study. The study was approved by the hospital ethics committee of the Konyang University Hospital.
The VH-IVUS study, using a dedicated VH-IVUS console (Volcano Therapeutics, Rancho Cordova, CA, USA), was performed on native de novo target lesions (significance of the stenosis defined by an angiographic diameter stenosis >70%) in patients undergoing clinically indicated PCI after intracoronary administration of 100 to 200 µg nitroglycerin. A 20-MHz, 2.9 F monorail, electronic Eagle Eye Gold IVUS catheter (Volcano Therapeutics, Rancho Cordova, CA, USA) was advanced into the target lesion after wiring (n=242, 71.4%) or small sized (1.5 mm diameter) ballooning (n=97, 28.6%) in order to minimize the effect of ballooning on plaque morphology. Automatic pullback at 0.5 mm/s was conducted onto an aorto-ostial junction. The VH-IVUS image was recorded on a DVD-ROM for offline analysis at a later stage. The VH-IVUS uses spectral analysis of IVUS radio-frequency data to construct a tissue map.
Qualitative and quantitative analyses of gray scale IVUS images were performed according to the criteria of the American College of Cardiology's Clinical Expert Consensus Document on IVUS.3) Minimal luminal area (MLA) was identified as if there were several slices with equal lumen size, and that with the largest external elastic membrane and plaque cross sectional area was selected. A small MLA was defined as an area less than 4 mm2. Proximal and distal reference was defined by the site with the largest lumen proximal and distal to a stenosis, but usually within 10 mm of the stenosis with no major intervening branches, respectively.
These analyses were done on the culprit lesion with customized software (IVUS Lab; Volcano Therapeutics, Rancho Cordova, CA, USA) by examiners (Bae JH and Kwon TG) who were unaware of the clinical characteristics of the patients. For both the lumen and the media-adventitia interface, automatic border detection was performed at the predefined lesion segment. Then, the border detection was manually corrected again in the lesion after automatic border detection. After confirming the border detection, the software automatically calculates and shows the results. For each frame, virtual histological findings were expressed in colors, as previously described (green for fibrous, green-yellow for fibrofatty, white for dense calcified, and red for necrotic core area). In addition, the area (mm2) and percentage of each tissue component of plaque was expressed as well as conducting a volumetric analysis (mm3). The predictive accuracy of this method with tissue mapping has been validated.4)
Clinical follow-up data were obtained from outpatient records or telephone interviews.
A MACCE was defined as the composite of all-cause death, nonfatal myocardial infarction (MI), cerebrovascular events, or target vessel revascularization. Non-fatal MI was defined as the presence of clinical symptoms, electrocardiographic change, or abnormal imaging findings of MI, combined with an increase in creatine kinase-MB fraction or troponin T/I >99th percentile of the upper normal limit that was not related to an interventional procedure.11) Cerebrovascular events were defined as a stroke, transient ischemic attack, and reversible ischemic neurological deficit, as determined by a neurologist and confirmed on imaging. Target vessel revascularization was defined as the PCI of any segment of the epicardial coronary artery, including the target lesion. Low left ventricular ejection fraction (LVEF) was defined as LVEF less than 40%.
All analyses were performed with Statistical Package for the Social Sciences (SPSS, version 18.0; SPSS Inc., Chicago, IL, USA). Values are expressed as mean±SD. We used the Student t-tests, chi-square test, and Fisher's exact test to compare the mean or frequency of variables by the groups. Cumulative event rates were estimated using the Kaplan-Meier method. Relevant baseline clinical and VH-IVUS characteristics showing a significant difference (p<0.05) were included in the multivariate analysis. Multivariate logistic regression analysis was performed to assess the predictors of long-term MACCE. Cox regression analysis with a forward stepwise method was performed to evaluate the independent factors of long-term clinical outcomes in study patients.
Between July 2006 and July 2008, a total of 339 patients with CAOD were enrolled after they had undergone a successful PCI (Table 1). The median age was 63.0 years, 70.5% were men, 29.2% had diabetes mellitus, 52.2% had hypertension, 34.8% had dyslipidemia, and 36.9% were smokers. The mean follow-up was 28 months.
Each of the plaque compositions in MLA {fibrous area (FA), fibrofatty area (FFA), dense calcium area (DCA) and necrotic core area (NCA)} and the entire culprit lesion {fibrous volume (FV), fibrofatty volume (FFV), dense calcium volume (DCV), and necrotic core volume (NCV)} were divided into two groups according to median value to evaluate the impact of each plaque composition on long-term clinical outcomes. We also evaluated MACCE according to the median value of corrected plaque compositions, adjusted with different lesion length (volume/lesion length). The univariate logistic analysis showed that age (≥65 year old), cardiogenic shock, and FFV were significant predictors for MACCE (Table 2). TCFA, high PB, and small MLA were not significant predictors for MACCE. Another plaque composition, such as FA, FV, FFA, DCA, DCV, NCA, NCV, and each corrected plaque composition did not have any impact on MACCE. Multivariate logistic analysis was evaluated with reliable variables including patient age, cardiogenic shock, high FFV, ACS, low LVEF, post-stent no reflow, and multivessel disease. The independent predictors of long-term MACCE were cardiogenic shock, FFV, and patient age (Table 3). A Cox regression analysis also showed that cardiogenic shock {hazard ratio (HR)=8.44; 95% confidence interval (CI) I=3.00-23.79; p<0.001) and high FFV (HR=1.85; 95% CI=1.12-3.07; p=0.016} were the only significant predictors for MACCE (Table 4).
Therefore, the investigators divided the study subjects according to FFV to evaluate the impact of plaque compositions on long-term clinical outcomes.
Subgroup analysis was performed according to clinical diagnosis because ACS is one of the main risk factors of MACCE. Univariate analysis showed that previous MI, high PB (≥70%), and high FFV were significant predictors of MACCE in patients with stable angina, however, patient age, male sex, diabetes, smoking, small MLA, and larger NCA were significant predictors in patients with ACS. Multivariate logistic analysis showed that small MLA and larger NCA were significant predictors for MACCE only in patients with ACS.
High FFV (FFV>8.90 mm3, n=169) and low FFV (FFV≤8.90 mm3, n=170) were classified according to median value (8.90 mm3). The patients with high FFV had a higher incidence of male gender (75.7% vs. 65.3%, p=0.043), ACS (53.3% vs. 35.9%, p=0.002), and multivessel disease (62.7% vs. 41.8%, p<0.001) than patients with low FFV. The high FFV group had a higher rate of post-stent slow flow (10.7% vs. 2.4%, p=0.002), lower LVEF (60.8±11.3% vs. 64.7±10.2%, p=0.001), and longer stent length (24.4±5.5 mm vs. 21.4±5.0 mm, p<0.001) than the low FFV group. There were no significant differences in age, risk factors for coronary artery disease, lipid profile, stent type, and stent diameter (Table 5).
At the MLA, the external elastic membrane (EEM) area, lumen area, plaque and media (P&M) area, PB, FA and FFA were significantly larger and higher in the high FFV group than in the low FFV group. Remodeling index and positive remodeling were not significantly different between the two groups (Table 6).
For the entire lesion length (21.4±8.3 mm vs. 16.3±6.5 mm, p< 0.001), EEM volume (382.2±174.0 mm3 vs. 197.2±87.2 mm3, p< 0.001), lumen volume (141.7±69.5 mm3 vs. 87.0±38.1 mm3, p< 0.001), P&M volume (240.5±113.6 mm3 vs. 110.9±54.0 mm3, p<0.001), FV (97.6±52.7 mm3 vs. 34.4±19.9 mm3, p<0.001), FFV (28.2±20.6 mm3 vs. 4.1±2.4 mm3, p<0.001), DCV (11.8±10.9 mm3 vs. 7.6±8.1 mm3, p<0.001), and NCV (26.3±21.3 mm3 vs. 15.5±13.7 mm3, p<0.001) were significantly higher in the high FFV group than the low FFV group. The high FFV group also had significant corrected higher total plaque and media volume (114.8±35.7 mm3/cm vs. 69.8±24.8 mm3/cm, p<0.001), corrected FV (46.9±19.4 mm3/cm vs. 22.2±11.9 mm3/cm, p<0.001), corrected FFV (14.3±10.2 mm3/cm vs. 2.9±2.3 mm3/cm, p<0.001), and corrected NCV (12.2±8.7 mm3/cm vs. 9.5±7.5 mm3/cm, p=0.002) per fixed segment length, except corrected DCV.
A composite rate of MACCE was 25.4% in the high FFV group and 14.7% in the low FFV group (p=0.015), but all-cause death (11.2% vs. 5.3%, p=0.051), nonfatal MI (0.6% vs. 0.6%, p=1.000), cerebrovascular events (1.8% vs. 0.0%, p=0.123) and TVR (11.8% vs. 8.8%, p=0.378) were not significantly different between the two groups (Table 7). The Kaplan-Meier curve showed a significantly higher MACCE rate in the high FFV group (p=0.012) (Fig. 1).
To the best of our knowledge, this is the first study showing the impact of VH-IVUS findings at a culprit lesion on long-term clinical outcomes in patients who had CAOD and underwent PCI.
The main finding of this study is that high FFV in a culprit lesion was an important predictor of long-term clinical outcomes. Previous studies showed that the FFV of culprit lesions in patients who had undergone primary PCI was an important factor in long-term clinical outcomes.12) In the present study, subjects with stable angina as well as ACS were included and FFV was also an important predictor of long-term clinical outcomes, but not other plaque compositions including NCV and DCV. Corrected high FFV adjusted by lesion length also had a similar tendency to increase the incidence of MACCE, although it was not statistically significant (p=0.055).
One study showed that NCA and DCA were greater in the non-culprit lesions of patients who had diabetes and metabolic syndrome with MACE than without MACE.10) However, this study showed no significant differences in plaque compositional area, including FA, FFA, DCA, and NCA for long-term MACCE. The reason could possible be because the study included only ACS patients, and the result was from non-culprit lesions.
The PROSPECT study showed TCFA, high PB, and small MLA were related with high MACE in non-culprit lesions in ACS.9) Our study showed that TCFA was correlated with ACS, but was not related with MACCE. In addition, high PB and small MLA did not affect MACCE. The high FFV group had larger MLA and had a worse MACCE rate than the low FFV group. This difference could result from the problems of culprit or non-culprit lesions and whether they included stable angina or not.
Previous studies have demonstrated that plaque composition has several clinical significances, such as positive remodeling, plaque vulnerability, slow flow phenomenon, risk factor for sudden coronary death and cardiovascular risk factors.13-17) Several studies have revealed that positive remodeling lesions have a large lipid burden, 7)18)19) but also less necrotic core percent area at the MLA site, compared with intermediate/negative remodeling lesions.15) It has been shown that a large amount of calcium deposition is related to negative remodeling,19)20) whereas other studies identified calcium deposition as an indicator of positive remodeling.18)21) The association between coronary artery remodeling and plaque composition remains controversial. In this study, coronary artery remodeling was not found to be correlated with MACCE.
Plaque composition is associated with plaque disruption and thrombosis, which leads to acute coronary events.22-25) Lesions with a large lipid core have a higher risk for disruption than sclerotic plaques.25-27) However, there were differences in clinical outcomes according to the coronary plaque composition. Bae et al.13) reported that patients with slow flow were associated with more FV and FFV, but not NCV, at the time of the primary PCI for acute MI. Nakamura et al.14) also reported a trend towards a larger percentage of FFV in the no-reflow group than in the normal-reflow group in a VH-IVUS study. Our study also showed that there was a significant correlation between high FFV and post-stent slow flow. To clarify this result, further investigation is required.
Our study has some limitations. We arbitrarily divided the study population into two groups according to plaque composition, because the present study focused only on the clinical outcomes according to coronary plaque composition. Furthermore, until now, there is no cut-off value or appropriate value for the absolute plaque composition. There may be the possibility of selection bias. However, multivariate analysis showed that FFV was a significant independent predictor of MACCE. Subgroup analysis depending on clinical manifestations such as ACS showed that FFV was not a significant predictor of MACCE. These conflicts should be resolved in future studies. Second, the current VH-IVUS tree is not able to differentiate intraluminal thrombus from other plaque components.28) Thrombus may be misclassified as fibrous plaque (fibrofatty dependent on age), proportionally increasing this plaque component at the expense of others.29) Therefore, we analyzed the lesions, excluding the plaque cavity, in order to avoid any possible thrombus, potentially leading to an underestimation of NCA. Finally, we did not analyze the impact of lesion type of the culprit lesion on MACCE, because there are still some ambiguous issues with the lesion type, such as lumen containing necrotic core angle, which is a main important characteristic of TCFA. Similarly, the amount of calcium can be misinterpreted due to some artifact usually expressed as a quadrangle.
In conclusion, the present study suggests that VH-IVUS analysis for plaque composition in a culprit lesion may be useful to predict the risk of long-term clinical outcomes in patients who have undergone PCI.
Figures and Tables
Fig. 1
Comparison of Kaplan-Meier curves for major adverse cardiac and cerebrovascular events (MACCE) according to fibrofatty volume (FFV). A log-rank showed a significant difference (p=0.012) between the low FFV (FFV≤8.90 mm3) and high FFV groups (FFV>8.90 mm3).
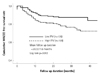
Table 2
Univariate analysis for MACCE
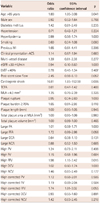
MACCE: major adverse cardiac and cerebrovascular events, MI: myocardial infarction, ACS: acute coronary syndrome, eGFR: estimated glomerular filtration rate, LVEF: left ventricle ejection fraction, TCFA: thin-cap fibroatheroma, MLA: minimal lumen area, FA: fibrous area, FFA: fibrofatty area, DCA: dense calcium area, NCA: necrotic core area, PV: plaque volume, FV: fibrous volume, FFV: fibrofatty volume, DCV: dense calcium volume, NCV: necrotic core volume
References
1. Shah PK. Mechanisms of plaque vulnerability and rupture. J Am Coll Cardiol. 2003; 41:4 Suppl S. 15S–22S.
2. DeMaria AN, Narula J, Mahmud E, Tsimikas S. Imaging vulnerable plaque by ultrasound. J Am Coll Cardiol. 2006; 47:8 Suppl. C32–C39.
3. Fujii K, Carlier SG, Mintz GS, et al. Association of plaque characterization by intravascular ultrasound virtual histology and arterial remodeling. Am J Cardiol. 2005; 96:1476–1483.
4. Nair A, Kuban BD, Tuzcu EM, Schoenhagen P, Nissen SE, Vince DG. Coronary plaque classification with intravascular ultrasound radiofrequency data analysis. Circulation. 2002; 106:2200–2206.
5. Nasu K, Tsuchikane E, Katoh O, et al. Accuracy of in vivo coronary plaque morphology assessment: a validation study of in vivo virtual histology compared with in vitro histopathology. J Am Coll Cardiol. 2006; 47:2405–2412.
6. Rodriguez-Granillo GA, McFadden EP, Valgimigli M, et al. Coronary plaque composition of nonculprit lesions, assessed by in vivo intracoronary ultrasound radio frequency data analysis, is related to clinical presentation. Am Heart J. 2006; 151:1020–1024.
7. Rodriguez-Granillo GA, Serruys PW, Garcia-Garcia HM, et al. Coronary artery remodelling is related to plaque composition. Heart. 2006; 92:388–391.
8. Nair A, Margolis MP, Kuban BD, Vince DG. Automated coronary plaque characterisation with intravascular ultrasound backscatter: ex vivo validation. EuroIntervention. 2007; 3:113–120.
9. Stone GW, Maehara A, Lansky AJ, et al. A prospective natural-history study of coronary atherosclerosis. N Engl J Med. 2011; 364:226–235.
10. Marso SP, Mercado N, Maehara A, et al. Plaque composition and clinical outcomes in acute coronary syndrome patients with metabolic syndrome or diabetes. JACC Cardiovasc Imaging. 2012; 5:3 Suppl. S42–S52.
11. Thygesen K, Alpert JS, Jaffe AS, et al. Third universal definition of myocardial infarction. Circulation. 2012; 126:2020–2035.
12. Kim WH, Park HW, Kim KH, et al. Fibro-fatty component is important for the long-term clinical events in patients who have undergone primary percutaneous coronary intervention. Korean Circ J. 2012; 42:33–39.
13. Bae JH, Kwon TG, Hyun DW, Rihal CS, Lerman A. Predictors of slow flow during primary percutaneous coronary intervention: an intravascular ultrasound-virtual histology study. Heart. 2008; 94:1559–1564.
14. Nakamura T, Kubo N, Ako J, Momomura S. Angiographic no-reflow phenomenon and plaque characteristics by virtual histology intravascular ultrasound in patients with acute myocardial infarction. J Interv Cardiol. 2007; 20:335–339.
15. Surmely JF, Nasu K, Fujita H, et al. Association of coronary plaque composition and arterial remodelling: a virtual histology analysis by intravascular ultrasound. Heart. 2007; 93:928–932.
16. Missel E, Mintz GS, Carlier SG, et al. In vivo virtual histology intravascular ultrasound correlates of risk factors for sudden coronary death in men: results from the prospective, multi-centre virtual histology intravascular ultrasound registry. Eur Heart J. 2008; 29:2141–2147.
17. Philipp S, Böse D, Wijns W, et al. Do systemic risk factors impact invasive findings from virtual histology? Insights from the international virtual histology registry. Eur Heart J. 2010; 31:196–202.
18. Burke AP, Kolodgie FD, Farb A, Weber D, Virmani R. Morphological predictors of arterial remodeling in coronary atherosclerosis. Circulation. 2002; 105:297–303.
19. Fuessl RT, Kranenberg E, Kiausch U, Baer FM, Sechtem U, Höpp HW. Vascular remodeling in atherosclerotic coronary arteries is affected by plaque composition. Coron Artery Dis. 2001; 12:91–97.
20. Sabaté M, Kay IP, de Feyter PJ, et al. Remodeling of atherosclerotic coronary arteries varies in relation to location and composition of plaque. Am J Cardiol. 1999; 84:135–140.
21. Ehara S, Kobayashi Y, Yoshiyama M, et al. Spotty calcification typifies the culprit plaque in patients with acute myocardial infarction: an intravascular ultrasound study. Circulation. 2004; 110:3424–3429.
22. Virmani R, Kolodgie FD, Burke AP, Farb A, Schwartz SM. Lessons from sudden coronary death: a comprehensive morphological classification scheme for atherosclerotic lesions. Arterioscler Thromb Vasc Biol. 2000; 20:1262–1275.
23. Falk E, Shah PK, Fuster V. Coronary plaque disruption. Circulation. 1995; 92:657–671.
24. Silva JA, Escobar A, Collins TJ, Ramee SR, White CJ. Unstable angina. A comparison of angioscopic findings between diabetic and nondiabetic patients. Circulation. 1995; 92:1731–1736.
25. Libby P. Molecular bases of the acute coronary syndromes. Circulation. 1995; 91:2844–2850.
26. Davies MJ, Richardson PD, Woolf N, Katz DR, Mann J. Risk of thrombosis in human atherosclerotic plaques: role of extracellular lipid, macrophage, and smooth muscle cell content. Br Heart J. 1993; 69:377–381.
27. Buja LM, Willerson JT. Role of inflammation in coronary plaque disruption. Circulation. 1994; 89:503–505.
28. Carlier SG, Mintz GS, Stone GW. Imaging of atherosclerotic plaque using radiofrequency ultrasound signal processing. J Nucl Cardiol. 2006; 13:831–840.
29. Frutkin AD, Mehta SK, McCrary JR, Marso SP. Limitations to the use of virtual histology-intravascular ultrasound to detect vulnerable plaque. Eur Heart J. 2007; 28:1783–1784.