Abstract
Background and Objectives
Vasospastic angina (VA) is a specific type of coronary artery disease and develops as a result of coronary artery spasm. Recently, a few studies have revealed that VA caused by coronary artery spasm is related to genetic traits. The objective of this study was to use the recently developed technique of array comparative genomic hybridization (CGH) to screen the genetic aberrations of VA.
Subjects and Methods
To identify candidate genes that might be causally involved in the pathogenesis of VA, genomic deoxyribonucleic acids were extracted from whole blood of 28 patients with VA who presented at Department of Cardiology at Seoul St. Mary's Hospital, Seoul, Korea. The copy number profiles of these patients was then analyzed using array CGH and reverse transcriptase (RT) quantitative polymerase chain reaction (PCR).
Results
Array CGH revealed gains in 31 different regions, with losses in the 4q35.2, 7q22.1, 10q26.3, 15q11.2, 16p13.11, 17p11.2 and 19q13.3 regions (more than 32% aberration in these regions). Several loci were found to be frequently including gains of 5p and 11q (50% of samples). The most common losses were found in 7q (54% of samples). Copy number aberrations in chromosomal regions were detected and corresponding genes were confirmed by RT quantitative PCR. The fold change levels were highest in the CTDP1 (18q23), HDAC10 (22q13.33), KCNQ1 (11p15.5-p15.4), NINJ2 (12p13.33), NOTCH2 (1p12-p11.2), PCSK6 (15q26.3), SDHA (5p15.33), and MUC17 (7q22.1) genes.
Vasospastic angina (VA), also called Prinzmetal's angina or variant angina, is a specific type of coronary artery disease and is developed due to not atherosclerosis but coronary artery spasm. This disease can cause acute coronary syndrome, including acute myocardial infarction, ventricular tachycardia, fibrillation or electro mechanical dissociation, and even sudden cardiac death.1) The incidence of VA is higher in Asians than Caucasians or Blacks,2)3) which strongly suggests that genetic traits might be involved in the pathogenesis of VA.4-11)
Studies have shown that the loss of Kir6.1 {poreforming inwardly rectifying K+ (Kir) channel subunit} or SUR2 (sulfo-nylurea receptor regulatory subunit of KATP channel) by related gene deletions in mice induced VA.4)5) So far, only these two genes have been shown to be associated with gene copy number alterations. Other investigations showed gene polymorphisms6-8) and functional expression9-11) in VA. Related gene polymorphisms in VA included an endothelial nitric oxide synthase gene,6) adrenergic receptor gene,7) and the paraoxonase 1 gene.8) The Rho-associated kinase gene9) and serotonin receptor (5-HT1Dβ) gene10)11) were also shown to be related to VA by their functional expression. To date, there have been no studies on deoxyribonucleic acid (DNA) copy number alterations in the whole human genome among patients with VA. The recent development of array-comparative genomic hybridization (CGH) provides a means to quantitatively measure DNA copy number aberrations and to map them directly to genomic sequences.12-14) Also, tiling resolution DNA microarrays comprised of large-insert genomic clones such as bacterial artificial chromosomes (BACs) are a very useful and accurate method for detecting genomic aberrations in many genetic diseases.15)16) Furthermore, array-CGH with BAC clones, has been proven to be a very useful and accurate tool for detecting genomic aberrations in whole blood samples for schizophrenia, epilepsy and mental retardation,17-19) as well as in tissue from some cancers.20-24) Both fluorescence in situ hybridization (FISH) and real-time polymerase chain reaction (RT-PCR) have also been applied empirically to confirm array-CGH results.17)18)21-24)
In the study presented here, we analyzed genomic DNA copy number aberrations in whole blood from 28 patients with VA using array CGH. Genetic aberrations, for a subset of gained or lost gene, were confirmed by RT-PCR.
Peripheral blood samples were collected from 28 patients with VA at the Department of Cardiology at Seoul St. Mary's Hospital, Seoul, Korea. In this study, the diagnosis of VA was made when patients met all of the following criteria: 1) repetitive burning or squeezing retrosternal chest pain, 2) definite positive response by coronary angiography with intra coronary acetylcholine provocation test, 3) no significant atherosclerotic stenosis in coronary artery (<50% narrowing of the coronary luminal diameter) according to quantitative coronary angiography. Blood samples were collected from 8 women and 20 men, aged 34-71 years (mean age±standard deviation, 56.44±11.4 in years). The clinical characteristics of the patients are shown in Table 1. Genomic DNA was extracted using a Puregene DNA isolation kit (Qiagen, Hilden, Germany). Reference DNA was pooled from 10 gender-matched (male), normal, healthy control subjects. All patients gave written informed consent to participate in this study. The protocol of this study was approved by the institutional review board of Seoul St. Mary's Hospital, The Catholic University of Korea.
Array CGH analyses were conducted on 28 individual samples using commercial MACArray™ Karyo 4K BAC-chips (Macrogen, Seoul, Korea) with 4,030 BAC clones, in duplicate, on the whole human genome with a resolution of about 1 Mbp. Array CGH was performed as described previously.25) Briefly, 500-700 ng target and reference DNAs were denatured in the presence of random primer and reaction buffer (BioPrime® DNA Labeling System, Invitrogen, Carlsbad, CA, USA) at 98-100℃ for 5 minutes, and then cooled on ice for 5 minutes. The denatured DNA was differentially labeled with 3 µL of 1 mM Cy3- and Cy5-conjugated dCTP by random primed labeling (Perkin Elmer, Waltham, MA, USA). The mixture was incubated with a Klenow fragment at 37℃ overnight. After labeling, unincorporated nucleotides were removed using MicroSpin™ G-50 columns (Amersham Biosciences, Buckinghamshire, UK). Cy3- and Cy5-labeled test DNA and reference DNA were mixed with 50 µL of human Cot-1 DNA for blocking of repeat sequences. After purification, this mixture was resolved in hybridization buffer (Macrogen) containing yeast tRNA for blocking of non-specific nucleotides binding.
After the MACArray™-Karyo 4K BAC-chip was prehybridized in hybridization buffer with salmon sperm DNA for 1 hour, chips were hybridized with the purification mixture. It was then incubated for 72 hours in the 37℃ hybridization chamber (BioMicro systems, Salt Lake City, UT, USA). After hybridization was complete, array chips were washed in 50% formamide-2x SSC at 46℃ for 15 minutes, and 0.1% SDS-2x SSC at 46℃ for 30 minutes. Next, the chips were washed in 50% sodium phosphate-0.1% NP40 for 15 minutes followed by washing with 2x SSC for 15 minutes at room temperature. After spin drying, hybridized arrays were scanned with a Genepix™ (Axon Instruments, Sunnyvale, CA, USA).
Raw signal intensities from the arrays were measured using the MAC Viewer v1.6.3 software (Macrogen). Log2 (Cy3 intensity/Cy5 intensity ratios) were normalized by using the median of fluorescence ratios computed from the housekeeping DNA control fragments linearly distributed across the genome. Measurements flagged as unreliable by MAC Viewer v1.6.3 were removed from subsequent analysis. The threshold corresponds to 2 standard deviation (SD) values from the mean. The information on each individual clone was obtained from the UCSC Genome Bioinformatics database (May 2004 freeze, http://genome.ucsc.edu).
To confirm the level of genomic imbalances identified by array CGH in this study, DNA samples with obvious genomic changes were analyzed using RT quantitative PCR.26) The reaction was performed in a total volume of 50 µL, including 25 µL of 2x IQ™ SYBR® green supermix (Bio-Rad, Hercules, CA, USA), 10 ng of DNA, and 10 pmol of each primer.27) Forty cycles of amplification, data acquisition, and data analysis were performed in an iCycler (Bio-Rad). Primers for eight genes (CTDP1, HDAC10, KCNQ1, NINJ2, NOTCH2, PCSK6, SDHA, and MUC17) were selected and the position of each clone was obtained from the UCSC genome database (Table 2). The relative genomic copy number was calculated using the comparative CT Method.28) GAPDH was used as an endogenous reference.28-30)
Chromosomal copy number changes were detected by array-CGH in VA. Copy number changes were presented as individual chromosome plots of log2 ratio of normalized Cy3 : Cy5 intensities versus chromosome regions for each of the individual 28 cases of VA. Table 3 shows the frequency of aberration, ranging from 7 (25% of chromosomal gain and loss) to 15 (54% of chromosomal loss at 7q) in 28 samples. Overall, the individual chromosomal aberration pattern was not random and tended to be consistent, showing DNA gains in 1p, 1q, 2p, 2q, 3p, 3q, 4p, 4q, 5p, 5q, 6p, 6q, 7q, 8p, 8q, 9q, 10p, 10q, 11p, 11q, 12q, 13q, 14q, 15q, 16p, 16q, 17p, 17q, 18q, 20p, 20q, 21q, and 22q; and showing DNA losses in 2p, 4q, 7q, 10q, 15q, 16p, 17p, and 19p. DNA gains, rather than DNA losses, were more frequently observed. The most frequent gain detected was at 5p15.33, and 11q12.2 (50%). Frequent DNA gains also were observed in 12p13.33, 14q32.33, and 15q11.2 in 13 samples (46%), and in 12p13.33 (43%), 1p12-p11.2, 4p15.1, 8q22.2, 11q25, and 15q26.3 in 11 samples (39%). Frequent gains (36%) were located at: 1q44, 3q24, 11q23.3, 15q11.2, 15q25.2, 15q26.3, 16p12.1, 16p13.2, 17p12, 18q12.3, and 21q11.2. Other regions with 25-32% frequency of DNA gains included 1p12-p11.2, 1p12, 1p21.1, 2p11.2, 2q21.2-q21.3, 2q22.1, 2q23.3, 2q31.1, 2q37.3, 3p14.1, 3q12.3, 4p15.1, 4p15.2, 4q24, 4q32.3, 4q34.3, 4q35.2, 5p12, 5p15.2, 5p15.33, 5q15, 6p12.2, 6q24.1, 7q11.23, 7q22.2, 8p23.3, 8q24.3, 9q22.1, 9q22.32, 9q33.3, 10p15.3, 10q11.23, 10q23.1, 10q26.3, 11p15.5, 11p15.5-p15.4, 11q14.1, 11q23.1, 11q23.2, 12p13.33, 12q22, 13q33.3, 14q31.3, 15q26.3, 16p11.2, 16q24.1, 17q24.3, 17q25.3, 18q23, 20p12.2, 20q12, 20q13.33, 21q11.2, 21q22.3, 22q12.2, and 22q13.33. The most common genomic losses were observed in 7q22.1 (54%), 4q35.2 (43%), and 15q11.2 (39%), 10q26.3 (36%). Other regions with 25-32% frequency of DNA losses included 2p24.1, 7q36.3, 10q26.3, 16p13.11, 16p13.3, 17p11.2, and 19p13.3 (Table 3). Fig. 1 shows chromosomal aberration regions. 1p12-p11.2, 5p15.33, 11p15.5-p15.4, 12p13.33, 15q26.3, 18q23, and 22q13.33 contained CTDP1, HDAC10, KCNQ1, NINJ2, NOTCH2, PCSK6, and SDHA genes (gained regions), while loss region 7q22.1 contained MUC17 gene.
To confirm the array CGH results, differential fold change was evaluated by RT quantitative PCR in VA samples. The results showed chromosomal fold changes in CTDP1 (18q23), HDAC10 (22q13.33), KCNQ1 (11p15.5-p15.4), NINJ2 (12p 13.33), NOTCH2 (1p12-p11.2), PCSK6 (15q26.3), SDHA (5p15.33), and MUC17 (7q22.1). Fig. 2 shows the RT PCR results. In gained regions of 7 genes, {CTDP1, HDAC10, KCNQ1, NINJ2, NOTCH2, PCSK6, and SDHA (A-G)}, the relative fold increases in RT quantitative PCR corresponded with array-CGH results. In MUC17 (H), the decrease in fold differences noted by RT quantitative PCR was similar to that seen with array-CGH. The N-value was delineated in RT PCR. The results compared linear-ratios in array-CGH, were demonstrated generally larger by RT quantitative PCR analysis than those by array-CGH analysis.
Several methods including classical cytogenetics, FISH, Southern blot analysis, metaphase CGH, and array CGH have been used to detect DNA copy number changes.23) Lately, array CGH has been shown to be a powerful tool for detecting genetic aberrations and genetic mapping, and offers high resolution, high-throughput, accuracy, and sensitivity for genetic analysis.12-14) It also allows the simultaneous quantitative analysis of all regions of a large genome,25) and can provide quantitative information at the level of chromosomal gain or loss.21)
Although the confirmation of array-CGH results is usually performed by FISH analysis, this technique has several limitations. For example, evaluation of the full extent of genetic gains or losses in the genome requires obtaining metaphase DNA and interpretion of karyotypes that are often complex and require prior knowledge or markers of sites of interest.2) Many recent studies have shown that RT quantitative PCR instead of FISH is also a very useful and accurate technique for the confirmation of array-CGH results,17)18)21-24) thus making it particularly attractive technique for the identification of acquired genetic aberrations in whole blood from patients with VA. Therefore, we used RT quantitative PCR for the confirmation and quantification of the identified genomic aberrations.
In this study, using array CGH, we have showed than there are genetic aberrations and altered genes related to VA. The DNA aberrations had a tendency to be located at rather specific chromosomal regions. Ulike amplification of oncogenes or deletions of tumor suppressor genes21-24) there were no specific genes amplified or deleted in VA in our study. However, many DNA copy number aberrations (91 DNA gains and 12 DNA losses) were detected. These DNA copy number aberrations were novel and different from those previously detected in the Korean population (DNA gains in 6p21.2 and DNA loses in 1p36.31, 4q13.1-4q22.13, 16p12.1, 21q22.3, and 22q11.22).31) This showed that many genetic alterations might be associated with the pathogenesis of VA.
Because array CGH shows genetic aberrations at the genomic DNA level and not at the messenger RNA or protein levels, many chromosomal regions which might be related to the pathogenesis of VA were found in this study. For example, the KCNQ1 gene, one of the genes analyzed by RT quantitative PCR,27) is translated into the potassium voltage-gated channel subfamily KQT member1, which is probably related to cardiac repolarization (www.ihop-net.org/UniPub/iHOP). Also, the NOTCH2 gene, another one of the genes analyzed by RT quantitative PCR, is translated into neurogenic locus notch homolog protein 2 precursors; notch homolog protein 2 is a receptor for the membrane-bound ligands involved in regulation of cell-fate determination (www.ihop-net.org/UniPub/iHOP). However, the function of the proteins translated by these genes has not been completely clarified, so definite pathogenesis of VA cannot be established based on our results. Nevertheless, our data may become the cornerstone that will establish the function of each genetic aberration involved in the pathogenesis of VA.
In summary, using array CGH, we analyzed genome-wide chromosomal aberrations and screened chromosomal candidate regions related to the pathogenesis of VA. The array-CGH results were confirmed by RT quantitative PCR. This study is the first for searching whole genomic alterations by array CGH in patients with VA. Our study may contribute to further clarification of important chromosomal regions, identification of candidate genes, and understanding of the pathogenesis of VA.
Figures and Tables
Fig. 1
Array comparative genomic hybridization (CGH) results. Average log2 ratios (Y axis) of each chromosomal region at 1p, 5p, 7q, 11p, 12p, 15q, 18q and 22q (X axis) show differences in copy number in spasm patients. Internal control (XX/XY) was used to detect genomic imbalances. Note the high-level aberrations (arrowed spots) on chromosomes.
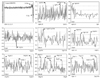
Fig. 2
Relative fold differences selected from 8 genes showed that the most frequent gains and losses detected were at 1p, 5p, 7q, 11p, 12p, 15q, 18q and 22q region. Each sample is depicted (X axis) and the fold difference of N-value was delineated in RT PCR (Y axis). A threshold level of 2 (N-value) indicates significant DNA gain (A-G), and 0.5 (N-value) indicates significant DNA loss (H). RT: reverse transcriptase, PCR: polymerase chain reaction, DNA: deoxyribonucleic acid.
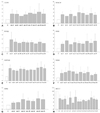
Acknowledgments
This work was supported by a grant (00-PJ3-PG6-GN02-0002) from the Korean Health 21 R&D Project, the Ministry of Health and Welfare, Republic of Korea.
References
1. Nakamura M, Takeshita A, Nose Y. Clinical characteristics associated with myocardial infarction, arrhythmias, and sudden death in patients with vasospastic angina. Circulation. 1987. 75:1110–1116.
2. Pristipino YC, Beltrame JF, Finochiaro ML, et al. Major racial differences in coronary constrictor response between Japanese and Caucasians with recent myocardial infarction. Circulation. 2000. 101:1102–1108.
3. Bertrand ME, LaBlanche JM, Tilmant PY, et al. Frequency of provoked coronary arterial spasm in 1089 consecutive patients undergoing coronary arteriography. Circulation. 1982. 65:1299–1306.
4. Hibino H, Kurachi Y. A new insight into the pathogenesis of coronary vasospasm. Circ Res. 2006. 98:579–581.
5. Chutkow WA, Pu J, Wheeler MT, et al. Episodic coronary artery vasospasm and hypertension develop in the absence of Sur2KATP channels. J Clin Invest. 2002. 110:203–208.
6. Chang K, Baek SH, Seung KB, et al. The Glu298Asp polymorphism in the endothelial nitric oxide synthase gene is strongly associated with coronary spasm. Coron Artery Dis. 2003. 14:293–299.
7. Park JS, Zhang SY, Jo SH, et al. Common adrenergic receptor polymorphisms as novel risk factors for vasospastic angina. Am Heart J. 2006. 151:864–869.
8. Cho YS, Choi JH, Zhang SY, et al. Relationship of polymorphisms in the oxidative stress related genes: paraoxonase and p22phox - to variant angina and coronary artery stenosis in Korean. Korean Circ J. 2003. 33:104–112.
9. Shimokawa H, Seto M, Katsumata N, et al. Rho-kinase-mediated pathway induces enhanced myosin light chain phosphorylations in a swine model of coronary artery spasm. Cardiovasc Res. 1999. 43:1029–1039.
10. Ishida T, Hirata K, Sakoda T, et al. 5-HT1Dβ receptor mediates the supersensitivity of isolated coronary artery to serotonin in variant angina. Chest. 1998. 113:243–244.
11. Shimizu M, Hata K, Takaoka H, et al. Sumatriptan provokes coronary artery spasm in patients with variant angina: possible involvement of serotonin 1B receptor. Int J Cardiol. 2007. 114:188–194.
12. Pinkel D, Segraves R, Sudar D, et al. High resolution analysis of DNA copy number variation using comparative genomic hybridization to microarrays. Nat Genet. 1998. 20:207–211.
13. Mantripragada KK, Buckley PG, Diaz de Ståhl T, Dumanski JP. Genomic microarrays in the spotlight. Trends Genet. 2004. 20:87–94.
14. Pinkel D, Albertson DG. Comparative genomic hybridization. Annu Rev Genomics Hum Genet. 2005. 6:331–354.
15. Snijders AM, Nowak N, Segraves R, et al. Assembly of microarrays for genome-wide measurement of DNA copy number. Nat Genet. 2001. 29:263–264.
16. Ishkanian AS, Malloff CA, Watson SK, et al. A tiling resolution DNA microarray with complete coverage of the human genome. Nat Genet. 2004. 36:299–303.
17. Moon HJ, Yim SV, Lee KW, et al. Identification of DNA copy-number aberrations by array-comparative genomic hybridization in patients with schizophrenia. Biochem Biophys Res Commun. 2006. 344:531–539.
18. Kim HS, Yim SV, Jung KH, et al. Altered DNA copy number in patients with different seizure disorder type: by array-CGH. Brain Dev. 2007. 29:639–643.
19. Bonaglia MC, Giorda R, Tenconi R, et al. A 2.3Mb duplication of chromosome 8q24.3 associated with severe mental retardation and epilepsy detected by standard karyotype. Eur J Hum Genet. 2005. 13:586–591.
20. Pinkel D, Albertson DG. Array comparative genomic hybridization and its applications in cancer. Nat Genet. 2005. 37:S11–S17.
21. Choi YW, Choi JS, Zheng LT, et al. Comparative genomic hybridization array analysis and real time PCR reveals genomic alterations in squamous cell carcinomas of the Lung. Lung Cancer. 2007. 55:43–51.
22. Choi JS, Zheng LT, Ha E, et al. Comparative genomic hybridization array analysis and real-time PCR reveals genomic copy number alteration for lung adenocarcinomas. Lung. 2006. 184:355–362.
23. Hurst CD, Fiegler H, Carr P, Williams S, Carter NP, Knowles MA. High-resolution analysis of genomic copy number alterations in bladder cancer by microarray-based comparative genomic hybridization. Oncogene. 2004. 23:2250–2263.
24. Mao X, Orchard G, Lillington DM, Russell-Jones R, Young BD, Whittaker S. Genetic alterations in primary cutaneous CD30+ anaplastic large cell lymphoma. Genes Chromosomes Cancer. 2003. 37:176–185.
25. Guillaud-Bataille M, Valent A, Soularue P, et al. Detecting single DNA copy number variations in complex genomes using one nanogram of starting DNA and BAC-array CGH. Nucleic Acids Res. 2004. 32:e112.
26. Li Y, Zhang R, Qiao H, et al. Generation of insulin-producing cells from PDX1 gene-modified human esenchymal stem cells. J Cell Physiol. 2007. 211:36–44.
27. Kang SM, Chang W, Lim S, et al. Losartan inhibits proliferation and inflammation of vascular smooth muscle cells by modulation of uric acid transporter. Tissue Eng Regen Med. 2008. 5:521–527.
28. Wang G, Brennan C, Rook M, et al. Balanced-PCR amplification allows unbiased identification of genomic copy changes in minute cell and tissue samples. Nucleic Acids Res. 2004. 32:e76.
29. Calvo R, West J, Franklin W, et al. Altered HOX and WNT7A expression in human lung cancer. Proc Natl Acad Sci U S A. 2000. 97:12776–12781.
30. Chujo M, Noguchi T, Miura T, Arinaga M, Uchida Y, Tagawa Y. Comparative genomic hybridization analysis detected frequent overrepresentation of chromosome 3q in squamous cell carcinoma of the lung. Lung Cancer. 2002. 38:23–29.
31. Jeon JP, Shim SM, Jung JS, et al. A comprehensive profile of DNA copy number variations in a Korean population: identification of copy number invariant regions among Koreans. Exp Mol Med. 2009. 41:618–628.