Abstract
Background and Objectives
Endothelial dysfunction is widely observed in diabetes mellitus, resulting in diabetic vascular complications. Krüppel-like factor 2 (KLF2) is implicated as being a key molecule that maintains endothelial function. We evaluated the expression of KLF2 in endothelial cells cultured in high glucose and investigated its functional implication in a diabetic animal model.
Subjects and Methods
Human umbilical vein endothelial cells (HUVECs) were cultured in physiologically high glucose (35 mM) condition. The Otsuka Long Evans Tokushima Fatty (OLETF) strain of rat was used as an excellent model of obese type II diabetes, and their lean littermates are Long Evans Tokushima Otsuka (LETO) rats.
Results
In HUVECs cultured in physiologically high glucose condition, FOXO1 was activated whereas KLF2 and endothelial nitric oxide synthase (eNOS) expression was near completely abolished, which was completely reversed by FOXO1 small interfering ribonucleic acid. In the vessels harvested from the OLETF rats, the animal model of type II diabetes, KLF2 and eNOS expression were found depleted. When vascular remodeling was induced in the left common carotid artery by reduction of blood flow with partial ligation of the distal branches, greater neointimal hypertrophy was observed in OLETF rats compared with the control LETO rats.
Diabetes mellitus is considered to be a 'cardiovascular disease equivalent' causing various vascular complications.1) Endothelial dysfunction is widely observed in diabetes mellitus, which results in various cardiovascular complications. In diabetes, endothelial dysfunction is characterized by a reduced availability of endothelium-derived vasodilating factors, such as nitric oxide.2)3) Impaired endothelium-dependent vasodilation has been demonstrated in patients with type 1 and 2 diabetes4)5) as well as in different animal models of diabetes.3)6) Moreover, endothelial dysfunction is induced after relatively short periods of high glucose treatment.7) In the natural history of type 2 diabetes, endothelial dysfunction is even considered to predate the onset of hyperglycemia.8)
Recently, Krüppel-like factor 2 (KLF2) has been reported to play an important role in the maintenance of endothelial function. KLF2 affects the expression of various vasoprotective molecules including endothelial nitric oxide synthase (eNOS) that confers anti-inflammatory, anti-thrombotic, and anti-proliferative effects.9) During the investigation of KLF2, we found that KLF2 contains forkhead factor binding motifs in its promoter region.10) This was of interest because dysregulated, chronic FOXO1 activation with prolonged hyperglycemia was suggested to cause pancreatic β-cell failure and hyperglycemia in type 2 diabetes.11)12) The presence of the forkhead factor binding motifs in the KLF2 promoter region and its observed function in endothelial cells led to the hypothesis that KLF2 may be suppressed under hyperglycemic conditions and that this phenomenon may be regulated by FOXO1. In this study, we evaluated the expression of KLF2 in endothelial cells cultured in high glucose and investigated its functional implication in a diabetic animal model.
Four to six passage human umbilical vein endothelial cells (HUVECs, Lonza) were plated onto 2% gelatin-coated dishes (Sigma) and maintained in EGM kit (Lonza). HUVECs were cultured using various concentrations of glucose. For shear stress experiments, confluent HUVECs were exposed to unidirectional laminar flow using an orbital shaker (FinePCR co. SH30).
Changes in ribonucleic acid (RNA) expression of eNOS, FOXO1, and KLF2 were determined by reverse transcriptase-polymer chain reaction (RT-PCR) as previously described.13) Primers used were: eNOS (forward primer: 5'-CCA GCT AGC CAA AGT CAC CAT-3; reverse primer: 5'-GTC TCG GAG CCA TAC AGG ATT-3'), FOXO1 (forward primer: 5'-GAA GAC ACC TGT ACA AGT GCC-3; reverse primer: 5'-CTG CTT CTC TCA GTT CCT GCT-3'), KLF2 (forward primer: 5'-CGG CAA GAC CTA CAC CAA GAG-3; reverse primer: 5'-CGC ACA GAT GGC ACT GGA AT-3'), and GAPDH (forward primer: 5'-CGT GGA AGG ACT CAT GAC-3; reverse primer: 5'-CAA ATT CGT TGT CAT ACC AG-3'). Total RNAs were isolated by the Trizol (Invitrogen) method, reverse-transcribed with a reverse transcription system (Promega), and amplified with primers for each molecule and GAPDH.
Immunoblot analysis was performed by modification of the protocol described in our previous work.13) The primary antibodies used were: anti-KLF2 (Santa Cruz Biotechnology, Inc.), anti-FOXO1 (Santa Cruz Biotechnology, Inc.), anti-eNOS (Santa Cruz Biotechnology, Inc.) and anti-α-tubulin (Oncogene). As a secondary antibody, anti-mouse IgG HRP (Molecular Probes) or anti-rabbit IgG HRP (Molecular Probes) was used at a dilution of 1:2000.
For the blockage of FOXO1, siFOXO1 (Samchully Pharm. Co., Ltd., Korea) was used. Control small interfering ribonucleic acid (siRNA) was purchased from Santa Cruz Biotechnology. SiRNA (30 nM) was transfected to HUVECs at room temperature with the use of Metafectene Pro (Bionex).
The experimental protocol was designed in accordance with the Guide for Experimental Animal Research issued by the Laboratory for Experimental Animal Research, Clinical Research Institute, University Hospital. The Otsuka Long Evans Tokushima Fatty (OLETF) strain of rat is an excellent model for obese type II diabetes, characterized by hypertension, hypertriglyceridemia, hyperinsulinemia, hyperglycemia, insulin resistance, and abundant visceral fat with 100% affected by diabetes by 25 weeks.14) Their lean littermates were Long Evans Tokushima Otsuka (LETO) rats. Male OLETF rats and LETO rats (6 weeks old) were a gift from Otsuka Pharmaceuticals (Tokushima, Japan). We started the animal experiment with 5 rats in each group, however, one of each group died during the experiment. Therefore, final analysis was performed in 4 rats in each group.
For immunostaining of KLF2, the abdominal aortic rings harvested at the celiac trunk bifurcating levels were treated with 3% H2O2, washed with phosphate buffered saline, blocked with 1.5% horse serum, and followed by incubation with a goat-anti-KLF2 antibody (Santa Cruz Biotechnology, Inc.), biotinylated secondary antibody against goat IgG (Vector Laboratories), avidin DH, and biotinylated horseradish peroxidase H reagents (Vectastain ABC reagents, Vector Laboratories). Hematoxylin and eosin staining was used to reveal the cell nuclei and cytoplasm. The results were examined by light microscopy, and images were acquired using a digital camera. For the induction of vascular remodeling, rats were anesthetized with a mixture of ketamine and xylazine and maintained at 37℃. Blood flow in the left common carotid artery was reduced by partial ligation of the left external and internal carotid arterial branches as previously described.15) The branches of the right carotid artery were exposed but not ligated (sham operation). The animals were allowed to recover for 7 days and then sacrificed. Both carotid artery branches were harvested and embedded in paraffin. Cross sections (4 µm) were processed with hematoxylin/eosin and analyzed by use of MCID image software (Imaging Research Inc) as described previously.16)
We expressed data as mean±SEM. The unpaired 2-tailed t-test between the two groups and analysis of variance for multiple comparisons was used to compare continuous variables. Statistical comparisons of continuous variables between ligation-operated and sham-operated arteries were performed using a paired t-test for comparison for intra-group comparisons and a Student's t-test for inter-group comparisons. All calculations were performed using Statistical Package for the Social Sciences (SPSS) 13.0 (SPSS Inc.). P<0.05 was considered statistically significant.
First, we cultured HUVECs at high concentrations of glucose ranging from 5 mM to 100 mM. We observed no changes of cell viability until day 3 by 35 mM of glucose. However, above 60 mM glucose cytopathic change was observed. Therefore, we determined 35 mM as physiologically high glucose for further experiments (Fig. 1).
Krüppel-like factor 2 expression significantly decreased in high glucose conditions in a dose-dependent manner, and eNOS expression was also suppressed (Fig. 2A and C). Then, we serially evaluated the expression of FOXO1 and KLF2 under physiologically high glucose conditions. FOXO1 became unphosphorylated, whereas there was no change in the total FOXO1 amount, which indicated that it was activated. In contrast, KLF2 was almost completely diminished from 6 hours after glucose treatment (p<0.05) (Fig. 2B and D).
Next, we evaluated the effect of high glucose under shear stress, which is one of the main physiologic stimuli of KLF2.9) A total of 12 dynes/cm2 of shear stress was applied for 24 hours using an orbital shaker. HUVECs exposed to the shear stress aligned following the flow direction, validating that shear stress was adequately applied (Fig. 3A). Shear stress induces KLF2 in HUVECs, followed by eNOS activation. However, both KLF2 and eNOS were significantly reduced with high glucose even under shear stress conditions (Fig. 3B).
Because KLF2 has the forkhead factor binding motifs in its promoter sequences and its suppression in high glucose was temporally well correlated with FOXO1 activation, we evaluated the role of FOXO1 in KLF2 regulation. When we inhibited FOXO1 by siRNA, KLF2 expression, which was suppressed in high glucose, recovered to a greater extent than the basal status. eNOS expression also recovered by FOXO1 siRNA and correlated with the expression pattern of KLF2 (Fig. 4).
In order to confirm our in vitro findings, we performed the animal experiment using OLETF rats as animal models of insulin resistant, type 2 diabetes. As compared to control LETO rats, the OLETF rats showed more than two-fold higher serum glucose levels. In addition, the body weight was also significantly greater in the OLETF rats than in the LETO rats. The serum triglyceride and total cholesterol levels in the OLETF rats were significantly higher than those of the LETO rats (Table 1). As was consistent with in vitro findings, KLF2 expression in the carotid arteries of the OLETF rats was significantly reduced compared to those of the control LETO rats (Fig. 5).
Next, we evaluated the expression of KLF2 at the branching point (bifurcation point) between the abdominal aorta and the celiac artery of the indicated rats. In the flow-dividing side of the bifurcation point of abdominal aorta, where the shear stress is high and more laminar, the expression level of KLF2 was reported to be more prominent compared to the lateral side (Fig. 6A).17) In the OLETF rats, KLF2 expression at the luminal lining was confined to the flow-dividing side of the bifurcation point and its circumference of expression was smaller than in the LETO rats (Fig. 6B and C).
In this carotid artery ligation mouse model, neointimal formation was reported to occur in association with reduced luminal area at the proximal portion of the ligated site.15) In the non-ligated contralateral side of carotid artery, there were no significant differences in intima/media ratio between OLETF rats and the control LETO rats. However, in the ligated side, the neointimal area significantly increased in OLETF rats compared to control LETO rats 1 week after the ligation (Fig. 7).
The major finding of our study was that KLF2 was suppressed under high glucose conditions. This study also had several additional, novel findings. First, FOXO1 in ECs was activated under high glucose conditions, which could mediate KLF2 suppression. Second, this suppression of KLF2 was followed by the suppression of its important downstream molecule, eNOS. Third, by using the OLETF rats as animal models of insulin resistant type 2 diabetes, we showed these in vitro findings could be extrapolated to an in vivo situation. Finally, we showed that this suppression of KLF2 in OLETF rats may increase neointimal formation as well as intraluminal thrombus formation.
Endothelial dysfunction is regarded as an initial critical mechanism of vascular damage in various cardiovascular diseases including diabetes. In diabetes, endothelial dysfunction is characterized by decreased eNOS activity.2) KLF2 is regarded as the main and most potent inducer of eNOS expression. Here we showed that KLF2 expression was markedly suppressed in high glucose. Moreover, we found for the first time that FOXO1 activated by high glucose is a negative transcriptional regulator of KLF2 leading to suppression of eNOS expression and vascular dysfunction in a diabetic animal model. This high glucose-FOXO1-KLF2 pathway may exert more profound effects in diabetic vasculopathy considering that even nearly half of the flow-regulated genes are dependent on KLF2.18)
In this study, we intended to evaluate the functional implication of KLF2 suppression via the induction of vascular remodeling in vivo. We assessed the change of KLF2 expression at the bifurcation point between abdominal aorta and celiac trunk, because this location has both the 'atherosclerosis-resistant flow-dividing side' with high shear stress and the 'atherosclerosis-prone lateral side' with disturbed low shear stress, and thus is good for observation of KLF2 expression change.17) In the diabetic animal model, KLF2 expression significantly decreased, which was only maintained at the flow-dividing side. This implicated that the resistance to turbulent flow at the bifurcation region may be impaired in diabetes. Moreover, one case of total carotid occlusion with thrombus was observed in the OLETF rat group. Of course, we cannot make a definitive conclusion with only this one case, but considering the anti-thrombotic effect of KLF2/eNOS, it seemed to be a very relevant finding.
The main limitation of this study is that we did not present the data of specific replenishing experiments of KLF2 in diabetic rats due to the lack of lentiviral vector. However, the findings of this study do suggest that KLF2 suppression, at least partially, may be responsible for impaired endothelial function in a diabetic condition.
The findings of this study are in accordance with many previous clinical findings in diabetic patients. In diabetic patients, even without preclinical or clinical atherosclerosis, endothelial function is already disturbed.19) Also, the prevalence of complex coronary lesions, such as bifurcation lesions, is more than two fold higher in diabetic patients.20) In addition, despite intervention with stents, diabetic patients have a greater than three times higher risk of stent thrombosis and major adverse cardiac events.21) KLF2 suppression under high glucose conditions may be a possible mechanism that explains the pathogenesis of such diabetic vascular complications.
Lastly, the findings of this study can have clinical implications on adopting the KLF2 replenishing strategy in diabetic patients for preventing diabetic vascular dysfunction. For example, KLF2 has recently been reported to be induced by several 3-hydroxy-3-methylglutaryl coenzyme A reductase inhibitors (statins) independent of its cholesterol lowering capacity.22-24) In this aspect, the findings of this study suggest the implication of intensive statin treatment in preventing diabetic vascular dysfunction. Another promising strategy is exercise training which can increase shear flow through the vessel lumen, subsequently introducing the physiologically relevant stimulus that augments endothelial KLF2 expression.25)
In conclusion, KLF2 suppression in endothelial cells by high glucose is a plausible mechanism of diabetic endothelial dysfunction. Therefore, the strategy of replenishing KLF2 may be effective for In conclusion, KLF2 suppression in endothelial cells by high glucose is a plausible mechanism of diabetic endothelial dysfunction. Therefore, the strategy of replenishing KLF2 may be effective for preventing diabetic vascular dysfunction.
Figures and Tables
Fig. 1
Morphologic changes of HUVECs 72 hours after culture under high glucose conditions. HUVECs were cultured in high glucose with the indicated concentration. HUVECs: human umbilical vein endothelial cells.
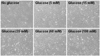
Fig. 2
Activation of FOXO1 and inhibition of KLF2 under high glucose conditions. A: KLF2 and eNOS protein expression at the indicated concentration of glucose. B: serial immunoblot analysis of phosphorylated FOXO1 on serine 256 (p-FOXO1), total FOXO1 (t-FOXO1), and KLF2 expression in endothelial cells incubated under physiologically high glucose conditions (35 mM). C: quantification of triplicate experiments of A by densitometry. D: quantification of triplicate experiments of B by densitometry. *p<0.05 compared to the control, †p<0.05 compared to the value of 36 hours. KLF2: Krüppel-like factor 2, eNOS: endothelial nitric oxide synthase.
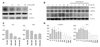
Fig. 3
Inhibition of shear flow-induced KLF2 expression under high glucose conditions. A: morphologic changes in HUVECs after shear stress application (24 hours, 12 dynes/cm2). B: immunoblot analysis evaluating the impact of shear stress and high glucose on the expression of KLF2 and eNOS. KLF2: Krüppel-like factor 2, HUVECs: human umbilical vein endothelial cells, eNOS: endothelial nitric oxide synthase.
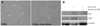
Fig. 4
Suppression of KLF2 and eNOS by FOXO1 in endothelial cells. A: representative RT-PCR analysis figure evaluating blocking effect of FOXO1 by FOXO1 siRNA on KLF2 and eNOS mRNA expression in high glucose condition. B-D: quantification of triplicate experiments of A. *p<0.05 compared to the control. KLF2: Krüppel-like factor 2, eNOS: endothelial nitric oxide synthase, RT-PCR: reverse transcriptase-polymer chain reaction, siRNA: small interfering ribonucleic acid, mRNA: messenger ribonucleic acid.
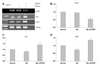
Fig. 5
Suppression of KLF2 in the blood vessels from diabetic, OLEFT rats. Representative immunofluorescent microscopic images of carotid arteries stained with KLF2 (colored-red) in the OLETF rats (left) and the control LETO rats (right). KLF2: Krüppel-like factor 2, OLEFT: Otsuka Long Evans Tokushima Fatty, LETO: Long Evans Tokushima Otsuka.
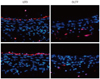
Fig. 6
Comparison of KLF2 expression in endothelial cells at abdominal-celiac branch point between OLETF and control LETO rats. A: schematic showing the branch point of the celiac artery off the abdominal aorta. The line shows the section through which the samples were taken for immunohistochemical examination of KLF2 expression. B: representative photographs of immunofluorescent staining of blood vessels with KLF2 in the OLETF rats and the LETO rats. KLF2 expression is stained red at the branching point between the abdominal aorta and the celiac artery of the indicated rats. Arrow indicates 'atherosclerosis-resistant flow-dividing' side and 'atherosclerosis-prone lateral' side. C: semi-quantitative graph of KLF2-stained luminal circumferential length (4 rats in each group). KLF2: Krüppel-like factor 2, OLEFT: Otsuka Long Evans Tokushima Fatty, LETO: Long Evans Tokushima Otsuka.
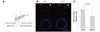
Fig. 7
Evaluation of neointimal formation after induction of vascular remodeling. A: representative photomicrographs of common carotid arteries sections from the indicated rats 1 week after ligation. At the left are photographs from the sham-operated artery. At the right are photographs from the ligation-operated artery. Yellow arrow indicates intima. B: comparison of intimal thickness. n=8 (two slide sections in one carotid artery sample) in each group (4 rats in each group). Statistical comparisons between ligation-operated and sham-operated arteries were performed using a paired t-test for comparison for intra-group comparisons and a Student's t-test for inter-group comparisons. OLEFT: Otsuka Long Evans Tokushima Fatty, LETO: Long Evans Tokushima Otsuka.
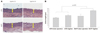
Acknowledgments
This work was supported by research grant for the young investigator from the Korean Society of Cardiology (2007) and by research grant from the Seoul National University Hospital (Lee HY). This study was also partially supported by National Research Foundation grant funded by the Korea government (MEST) (2010-0020257, Kim HS).
References
1. Haffner SM, Lehto S, Rönnemaa T, Pyörälä K, Laakso M. Mortality from coronary heart disease in subjects with type 2 diabetes and in nondiabetic subjects with and without prior myocardial infarction. N Engl J Med. 1998. 339:229–234.
2. Hink U, Li H, Mollnau H, et al. Mechanisms underlying endothelial dysfunction in diabetes mellitus. Circ Res. 2001. 88:E14–E22.
3. De Vriese AS, Verbeuren TJ, Van de Voorde J, Lameire NH, Vanhoutte PM. Endothelial dysfunction in diabetes. Br J Pharmacol. 2000. 130:963–974.
4. Johnstone MT, Creager SJ, Scales KM, Cusco JA, Lee BK, Creager MA. Impaired endothelium-dependent vasodilation in patients with insulin-dependent diabetes mellitus. Circulation. 1993. 88:2510–2516.
5. Balletshofer BM, Rittig K, Enderle MD, et al. Endothelial dysfunction is detectable in young normotensive first-degree relatives of subjects with type 2 diabetes in association with insulin resistance. Circulation. 2000. 101:1780–1784.
6. Hattori Y, Kawasaki H, Abe K, Kanno M. Superoxide dismutase recovers altered endothelium-dependent relaxation in diabetic rat aorta. Am J Physiol. 1991. 261(4 Pt 2):H1086–H1094.
7. Beckman JA, Goldfine AB, Gordon MB, Garrett LA, Creager MA. Inhibition of protein kinase Cbeta prevents impaired endothelium-dependent vasodilation caused by hyperglycemia in humans. Circ Res. 2002. 90:107–111.
8. Steinberg HO, Chaker H, Leaming R, Johnson A, Brechtel G, Baron AD. Obesity/insulin resistance is associated with endothelial dysfunction: implications for the syndrome of insulin resistance. J Clin Invest. 1996. 97:2601–2610.
9. Atkins GB, Jain MK. Role of Krüppel-like transcription factors in endothelial biology. Circ Res. 2007. 100:1686–1695.
10. Samatar AA, Wang L, Mirza A, Koseoglu S, Liu S, Kumar CC. Transforming growth factor-beta 2 is a transcriptional target for Akt/protein kinase B via forkhead transcription factor. J Biol Chem. 2002. 277:28118–28126.
11. Buteau J, Accili D. Regulation of pancreatic beta-cell function by the forkhead protein FoxO1. Diabetes Obes Metab. 2007. 9:Suppl 2. 140–146.
12. Kamagate A, Qu S, Perdomo G, et al. FoxO1 mediates insulin-dependent regulation of hepatic VLDL production in mice. J Clin Invest. 2008. 118:2347–2364.
13. Kim HS, Skurk C, Thomas SR, et al. Regulation of angiogenesis by glycogen synthase kinase-3beta. J Biol Chem. 2002. 277:41888–41896.
14. Kanemoto N, Hishigaki H, Miyakita A, et al. Genetic dissection of "OLETF", a rat model for non-insulin-dependent diabetes mellitus. Mamm Genome. 1998. 9:419–425.
15. Kumar A, Lindner V. Remodeling with neointima formation in the mouse carotid artery after cessation of blood flow. Arterioscler Thromb Vasc Biol. 1997. 17:2238–2244.
16. Korshunov VA, Berk BC. Flow-induced vascular remodeling in the mouse: a model for carotid intima-media thickening. Arterioscler Thromb Vasc Biol. 2003. 23:2185–2191.
17. Wang N, Miao H, Li YS, et al. Shear stress regulation of Krüppel-like factor 2 expression is flow pattern-specific. Biochem Biophys Res Commun. 2006. 341:1244–1251.
18. Parmar KM, Larman HB, Dai G, et al. Integration of flow-dependent endothelial phenotypes by Kruppel-like factor 2. J Clin Invest. 2006. 116:49–58.
19. Hurks R, Eisinger MJ, Goovaerts I, et al. Early endothelial dysfunction in young type 1 diabetics. Eur J Vasc Endovasc Surg. 2009. 37:611–615.
20. Baris N, Akdeniz B, Uyar S, et al. Are complex coronary lesions more frequent in patients with diabetes mellitus? Can J Cardiol. 2006. 22:935–937.
21. Iakovou I, Schmidt T, Bonizzoni E, et al. Incidence, predictors, and outcome of thrombosis after successful implantation of drug-eluting stents. JAMA. 2005. 293:2126–2130.
22. Parmar KM, Nambudiri V, Dai G, Larman HB, Gimbrone MA Jr, García-Cardeña G. Statins exert endothelial atheroprotective effects via the KLF2 transcription factor. J Biol Chem. 2005. 280:26714–26719.
23. Rossi J, Rouleau L, Tardif JC, Leask RL. Effect of simvastatin on Kruppel-like factor2, endothelial nitric oxide synthase and thrombomodulin expression in endothelial cells under shear stress. Life Sci. 2010. 87:92–99.
24. Arslan F, Pasterkamp G, de Kleijn DP. Unraveling pleiotropic effects of statins: bit by bit, a slow case with perspective. Circ Res. 2008. 103:334–336.
25. Thum T, Bauersachs J. Sports or statins for atheroprotection? New insight from Kruppel-like factor 2. Cardiovasc Res. 2006. 72:193–195.