Abstract
Background and Objectives
Existing data on the spatiotemporal expression patterns of a variety of galectins in murine atherosclerosis are limited. We investigated the expression levels of galectins, and their in vivo spatiotemporal expression patterns and statin responsiveness in the inflamed atherosclerotic plaques of apolipoprotein E (apoE)-/- mice.
Materials and Methods
Galectins expression patterns in aortic atherosclerotic plaques and serum galectin-3 levels were investigated in 26-week-old apoE-/- (n=6) and C57BL/6 mice (n=9). To investigate the spatial and temporal patterns of galectin-1 and galectin-3 in plaques, high-cholesterol diet-fed 26-week-old (n=12) and 36-week-old apoE-/- mice (n=6) were sacrificed and their aortas were examined for galectins' expression using immunoblot analysis and immunohistochemical stain. 36-week-old apoE-/- mice were treated with atorvastatin (n=3, 0.57 mg/kg/day) for the evaluation of its effect on aortic galectins' expression.
Results
Immunoblot analyses showed that galectin-1 and galectin-3 were the predominant galectins expressed in murine atherosclerosis. The serum galectin-3 level was significantly higher in apoE-/- mice (p<0.001). While galectin-1 was weakly expressed in both intimal plaques and the media of atherosclerotic aortas, galectin-3 was heavily and exclusively accumulated in intimal plaques. Galectin-3 distribution was colocalized with plaque macrophages' distribution (r=0.66). As the degree of plaque extent and inflammation increased, the intraplaque galectin-3 expression levels proportionally elevated (p<0.01 vs. baseline), whereas galectin-1 expression had not elevated (p=0.14 vs. baseline). Atorvastatin treatment markedly reduced intraplaque galectin-3 and macrophage signals (p<0.001 vs. baseline), whereas it failed to reduce galectin-1 expression in the aortas.
Inflammation is involved in all stages of atherosclerosis including foam cell formation, the proliferation and migration of vascular smooth muscle cell (VSMC), and ultimately plaque disruption and thrombus formation.1) As cell-to-cell interactions are critical in the processes of most diseases, galectins (gals) have become of interest as novel regulators of inflammation.2)3) Gals are a family of β-galactoside-binding lectins that recognize specific oligosaccharide, ligand glycoproteins or glycolipids on the membranes of neighboring cells or in the extracellular matrix.4) They are involved in intercellular signaling, cell-to-cell, and cell-to-matrix adhesion, apoptosis, angiogenesis, and innate and adaptive immunity, which may contribute to the pathogenesis of atherosclerosis.5)
However, data on whether gals themselves are upregulated, what kinds of gals are highly expressed, and which gals increase in proportion to the progression of atherosclerosis in inflammatory atherosclerosis, are limited. Of the 14 to 15 gals found in mammals, gal-1 and gal-3 have been widely studied for their association with atherosclerosis. While galectin-1 is upregulated in both VSMCs and monocytes,6) gal-3 is mainly secreted from activated macrophages,7)8) and highly expressed in macrophages of inflammatory atherosclerotic plaques,9) suggesting different spatial and temporal distributions of gal-1 and gal-3 in vessel walls. Furthermore, gal-3 has been postulated as a new possible biomarker for acute coronary syndrome.10) Patients with unstable angina have higher plasma gal-3 levels than stable patients. Based on these findings, we hypothesized that gal-3 might be an indicator for both atherosclerotic plaque progression and its regression in response to statin treatment.
To address these possibilities, we first investigated the expression levels of a variety of gals in atherosclerotic plaques of apolipoprotein E (apoE)-/-, a mouse model of murine atherosclerosis, and compared with those in C57BL/6 mice. We then investigated further the in vivo spatial and temporal expression pattern of gal-1 and gal-3 in atherosclerotic plaques of apoE-/- mice and finally determined whether gal-1 and/or gal-3 could function as a marker of statin responsiveness, i.e., plaque regression. These results could reveal that gals may be used as an indicator for the progression or regression of inflamed atherosclerotic plaques.
Gals expression patterns in aortic atherosclerotic plaques were investigated in apoE-/- mice (n=18 male mice; Jackson Laboratories, Bar Harbor, ME, USA) and C57BL/6 mice (n=9). ApoE-/- mice consumed a high-cholesterol diet (HCD; D12079B; 41% fat, 0.21% cholesterol; New Brunswick, NJ, USA) from the age of 10 weeks. They were housed in colony cages in a temperature-controlled environment (20-26℃) with a 12 hour light/dark cycle. They were allowed water and their specified diet ad libitum. To compare the gals' expression patterns as the plaque inflammation worsened, the mice were sacrificed at the age of 26 weeks and 36 weeks, their bloods were taken to obtain serum samples, and their aortas were extracted for histology and molecular works. Another group of 36 week-old HCD-fed apoE-/- mice (n=6) were randomized to atorvastatin (n=3; 0.57 mg/kg/day using a gavage tube)11) or saline of the same amount for 5 days. Histopathological analyses were then carried out on the mice after euthanasia. All animal experiments were conducted according to the Guideline for the Care and Use of Laboratory Animals published by the US National Institute of Health (NIH Publication No. 85-23, revised 1996), and approved by the Animal Committee.
After sacrifice, the mice were perfused with 0.9% saline (20 mL) via the left ventricle. For histopathological analysis, excised aortic roots were embedded in optimal cutting temperature compound (Sakura Finetek, Torrance, CA, USA) and the remainder of the aorta was snap-frozen for western blot analysis. Serial 5-µm thick cryostat sections were obtained from embedded aortic roots and were stained with hematoxylin and eosin for general morphology. Immunohistochemistry on adjacent sections was performed for macrophages (anti-macrophage/monocytes antibody, Merck Millipore, Darmstadt, Germany), gal-1 (anti-mouse gal-1, Abcam Inc., Cambridge, MA, USA) and gal-3 (anti-mouse gal-3, Abcam Inc.) using HRP-conjugated secondary antibody (Histomouse™-MAX kit, Invitrogen, Carlsbad, CA, USA). Sections treated with 3% hydrogen peroxide were incubated overnight (4℃) with a primary antibody, followed by HRP-conjugated secondary antibody. The reaction was visualized with DAB chromogen/substrate, and counterstained with Harris hematoxylin solution. We then performed the immunofluorescence staining for macrophages detection (anti-macrophage/monocytes antibody, Merck Millipore, Darmstadt, Germany) and gal-3 (anti-mouse gal-3, Abcam Inc.) using anit-rabbit Alexa 488 for green fluorescent signal and anti-rat Alexa 555 for red fluorescent signal (Molecular probe, Eugene, OR, USA) for multicolor immunofluorescence using an upright fluorescence microscope (Axio Imager M1 Microscope, Carl Zeiss, Germany).
Stained tissue sections were viewed using an uplight microscope (LEICA CTR 5000, LEICA, Wetzlar, Germany). Measurements were performed with TOMORO SCOPE EYE™ 3.5 software (version 3.5.208). The percentage macrophage, percentage gal-1 and percentage gal-3 staining areas were defined as the respective positive immunostained areas divided by the total aortic plaque area.
For immunoblotting of aortic lysates, the frozen aortas were first pulverized using a gentle MACS Dissociator. They were then homogenized by adding a RIPA buffer (50 mM Tris, 150 mM NaCl, 1% Nonidet P-40, 0.5% sodium deoxycholate, 0.1% sodium dodecyl sulfate) with freshly added protease inhibitors and centrifuged (20 minutes, 12000 rpm, 4℃). Next, the protein extract was boiled in an electrophoresis buffer for 10 minutes, followed by the separation of on 10% polyacrylamide gels under reducing conditions (β-mercaptoethanol) and the was then transferred onto PVDF membranes using a semi-dry transfer method (15 minutes, 30 V). After nonspecific binding sites were blocked for 1 hour with 5% delipidated milk in TBST (20 mM Tris, 55 mM NaCl, and 1% Tween 20), immunoblotting for gal-1 (Abcam Inc., ab108389, Rabbit monoclonal), gal-2 (Abcam Inc., ab93336, Rabbit polyclonal), gal-3 (Abcam Inc., ab53082, Rabbit polyclonal), and gal-8 (Abcam Inc., ab109519, Rabbit monoclonal) was performed using chemiluminescence (Young In Frontier, Seoul, Korea).
At the time of sacrifice, blood was obtained for the measurement of serum gal-3 level using a commercial enzyme-linked immuno sorbent assay (ELISA) Kit (RayBiotech Inc., Norcross, GA, USA) in both HCD-fed 26 week-old apoE-/- mice and chow diet-fed C57BL/6 mice of the same age.
All statistical analyses were performed using Statistical Package for the Social Sciences (SPSS) version 19 (SPSS, Inc., an IBM Company, Chicago, IL, USA). Data were expressed as mean±SD. The unpaired Student's t-test was used to test the difference between the two groups in the percentage of gal-1, gal-3, macrophage expression levels in histology, gal protein levels on immunoblotting, and serum levels of gal-3. Pearson correlation coefficients (r-values) were calculated for correlations between the percentage macrophage area and the percentage gal-3 area in atherosclerotic plaques. Null hypotheses of no difference were rejected if p were less than 0.05.
To determine the expression of various gals during atherogenesis, apoE-/- aortas with plaques were immunoblotted for gal-1, gal-2, gal-3 and gal-8. While gal-2 and gal-8 proteins were not detected in both HCD-fed aged apoE-/- mice with heavy plaque burdens and C57BL/6 mice with healthy vessels, gal-1 and gal-3 proteins were evidently expressed only in apoE-/- mice and not in C57BL/6 mice (Fig. 1A and B). Quantitative densitometry showed gal-3 proteins were more predominantly expressed than gal-1 in atherosclerotic plaques of apoE-/- mice compared with vessels of C57BL/6 mice: (data expressed as mean±SD) gal-1, 0.35±0.03 vs. 0.1±0.01, arbitrary units, p<0.001; gal-3, 1.19±0.34 vs. 0.06±0.01, arbitrary units, p<0.01. In addition, to compare the secreted gal-3 levels in serum between cholesterol-fed apoE-/- mice and the control, we examined serum gal-3 levels using ELISA. Cholesterol-fed 26-week-old apoE-/- mice showed markedly elevated serum gal-3 levels without any exceptions compared with C57BL/6 mice: 209.5±30.5 vs. 35.6±7.7 ng/mL, p<0.001) (Fig. 1C).
Immunostaining for gal-1 and gal-3 was carried out in the aortic roots of cholesterol-fed 26-week-old apoE-/- mice to assess their distribution throughout the vessels. Gal-3 was more abundantly expressed in intimal lesions than gal-1, similar to the immunoblotting results (Fig. 2C and D). The distribution of gal-3 expression was colocalized with the pattern of macrophage staining (Fig. 2B and D). Interestingly, the expression of gal-3 protein was confined to the intimal plaque area, but not in the media (Fig. 2D). However, gal-1 protein was present in the media as well as in the intimal lesion, though it was weakly expressed.
To assess the changes in the expression of gal-1 and gal-3 as plaque inflammation worsened, immunostaining was performed in the aortas of HCD-fed 26-week-old and 36-week-old apoE-/- mice and compared (Fig. 3A and B). The plaques of aged mice with more macrophage content demonstrated significantly increased gal-3 proteins over the plaques of younger mice with less macrophage content (Fig. 3B): percentage gal-3 staining area for 26-week-old versus 36-week-old apoE-/- mice, 69.8±4.7% vs. 82.1±2.6% (18% increase) (p<0.01). In contrast, gal-1 expression did not show an increasing trend as plaque inflammation became severe: percentage gal-1 staining area for 26-week-old versus 36-week-old apoE-/- mice, 65.1±4.8% vs. 70.7±5.5% (p=0.14) (Fig. 3C). Generally, the in vivo gal-1 and gal-3 expression patterns during plaque growth differed significantly.
To better understand the relationship between plaque gal-3 expression and plaque macrophage contents in vivo, Pearson correlation coefficients between the percentage of gal-3 and the percentage of the macrophage staining area were calculated from all included apoE-/- mice (Fig. 4). In the entire cohort, a strong relationship existed between the percentage of gal-3 and the macrophage staining area (r=0.66, p=0.007).
To determine whether statin represses gal protein expression in vivo, atorvastatin was administered to HCD-fed 36-week-old apoE-/- mice for 5 days and compared with their saline-treated counterparts (Fig. 5A and B). Statin treatment markedly reduced gal-3 protein expression as well as macrophage content even with a short course of therapy: percentage gal-3 staining area for saline-treated vs. atorvastatin-treated apoE-/-mice, 82.1±2.6% vs. 40.9±13.1% (p<0.001) (50% reduction) (Fig. 5C); percentage macrophage staining area for saline-treated vs. atorvastatin-treated apoE-/- mice, 68.3±12.7% vs. 53.8±6.8% (21% reduction) (p=0.054). Meanwhile, gal-1 protein expression was not significantly altered with statin therapy (salinetreated apoE-/- mice, 70.7±5.5% vs. atorvastatin-treated apoE-/- mice, 73.4±3.6%, p=0.67). Overall findings were consistent in Westen blot analyses of saline-treated vs statin-treated apoE-/- mice aortas (Fig. 5D and E).
Gals are animal lectins defined by shared consensus amino acid sequences and have an affinity for beta-galactose-containing oligosaccharides. Gals have recently been shown to function as novel regulators of inflammation.2)3) They are involved in cell adhesion, apoptosis, and chemotaxis and can also function as scavenger receptors.5) In addition, a growing body of experimental evidence indicates that gals may play a key role in the initiation, amplification or resolution of chronic inflammatory processes such as atherosclerosis.12) However, the role of gals in inflammation and atherogenesis is still contradictory. While some studies have suggested that gal-3 negatively regulates T cell activation13) and drives alternative macrophage activation,14) others have shown that gal-3 exerts cytokine-like regulatory actions through the JAK-STAT pathway,15) and aggravates joint inflammation in antigen-induced arthritis.16) In atherogenesis, whereas gal-3 has been reported to propagate vascular inflammation by inducing the expression of proinflammatory mediators in macrophages and the migration of monocytes into vascular walls,8) in another study9) gal-3 ablation has been shown to increase plaque infiltration of Th1 lymphocytes and monocyte-macrophage infiltration leading to accelerated atherogenesis.
Before elucidating the pathogenetic role of gals in atherogenesis, it should be noted that existing data on whether gals themselves are upregulated, what kinds of gals are highly expressed, and which gals increase in proportion to the progression of atherosclerosis in inflammatory atherosclerosis, is limited. We confirmed that gals were exclusively expressed in the plaques of atheroprone mice but not in the healthy vessels of control mice using aorta-proteomics. We also verified that, among gals associated with the pathogenesis of inflammation such as gal-1, gal-2, gal-3, and gal-8,17-25) gal-1 and gal-3 were predominant in atherosclerotic plaques as vessel wall inflammation worsened. Additionally, we found that while gal-3 predominantly accumulated in the atherosclerotic plaques as the plaque macrophage burden increased, it is sparsely distributed in the media comprising VSMCs. Thus, as vessel wall inflammation worsened, the extent of gal-3 proportionally increased. Meanwhile, gal-1 was expressed both in the intimal plaques and media, though weakly expressed compared with gal-3. Interestingly, in contrast with gal-3, gal-1 did not show an incremental expression pattern in proportion to the plaque macrophage content.
When we analyzed and compared the spatial and temporal expression pattern of gal-1 and gal-3, we found that gal-3 is exclusively present in macrophage-rich plaque areas but is scarce in VSMC-rich media, and that the gal-3 contents were proportionally increased with inflammatory plaque burdens. However, gal-1 expression did not change to a greater extent as the plaque area increased, and gal-1 was diffusely expressed through the vessel walls, including the intimal lesion and media. Whereas gal-1 is found on the cell surfaces of endothelial or smooth muscle cells in human artery and seems to affect adhesion, migration, and proliferation of VSMCs,26) gal-3 was found to be expressed in foam cells or macrophages of atherosclerotic plaques. Gal-3 deficiency significantly reduced the progression of atherosclerosis in apoE-/- mice, which was characteristically associated with lower numbers of inflammatory infiltrates.17)20) Gal-3 is highly upregulated when monocytes differentiate into macrophages,27) and may be secreted from plaque foam cells or activated macrophages.7)8) In addition, gal-3 is a potent chemoattractant for monocytes and thus further enhances inflammatory cells into vessel walls.8) These features of gal-1 and gal-3 may explain for their in vivo distribution pattern in murine atherosclerosis, as shown in this study.
Statins can effectively reduce the macrophage contents in human carotid atherosclerotic plaques by modulating low-density lipoprotein cholesterol levels,28) and rapidly reducing monocyte recruitment to existing atherosclerotic plaques, followed by decreased plaque macrophage contents.11) Thus, we hypothesized that the statininduced alterations in the intimal macrophage contents might be accompanied by reduced gal-3 expression in atherosclerotic plaques. We found that statin therapy dramatically reduced plaque gal-3 expression as well as plaque macrophage contents while statin failed to induce noticeable alterations in gal-1 expression. This finding suggests that gal-3 may be more associated with the pathogenesis of atherosclerosis and an ideal target to reflect changes in plaque inflammation. Remarkable statin responsiveness of gal-3 in addition to its higher serum levels and proportionally stronger expression of gal-3 as plaque inflammation worsens indicates that gal-3 may accurately reflect the degree of plaque inflammation in vivo. However, since this study did not examine the pathogenesis of atherosclerosis, we cannot draw a decisive conclusion on whether gals are proinflammatory or anti-inflammatory during the progression of atherosclerosis.
In conclusion, we demonstrate that gal-1 and gal-3 are the predominant gals in atherosclerotic plaques, and show their specific spatial and temporal intraplaque expression patterns during plaque growth. Especially, gal-3 is more abundantly distributed and characteristically colocalized with macrophages. As plaque progresses, gal-3 expression markedly increase, while as plaque regresses, the gal-3 content dramatically decreases. These findings suggest that gal-3 might be used as a marker for atherosclerotic plaque progression and regression, especially as an indicator of the statin response of in vivo atherosclerosis.
Figures and Tables
Fig. 1
A: immunoblots of different galectin proteins such as gal-1, gal-2, gal-3 and gal-8 in the aortas of C57BL/6 and apoE-/- mice. B: densitometry analyses of aortic gal proteins. C: serum gal-3 levels of 26-week-old chow diet-fed C57BL/6 (n=6) and high cholesterol diet-fed apoE-/- mice (n=6). apoE: apolipoprotein E.
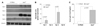
Fig. 2
Differential spatial expression pattern of macrophage, gal-1, and gal-3 proteins in atherosclerotic plaques of 26-week-old apoE-/- mice aortic roots (n=3). A: hematoxylin and eosin (H&E) staining. B, C and D: immunohistochemical (IHC) staining for macrophage, gal-1, and gal-3 proteins. apoE: apolipoprotein E.
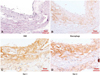
Fig. 3
Differential temporal expression pattern of gal-1 and gal-3 proteins in atherosclerotic plaques of 26-week-old apoE-/- mice versus 36-week-old apoE-/- mice (n=3). A: IHC staining for gal-1 protein in aortic roots of 26-week-old apoE-/- mice (left panel) versus 36-week-old apoE-/- mice (right panel). B: IHC staining for gal-3 protein in aortic roots of 26-week-old apoE-/- mice (left panel) vs. 36-week-old apoE-/- mice (right panel). C: quantitative histologic analyses of intraplaque gal-1 and gal-3 protein contents between 26-week-old apoE-/- mice and 36-week-old apoE-/- mice. IHC: immunohistochemical, apoE: apolipoprotein E.
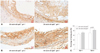
Fig. 4
Correlation analysis of in vivo plaque gal-3 protein and plaque macrophage content in the entire apoE-/-, mice cohort including 26-week-old, 36-week-old apoE-/- (n=3), treated with or without atorvastatin. apoE: apolipoprotein E.
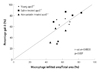
Fig. 5
Statin responsiveness of intraplaque gal-1 and gal-3 proteins in 36-week-old apoE-/- mice (n=3). A: representative aortic root sections of salinetreated apoE-/- mice for control and atorvastatin-treated apoE-/- mice. B: images (original magnification ×200) from left to right demonstrate adjacent sections of immunoreactive macrophage, immunoreactive gal-1, immunoreactive gal-3, and multicolor immunofluorescent macrophage (red) with gal-3 (green) proteins. C: quantitative histologic analyses of intraplaque gal-1 and gal-3 protein contents between saline-treated and atorvastatin-treated 36-week-old apoE-/- mice. D: immunoblots of different galectin proteins such as gal-1 and gal-3 in the aortas of saline-treated apoE-/- (n=3) mice and atorvastatin treated apoE-/- (n=3) mice. E: densitometry analyses of aortic gal-1 and gal-3 proteins. GAPDH: glyceraldehyde phosphate dehydrogenase, apoE: apolipoprotein E.
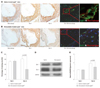
Acknowledgments
This journal was supported by National Research Foundation of Korea Grant funded by the Korea Government (NRF-2012R1A1A 2042674).
References
1. Libby P, Ridker PM, Hansson GK;. Leducq Transatlantic Network on Atherothrombosis. Inflammation in atherosclerosis: from pathophysiology to practice. J Am Coll Cardiol. 2009. 54:2129–2138.
2. Almkvist J, Karlsson A. Galectins as inflammatory mediators. Glycoconj J. 2004. 19:575–581.
3. Rubinstein N, Ilarregui JM, Toscano MA, Rabinovich GA. The role of galectins in the initiation, amplification and resolution of the inflammatory response. Tissue Antigens. 2004. 64:1–12.
4. Zanetta JP, Badache A, Maschke S, Marschal P, Kuchler S. Carbohydrates and soluble lectins in the regulation of cell adhesion and proliferation. Histol Histopathol. 1994. 9:385–412.
5. Rabinovich GA, Toscano MA, Ilarregui JM, Rubinstein N. Shedding light on the immunomodulatory properties of galectins: novel regulators of innate and adaptive immune responses. Glycoconj J. 2004. 19:565–573.
6. Moiseeva EP, Javed Q, Spring EL, de Bono DP. Galectin 1 is involved in vascular smooth muscle cell proliferation. Cardiovasc Res. 2000. 45:493–502.
7. Hughes RC. Secretion of the galectin family of mammalian carbohydrate-binding proteins. Biochim Biophys Acta. 1999. 1473:172–185.
8. Sano H, Hsu DK, Yu L, et al. Human galectin-3 is a novel chemoattractant for monocytes and macrophages. J Immunol. 2000. 165:2156–2164.
9. Iacobini C, Menini S, Ricci C, et al. Accelerated lipid-induced atherogenesis in galectin-3-deficient mice: role of lipoxidation via receptormediated mechanisms. Arterioscler Thromb Vasc Biol. 2009. 29:831–836.
10. Falcone C, Lucibello S, Mazzucchelli I, et al. Galectin-3 plasma levels and coronary artery disease: a new possible biomarker of acute coronary syndrome. Int J Immunopathol Pharmacol. 2011. 24:905–913.
11. Kircher MF, Grimm J, Swirski FK, et al. Noninvasive in vivo imaging of monocyte trafficking to atherosclerotic lesions. Circulation. 2008. 117:388–395.
12. Rabinovich GA, Baum LG, Tinari N, et al. Galectins and their ligands: amplifiers, silencers or tuners of the inflammatory response? Trends Immunol. 2002. 23:313–320.
13. Chen HY, Fermin A, Vardhana S, et al. Galectin-3 negatively regulates TCR-mediated CD4+ T-cell activation at the immunological synapse. Proc Natl Acad Sci U S A. 2009. 106:14496–14501.
14. MacKinnon AC, Farnworth SL, Hodkinson PS, et al. Regulation of alternative macrophage activation by galectin-3. J Immunol. 2008. 180:2650–2658.
15. Jeon SB, Yoon HJ, Chang CY, Koh HS, Jeon SH, Park EJ. Galectin-3 exerts cytokine-like regulatory actions through the JAK-STAT pathway. J Immunol. 2010. 185:7037–7046.
16. Forsman H, Islander U, Andréasson E, et al. Galectin 3 aggravates joint inflammation and destruction in antigen-induced arthritis. Arthritis Rheum. 2011. 63:445–454.
17. Nachtigal M, Al-Assaad Z, Mayer EP, Kim K, Monsigny M. Galectin-3 expression in human atherosclerotic lesions. Am J Pathol. 1998. 152:1199–1208.
18. Ozaki K, Inoue K, Sato H, et al. Functional variation in LGALS2 confers risk of myocardial infarction and regulates lymphotoxin-alpha secretion in vitro. Nature. 2004. 429:72–75.
19. Chellan B, Narayani J, Appukuttan PS. Galectin-1, an endogenous lectin produced by arterial cells, binds lipoprotein(a) [Lp(a)] in situ: relevance to atherogenesis. Exp Mol Pathol. 2007. 83:399–404.
20. Nachtigal M, Ghaffar A, Mayer EP. Galectin-3 gene inactivation reduces atherosclerotic lesions and adventitial inflammation in ApoE-deficient mice. Am J Pathol. 2008. 172:247–255.
21. Khallou-Laschet J, Varthaman A, Fornasa G, et al. Macrophage plasticity in experimental atherosclerosis. PLoS One. 2010. 5:e8852.
22. Liu FT, Rabinovich GA. Galectins: regulators of acute and chronic inflammation. Ann N Y Acad Sci. 2010. 1183:158–182.
23. Romaniuk MA, Tribulatti MV, Cattaneo V, et al. Human platelets express and are activated by galectin-8. Biochem J. 2010. 432:535–547.
24. Liu FT, Yang RY, Hsu DK. Galectins in acute and chronic inflammation. Ann N Y Acad Sci. 2012. 1253:80–91.
25. Arar C, Gaudin JC, Capron L, Legrand A. Galectin-3 gene (LGALS3) expression in experimental atherosclerosis and cultured smooth muscle cells. FEBS Lett. 1998. 430:307–311.
26. Moiseeva EP, Williams B, Samani NJ. Galectin 1 inhibits incorporation of vitronectin and chondroitin sulfate B into the extracellular matrix of human vascular smooth muscle cells. Biochim Biophys Acta. 2003. 1619:125–132.
27. Liu FT, Hsu DK, Zuberi RI, Kuwabara I, Chi EY, Henderson WR Jr. Expression and function of galectin-3, a beta-galactoside-binding lectin, in human monocytes and macrophages. Am J Pathol. 1995. 147:1016–1028.
28. Puato M, Faggin E, Rattazzi M, et al. Atorvastatin reduces macrophage accumulation in atherosclerotic plaques: a comparison of a nonstatin-based regimen in patients undergoing carotid endarterectomy. Stroke. 2010. 41:1163–1168.