Abstract
Background and Objectives
We evaluated which plaque components are associated with long-term clinical events in patients who underwent primary percutaneous coronary intervention (PCI).
Subjects and Methods
The study subjects consisted of 57 consecutive patients (mean age, 58.5±14.5 years; 45 males) who underwent primary PCI and a virtual histology-intravascular ultrasound examination. Major adverse cardiac events (MACEs) including death, acute myocardial infarction, stroke, and revascularization were evaluated during the mean 28 month follow-up period.
Results
Patients with high fibro-fatty volume (FFV >13.4 mm3, n=29; mean age, 61.3 years) had a lower ejection fraction (52.7% vs. 59.4%, p=0.022), a higher incidence of multi-vessel disease (69.0% vs. 28.6%, p=0.002), larger plaque area (25.7 mm2 vs. 15.9 mm2, p<0.001), and larger plaque volume (315 mm3 vs. 142 mm3, p<0.001) than those with a low FFV (≤13.4 mm3, n=28; mean age, 55.6 years). Patients with high FFV had a significantly higher incidence (32.1% vs. 8.3%, p=0.036) of MACE than those with low FFV. When we divided the study population according to the necrotic core volume (NCV), fibrous volume, or dense calcified volume, no significant findings in terms of demographics and MACE rates were observed. A Cox regression analysis revealed that the independent factor for MACE was FFV (hazard ratio, 6.748; 95% confidence interval, 1.168-38.971, p=0.033) in this study population.
Virtual histology-intravascular ultrasound (VH-IVUS) has the potential to provide detailed qualitative and quantitative information of coronary artery disease and can identify four specific coronary plaque components, such as fibrous (FT), fibro-fatty (FF), dense calcified (DC), and necrotic core (NC) regions using tissue mapping.1)
Necrotic core regions are important in vulnerable plaque2-4) and the number of NC regions is larger in patients with acute coronary syndrome (ACS) than patients with stable angina.5-7) Furthermore, NC is associated with positive remodeling.8)9) However, these findings have not always been consistent in previous studies.10)11)
A controversy exists as to whether NC or FF is associated with slow/no reflow after percutaneous coronary intervention (PCI).4)5)12-15) We previously found that fibro-fatty volume (FFV) is associated with slow flow in patients with acute myocardial infarction (AMI) who underwent primary PCI.12) But, other investigators have reported that NC is associated with slow flow in patients with ACS who underwent PCI.4)5)14)15) Some differences existed between the study populations in these studies. However, no study has reported which component is associated with long-term clinical outcomes.
Therefore, we evaluated which coronary plaque components are associated with long-term clinical outcomes using a post-hoc analysis from our previous study that showed a relationship between slow flow and plaque composition in patients with AMI who underwent primary PCI.12)
The study subjects consisted of 57 consecutive patients who underwent primary PCI for AMI and a VH-IVUS examination from July 2006 to July 2007. Exclusion criteria included vessel tortuosity, which precluded the VH-IVUS examination, a history of PCI or coronary artery bypass surgery, and refusal of patient consent. Patient demographics and laboratory data, including fasting lipid profiles, serum glucose, etc were obtained before primary PCI. Written informed consent was obtained from all patients, and the study was approved by the hospital ethics committee of Konyang University Hospital.
The primary endpoint was major adverse cardiac events (MACEs). Clinical follow-up was performed for a mean of 27.5±18.3 months, and follow-up data were obtained from all patients. MACE were documented by making phone calls (four patients) and by reviewing the hospital records (53 patients). MACE was defined as death, AMI, stroke, and revascularization. Death was defined as cardiac death due to ACS, intractable heart failure, malignant arrhythmia, and sudden cardiac death. AMI was defined based on the criteria of clinical presentation, electrocardiogram findings, and cardiac enzyme studies (new ST-segment elevation or depression, development of Q waves or left bundle branch block on electrocardiogram, or biochemical evidence of necrosis such as total creatine kinase >twice the normal upper limit with an elevated creatine kinase-MB isoenzyme or a positive troponin). Stroke was defined as a loss of neurological function caused by an ischemic event, and this loss persisted for more than 24 hours, left residual signs, or was shown by an imaging study. Revascularization was defined as target lesion revascularization (TLR) or PCI for a native, de novo coronary lesion. TLR was defined as ischemia-driven PCI of the target lesion due to restenosis or re-occlusion within the stent or in the adjacent 5 mm of the distal or proximal segments.
All patients were taking 200 mg/day aspirin plus 75 mg/day clopidogrel (after a 300 mg of clopidogrel loading dose) and received intravenous doses of unfractionated heparin during PCI titrated to maintain a therapeutic activated coagulation time.
Percutaneous coronary intervention was performed with a femoral or radial approach using a 6 Fr or 7 Fr guiding catheter and 0.014-inch standard or extra-support coronary guidewires. Balloon-exexpandable stents were successfully implanted in all patients after predilation with ballooning.
The IVUS examination methods were described previously.16) The VH-IVUS examination was performed with a dedicated VH-IVUS console (Volcano Therapeutics, Rancho Cordova, CA, USA) during the primary PCI after an intracoronary administration of 100 to 200 µg nitroglycerin. A 20-MHz, 2.9 F monorail, electronic Eagle Eye Gold IVUS catheter (Volcano Therapeutics) was advanced into the target lesion after wiring (n=36, 63.2%) or small sized (1.5 mm diameter) ballooning (n=21, 36.8%) to minimize the effect of ballooning on plaque morphology, and an automatic pullback at 0.5 mm/s was performed to the aorto-ostial junction. The VH-IVUS image was recorded on a DVD-ROM for later offline analysis. VH-IVUS uses a spectral analysis of IVUS radiofrequency data to construct a tissue map.
The grey-scale IVUS images were qualitatively and quantitatively analyzed according to the criteria of the American College of Cardiology Clinical Expert Consensus Document on IVUS.17) Reference segments were analyzed in a similar manner to the target site. The proximal and distal reference segments were the most normal-looking cross sections ≤10 mm distal and proximal to the lesion. The edges of the lesion for determining lesion length were defined as the nearest point of the most normal-looking cross sections ≤10 mm distal and proximal to the lesion. Reference data were the average of the proximal and distal reference segments. Plaque rupture was defined as an ulcerated ruptured plaque contained in a cavity that communicated with the lumen and an overlying residual fibrous cap fragment. The ruptured cavity area was measured using manual interrogation by tracing the leading edge of the cavity and the presumptive border with the lumen in a single image slice with the largest cavity size.12) The volume of the ruptured cavity was measured by multiplying 0.05 mm by the sum of all the cavity areas in the image slices showing a ruptured cavity.
These analyses were conducted on the target lesion of the infarct-related artery using customized software (IVUSLab; Volcano Therapeutics) by either examiner (Bae JH and Kwon TG) who was unaware of the patient clinical characteristics. The border was automatically detected at the predefined lesion segment for both the lumen and the media-adventitia interface. Then, border detection was manually corrected in the lesion again after automatically detecting the border. After confirming border detection, the software automatically calculated and presented the results. For each frame, histological findings were expressed in colors, as previously described (green for FT, green-yellow for FF, white for DC, and red for NC). Additionally, the area (mm2) and percentage of each plaque tissue component was expressed as well as the volumetric analysis (mm3). The predictive accuracy of this method with tissue mapping has been validated.1)
All analyses were performed with Statistical Package for the Social Sciences (SPSS) version 18.0 (SPSS Inc., Chicago, IL, USA). Values are expressed as mean±SD. We used the Student's t-test and chi-square test to compare the groups according to the variables. Relevant baseline clinical and VH-IVUS characteristics showing a significant difference (p<0.05) were included in the multivariate analysis. Forward-stepwise Cox regression analysis was performed to evaluate the independent factors of long-term clinical outcomes in study patients. The Kaplan-Meier method was used to analyze event-free survival. Statistical significance was inferred at p<0.05.
The study subjects were divided into two groups based on the median value of each coronary plaque component; patients with high FFV (>13.4 mm3, n=29; 24 males; mean age, 61.3±15.1 years) and patients with low FFV (≤13.4 mm3, n=28; 21 males; mean age, 55.6±13.5 years) by the median FFV value. Baseline characteristics are summarized in Table 1. No significant differences were observed for age, gender, coronary artery disease risk factors, clinical presentation, medications, lipid profile, or cardiac enzymes. The group with a higher FFV had a lower left ventricular ejection fraction (52.7±10.4% vs. 59.4±10.3%, p=0.022). When we divided the study population according to median value of the necrotic core volume (NCV), FV, or dense calcified volume (DCV), no significant findings were observed in terms of demographics and MACE rate (data not shown).
Major adverse cardiac event rates are listed in Table 2. MACE rates tended to be higher in the high FFV group, but the result was not statistically significant. However, patients with a high FFV had a significantly higher incidence (32.1% vs. 8.3%, p=0.036) of total MACE (death, AMI, stroke, revascularization) than those in the low FFV group. The Kaplan-Meier survival estimates showed worse outcomes in the high FFV group than those in the low FFV group (log-rank p=0.036) (Fig. 1). No stent thrombosis was noted in either group during the follow-up. The univariate Cox regression analysis showed that significant predictors for total MACE were FFV and plaque burden. The multivariate Cox regression analysis, including all significant variables as covariates, revealed that FFV was the only significantly independent predictor for MACE (hazard ratio, 6.748; 95% confidence interval, 1.168-38.971, p=0.033) (Table 3).
When we divided the study population according to the absolute
and relative FV, NCV, and DCV, no significant findings were
observed in terms of demographics or MACE rate.
The coronary angiography findings are summarized in Table 4. No significant differences were observed in terms of lesion location, reference diameter, type and size of stent, inflation pressure, time of VH-IVUS examination, prevalence of thrombus, or Thrombolysis in Myocardial Infarction flow grade. However, multi-vessel disease was encountered more often in patients with high FFV than those with low FFV (69% vs. 28%, p=0.002). Stent length was significantly longer in the high FFV group compared with that in the low FFV group (25.07±4.71 mm vs. 21.23±4.19 mm, p=0.002). When we compared the angiography findings according to the median value of FV, DCV, and NCV, we found no significant differences between the groups.
The VH-IVUS findings are summarized in Table 5. IVUS lesion length was significantly longer in the high FFV group compared with that in the low FFV group (25.71±8.33 mm vs. 15.97±6.02 mm, p<0.05).
A lesion analysis at the minimal lumen area site revealed that patients with high FFV had larger vessel area (17.91±5.37 mm2 vs. 12.19±3.89 mm2, p<0.001), larger lumen area (4.10±1.16 mm2 vs. 3.78±1.25 mm2, p=0.017), larger plaque area (15.90±4.65 mm2 vs. 10.61±4.30 mm2, p<0.05), and a larger fibrous area (7.43±3.00 mm2 vs. 4.45±2.73 mm2, p<0.05) than those in the low FFV group.
Patients with high FFV had larger vessel volume (380.9±172.9 mm3 vs. 197.3±86.9 mm3, p<0.001), larger plaque volume (314.5±141.4 mm3 vs. 141.6±71.6 mm3, p<0.05), larger plaque burden (61.7±5.1% vs. 57.5±8.1%, p=0.021), larger FV (131.3±69.3 mm3 vs. 49.9±28.4 mm3, p<0.05), and larger NCV (35.2±29.2 mm3 vs. 21.1±17.3 mm3, p=0.032) over the entire lesion length than those in the lower FFV group.
The VH volumetric data of each plaque constituent were divided by the length of the examined vessel segment to compensate for the different lesion length in each examined vessel. The FV, FFV, plaque volume, and vessel volume after adjustment for the different examined vessel segments were larger in the high FFV group (4.97±1.67 mm3/mm vs. 3.11±1.63 mm3/mm, p<0.001; 1.53±0.99mm3/mm vs. 0.54±0.62 mm3/mm, p<0.001; 12.14±3.27 mm3/mm vs. 8.77±2.95 mm3/mm, p<0.001; 19.62±4.87% vs. 15.03±4.16%, p<0.001, respectively). However, no significant difference in the NCV and DCV was observed between the groups after adjusting for lesion length (1.33±0.98 mm3/mm vs. 1.20±0.78 mm3/mm, p=0.591). No significant difference was observed for the remodeling index, the prevalence of plaque rupture, or ulceration between the two groups.
Finally, we performed an receiver-operating characteristic curve analysis to calculate an FFV cutoff value for future MACE in these patients. The absolute FFV optimal cut-off point to predict future MACE was 12.3 mm3. As shown in Fig. 2, this cut-off point had a sensitivity of 90.9% and a specificity of 48.8% (area under the curve, 0.704, p=0.038, 95% confidence interval, 0.526-0.882) (Fig. 2).
The main finding of our study was that high FFV over the entire lesion length was an important predictor of long-term clinical outcome in patients with AMI who underwent primary PCI, although it is still unclear whether FFV or NCV is associated with slow/no-reflow in previous cross-sectional studies.4)5)12-15)
The slow/no reflow phenomenon during PCI is associated with increased in-hospital mortality, post-procedural myocardial infarction, and poor mid-term clinical outcomes.18-20) However, controversies exist for the association between plaque components and slow/no reflow phenomenon after PCI in patients with ACS.5)12-15)21) Kawaguchi et al.5) reported that NC volume clearly predicts distal embolization after stent deployment in patients with an ST-segment elevation MI, as compared with FT, FF, DC, and total plaque volume. Bae et al.12) reported that patients with slow flow had more FT and FF volumes, but not NC volume, at the time of primary PCI for AMI. Nakamura et al.13) reported that VH-IVUS showed a trend towards a larger percentage of FF plaque volume in a no-reflow group than that in a normal-reflow group. Hong et al.15) also reported that NC is associated with slow flow after PCI in patients with ACS. Thus, no consensus exists regarding plaque composition associated with slow flow.
Several studies have demonstrated that NC is larger in patients with ACS than in patients with stable angina.5-7) Three studies5-7) reported that the amount of NC or the percentage of NC and its ratio to DC are larger in patients with ACS compared to those with stable angina. There is also discrepancy with regards to vascular remodeling. That is, positive remodeling, which is an adverse prognostic factor, is associated with NC,8)9) whereas others have reported it is associated with FFV.10)11) Therefore, it is still unclear whether NCV or FFV is associated with ACS, positive remodeling, and slow flow in several cross-sectional studies.2-11)
Few studies have investigated the impact of coronary plaque components on long-term clinical events in patients who underwent PCI. Therefore, this study was conducted to evaluate the impact of plaque components on long-term clinical events in a homogenous study population, particularly in patients who underwent primary PCI. Our study showed that FFV rather than NCV was associated with long-term clinical outcome. The reason why FFV, not NCV, was associated with total MACE in our study was the greater chance for an NCV artifact, particularly by calcium. That is, there was a greater chance to be read as NCV behind calcium. The second reason was that there was a larger number of already ruptured plaque cavities, which precluded true measurement of NCV in the lesion. A fresh thrombus is composed of a layered pattern of platelet fibrin, erythrocytes, and intact granulocytes, and an old thrombus shows in-grown smooth muscle cells with or without deposition of connective tissue and capillary vessel in-growth, which is similar to a fibrous plaque.22)23)
The current study had some limitations. This study was based on a small number of patients, and was a single-center study. However, our study was based on consecutive patients with AMI who underwent primary PCI, although we did not reflect the whole spectrum of patients with ACS. The other limitation considers thrombi. Actually, VH-IVUS cannot identify a thrombus that commonly follows a plaque rupture. Most lesions (53 of 57, 94.7%) in the current study had already ruptured or became ulcerated plaques, whose cavity was linked to the lumen. Nasu et al.24) have shown that thrombi are colored as FT and FF by VH-IVUS. Therefore, we analyzed the lesions, excluding the plaque cavity to avoid any possible thrombus; thus, a larger amount of NCV may have migrated into the distal coronary bed before or during primary PCI in the patients with AMI. Thus, there was possibility of failing to detect a higher NCV and of underestimating NCV. Finally, we arbitrarily divided the study population into two groups according to the median value of plaque components, because we focused only on the clinical outcomes according to coronary plaque components, and there was no cutoff value or appropriate value for absolute plaque components until now.
Our results suggest that plaque components, particularly FFV, but not NCV, are important in the long-term clinical outcomes of patients who underwent primary PCI, although further studies are needed to evaluate the relationship between coronary plaque components and long-term clinical outcomes. A VH-IVUS analysis for plaque components over the entire lesion may be useful to predict the risk of long-term clinical outcomes in patients with AMI who underwent primary PCI. Lesions with high FFV should trigger more aggressive secondary prevention in patients with AMI who underwent primary PCI.
Figures and Tables
Fig. 1
Kaplan-Meier survival curve. Low FFV ≤13.4 mm3; high FFV >13.4 mm3. MACE: major adverse cardiac events, FFV: fibrofatty volume, AMI: acute myocardial infarction, PCI: percutaneous coronary intervention, TLR: target lesion revascularization.
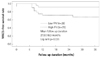
Table 1
Study subject clinical characteristics
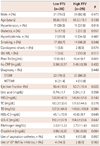
Low FFV ≤13.4 mm3; high FFV >13.4 mm3. FFV: fibrofatty volume, TnI: troponine I, hs-CRP: high sensitivity C-reactive protein, STEMI: ST elevation myocardial infarction, NSTEMI: non-ST elevation myocardial infarction, FBS: fasting blood sugar, TC: total cholesterol, TG: triglyceride, HDL-C: high density lipoprotein-cholesterol, LDL-C: low density lipoprotein-cholesterol, GP IIb/IIIa inhibitor: glycoprotein IIb/IIIa inhibitor, MI: myocardial infarction
References
1. Nair A, Kuban BD, Tuzcu EM, et al. Coronary plaque classification with intravascular ultrasound radiofrequency data analysis. Circulation. 2002. 106:2200–2206.
2. Virmani R, Burke AP, Kolodgie FD, Farb A. Vulnerable plaque: the pathology of unstable coronary lesions. J Interv Cardiol. 2002. 15:439–446.
3. Rodriguez-Granillo GA, McFadden EP, Valgimigli M, et al. Coronary plaque composition of nonculprit lesions, assessed by in vivo intracoronary ultrasound radio frequency data analysis, is related to clinical presentation. Am Heart J. 2006. 151:1020–1024.
4. Böse D, von Birgelen C, Zhou XY, et al. Impact of atherosclerotic plaque composition on coronary microembolization during percutaneous coronary interventions. Basic Res Cardiol. 2008. 103:587–597.
5. Kawaguchi R, Oshima S, Jingu M, et al. Usefulness of virtual histology intravascular ultrasound to predict distal embolization for ST-segment elevation myocardial infarction. J Am Coll Cardiol. 2007. 50:1641–1646.
6. Missel E, Mintz GS, Carlier SG, et al. Necrotic core and its ratio to dense calcium are predictors of high-risk non-ST-elevation acute coronary syndrome. Am J Cardiol. 2008. 101:573–578.
7. Hong MK, Mintz GS, Lee CW, et al. Comparison of virtual histology to intravascular ultrasound of culprit coronary lesions in acute coronary syndrome and target coronary lesions in stable angina pectoris. Am J Cardiol. 2007. 100:953–959.
8. Rodriguez-Granillo GA, Serruys PW, Garcia-Garcia HM, et al. Coronary artery remodelling is related to plaque composition. Heart. 2006. 92:388–391.
9. Kataoka T, Mathew V, Rubinshtein R, et al. Association of plaque composition and vessel remodeling in atherosclerotic renal artery stenosis: a comparison with coronary artery disease. JACC Cardiovasc Imaging. 2009. 2:327–338.
10. Fujii K, Carlier SG, Mintz GS, et al. Association of plaque characterization by intravascular ultrasound virtual histology and arterial remodeling. Am J Cardiol. 2005. 96:1476–1483.
11. Surmely JF, Nasu K, Fujita H, et al. Association of coronary plaque composition and arterial remodelling: a virtual histology analysis by intravascular ultrasound. Heart. 2007. 93:928–932.
12. Bae JH, Kwon TG, Hyun DW, Rihal CS, Lerman A. Predictors of slow flow during primary percutaneous coronary intervention: an intravascular ultrasound-virtual histology study. Heart. 2008. 94:1559–1564.
13. Nakamura T, Kubo N, Ako J, Momomura S. Angiographic no-reflow phenomenon and plaque characteristics by virtual histology intravascular ultrasound in patients with acute myocardial infarction. J Interv Cardiol. 2007. 20:335–339.
14. Higashikuni Y, Tanabe K, Tanimoto S, et al. Impact of culprit plaque composition on the no-reflow phenomenon in patients with acute coronary syndrome: an intravascular ultrasound radiofrequency analysis. Circ J. 2008. 72:1235–1241.
15. Hong YJ, Jeong MH, Choi YH, et al. Impact of plaque components on no-reflow phenomenon after stent deployment in patients with acute coronary syndrome: a virtual histology-intravascular ultrasound analysis. Eur Heart J. 2009. doi: 10.1093/eurheartj/ehp034.
16. Tobis JM, Mallery J, Mahon D, et al. Intravascular ultrasound imaging of human coronary arteries in vivo: analysis of tissue characterizations with comparison to in vitro histological specimens. Circulation. 1991. 83:913–926.
17. Mintz GS, Nissen SE, Anderson WD, et al. American College of Cardiology Clinical Expert Consensus Document on Standards for Acquisition, Measurement and Reporting of Intravascluar Ultrasound Studies (IVUS): a report of the American College of Cardiology Task Force on Clinical Expert Consensus Documents. J Am Coll Cardiol. 2001. 37:1478–1492.
18. Resnic FS, Wainstein M, Lee MK, et al. No-reflow is an independent predictor of death and myocardial infarction after percutaneous coronary intervention. Am Heart J. 2003. 145:42–46.
19. Ramírez-Moreno A, Cardenal R, Pera C, et al. Predictors and prognostic value of myocardial injury following stent implantation. Int J Cardiol. 2004. 97:193–198.
20. Yip HK, Chen MC, Chang HW, et al. Angiographic morphologic features of infarct-related arteries and timely reperfusion in acute myocardial infarction: predictors of slow-flow and no-reflow phenomenon. Chest. 2002. 122:1322–1332.
21. Kawamoto T, Okura H, Koyama Y, et al. The relationship between coronary plaque characteristics and small embolic particles during coronary stent implantation. J Am Coll Cardiol. 2007. 50:1635–1640.
22. Henriques de Gouveia R, van der Wal AC, van der Loos CM, Becker AE. Sudden unexpected death in young adults: discrepancies between initiation of acute plaque complications and the onset of acute coronary death. Eur Heart J. 2002. 23:1433–1440.
23. Murakami T, Mizuno S, Takahashi Y, et al. Intracoronary aspiration thrombectomy for acute myocardial infarction. Am J Cardiol. 1998. 82:839–844.
24. Nasu K, Tsuchikane E, Katoh O, et al. Accuracy of in vivo coronary plaque morphology assessment: a validation study of in vivo virtual histology compared with in vitro histopathology. J Am Coll Cardiol. 2006. 47:2405–2412.