Abstract
Background and Objectives
Hyperacute rejection (HAR) is a major obstacle to successful xenotransplantation of vascularized organs. This study was conducted to observe the effect of hemolysis of perfused human whole blood on pig heart function, and determine the major risk factors for preservation of xenoperfused cardiac function using ex-vivo pig to human xenogeneic cardiac perfusion model.
Materials and Methods
Harvested pig hearts were perfused with normal human whole blood (group 1), two different types of pre-treated human whole blood (group 2: immunoglobulins were depleted by plasmapheresis, group 3: pre-treated with plasmapheresis, GAS914, cobra venom factor (CVF) and steroid), and normal porcine whole blood as control (group 4) for 3 hours.
Results
Duration of heart beat was significantly prolonged in group 2 and group 3. Histological examination showed widespread HAR features but was gradually delayed in groups 2 and 3 compared to group 1. The absolute levels of serum creatine kinase-MB and Troponin I increased gradually, and was lower in group 3. Serum hemoglobin levels were rapidly increased in groups 3 and 4, compared to group 1. Extracellular potassium level increased sharply from the beginning of blood perfusion in groups 1, 2 and 3, compared to group 4.
Conclusion
Pretreatment of human whole blood, including immunoglobulin depletion, CVF and steroid reduced and delayed the destruction of pig myocardium by HAR. However, the increased extracellular potassium levels in groups 1, 2 and 3 reflected that these treatments could not prohibit myocardial injury by HAR.
Organ transplantation is the most effective and unique method of support for end-stage organ failure patients. However, transplantation is currently limited by shortage of human donors. This drawback may be eliminated by xenotransplantation using porcine organs, but there are several barriers to the transplantation of porcine organs into humans.1-4) Hyperacute rejection (HAR) is the most serious complication when porcine organ is transplanted to human. When xenoantigens that exist in porcine organs bind and react with natural antibodies in human blood, the human complement system will in turn be activated, ultimately lead to the transplanted porcine organ being destroyed within minutes or hours.5-7) Therefore, efforts to prevent HAR have concentrated on removing xenoreactive natural antibodies, and inhibiting complement activation.5-8) Ex vivo perfusion model has been used to evaluate HAR in pig to human combination, because this allows continuous monitoring of physiologic parameters as well as sequential sampling of tissue and blood. Results obtained from our ex vivo perfusion model may help verify the efficacy of treatments and provide a framework with which to support in vivo experiments later.
Risk factors have been addressed frequently in many xenogeneic perfusion models, such as kidney, lung and liver,9-11) especially they have been reported to impose the most significant risks on xenograft function secondary to hemolysis, because severe hemolysis causes intravascular thrombi comprised of fibrin and platelet, rapid reduction of coagulation factors, and results in disseminated intravascular coagulation.9) However, there exist few established reports that associate with hemolysis in the cardiac xenotransplantation and heart is not similar to liver, kidney or lung. We investigated whether hemolysis may be the major risk factor of xenoperfused cardiac function or not. Hyperkalemia that could be induced by hemolysis of xenoperfused blood and myocardial cell injury by HAR would fail the transplanted heart function. We analyzed extracellular potassium levels, and analyzed its role in xenoperfused cardiac function.
In this study, we used an ex-vivo xenogeneic cardiac perfusion model with porcine hearts and fresh human whole blood, and then evaluated the protective effects of pre-treatments, such as plasmapheresis, GAS914, cobra venom factor (CVF) and steroid. Plasmapheresis has been used to remove anti-porcine antibodies, especially IgM and IgG from human serum. As such, it reduces HAR secondary to natural antibodies and xenoantigens induction.1)5)8)12) GAS914 is a soluble, polymeric form of a Gal α-1,3-Gal trisaccharide. Intravenous infusion of GAS914 can successfully reduce preformed antibodies in the recipient.13-15) CVF acts as a homolog of C3 in vivo,16) and administration to recipient before transplantation can suppress graft rejection by depleting the complement system in advance.17-19) Several studies concluded that CVF administration before xenotransplantation prolonged graft survival.20)21) To ascertain the effects of the aforementioned on xenoperfused porcine myocardium, we evaluated basic laboratory outcomes, such as complete blood cell counts (CBC), arterial blood gas analysis (ABGA), and evaluated myocardial cell injury by measuring creatine kinase-MB (CK-MB) and Troponin I. In addition, we observed the histological changes of xenoperfused myocardium, and the effects of perfused blood hemolysis and hyperkalemia on porcine cardiac function.
Outbred piglets, weighing approximately 5 kg, were used as cardiac donors. Piglets were chosen because they can be easily handled and their hearts are lighter than that of adult pig. All animal experiments were performed after receiving the approval of the Institutional Animal Care and Use Committee of the Clinical Research Institute at Seoul National University Hospital. National Research Council 'Guidelines for the Care and Use of Laboratory Animals' were observed (revised 1996). Piglet hearts were harvested as described previously.1) Briefly, the superior vena cava (SVC), inferior vena cava (IVC), aorta, right innominate artery (RIA), pulmonary artery (PA), pulmonary veins, and left azygous vein were isolated, and heparin (200 unit/kg of body weight) was administered through the right atrium. The proximal RIA was cannulated with a 12-gauge catheter to infuse heart preservation solution and to connect the perfusion system for later use. The SVC, distal RIA and left azygous vein were ligated and the IVC was transected. The aortic arch was cross-clamped posterior to the RIA by ligation with a thick string, and 20 mL/kg of body weight of University of Wisconsin solution was infused into the aortic root through the catheter. Immediate heart arrest was obtained and the heart was then excised. Extracted piglet hearts weigh about 30 g. Subsequently, the heart was connected to the ex vivo xenoperfusion circuit (Fig. 1). The ischemic period was less than 10 minutes.
Fresh human whole blood for perfusion was obtained from normal healthy male volunteers within 24 hours of the experiment without pre-treatment. Use of human subjects as blood donors was certified by the Seoul National University College of Medicine and the Seoul National University Hospital Institutional Review Board. Each blood unit (about 350 mL) from one volunteer was used for a separate experiment after dilution with Hartmann solution. Three test groups (group 1-3) and one control group (group 4) were formed according to the preparation of perfused blood. Group 1 received normal fresh human whole blood (n=4); group 2 received human whole blood pre-treated with plasmapheresis to remove natural antibodies just before experiment (n=3); group 3 received human whole blood treated with plasmapheresis, GAS914 (Novartis Pharma Ag, 1 mg/kg), CVF (0.5 mg/kg), and steroid (Solumedrol 24 mg/kg, n=2); and group 4 received normal fresh porcine whole blood (n=2). We applied identical methods and parameters to all four groups. Plasmapheresis was performed using COBE plasma filtration device (COBE Spectra, Lakewood, USA) and Evalflux 2A membrane (Kawasumi laboratories, Tokyo, Japan) in accordance with the manufacturer's instruction.
Extracted hearts were connected to non-working isolated heart perfusion circuit with membranous oxygenator (Minimax, Medtronic, USA), and then blood was perfused to porcine coronary artery through the innominate artery using roller pump (Cobe Heart-Lung machine, Cobe, USA). The mean aortic perfusion pressure was fixed at 80 mmHg. Each human blood unit was diluted with Hartmann solution before perfusion to maintain hemoglobin (Hb) level at 5-6 mg/mL, hematocrit at 15-20%. This level of Hb is sufficient to maintain the oxygen carrying capacity and low viscosity for artificial circuit circulation. The total volume of diluted blood was about 800 mL. The oxygen tension pressure was maintained above 150 torr. In this non-working isolated heart perfusion circuit, human blood flowed through the innominate artery, coronary arteries, right atrium, right ventricle and the main PA of porcine heart before re-entering the circuit again.
From the beginning of blood perfusion through the porcine heart, we recorded the duration of heart beat until myocardial contraction ceased. We collected tiny pieces of myocardium (about 5×5×5 mm) at the apex of the left ventricle 10, 60, 120 and 180 minutes after reperfusion, and rapidly fixed them in 4% paraformaldehyde. Histological changes were assessed after routine hematoxylin and eosin staining of paraffin-embedded sections. In addition, blood samples were obtained at defined intervals for the measurement of CBC and serum chemistry. They were collected before cardiac perfusion, 5 minutes intervals until 30 minutes after perfusion, every 15 minutes until 120 minutes, and then every 30 minutes until 180 minutes. All blood samples were examined for CBC (XE-2100D, Syxmex co. Japan), ABGA (ABL 520, Diamond Diagnostic, USA), cardiac enzyme (AXSYM, Abbott Lab, USA), serum hemoglobin (sHb, Milton Roy Spectronic 601, Pharmacia, USA), electrolyte (Toshiba-200FR, Toshiba, Japan), and antibody titers (Olympus AU5421, Olympus Amerlca Inc., USA).
All results are expressed as mean and standard deviation. We used Statistical Package for the Social Sciences (version 10.0) statistics program for data analysis. For data comparison, we used the analysis of variance (ANOVA) test to compare heart beating time. We also used the repeated measurement analysis method (Repeated measures ANOVA) for changes in several variables of the sampled blood with time, and then used the Tukey test as post hoc multiple comparisons. A p<0.05 was considered to be a statistically significant difference.
The total elimination rates of immunoglobulin by plasmapheresis were 65.3% (IgM) and 77.4% (IgG) in group 2. Although we were not aware of the precise exclusion rate of anti-Gal specific antibodies by GAS914, the elimination rates of immunoglobulin in group 3 were increased to 83.7% (IgM) and 84.8% (IgG) respectively.
Isolated piglet hearts started beating within 1 minute after perfusion in all groups. Compared to group 1, the hearts in groups 2 and 3 beat significantly longer (p=0.014). The durations of heart beating were 4.8±3.2 minutes (group 1), 18.0±5.0 minutes (group 2), 15.0±5.0 minutes (group 3) and over 180 minutes (group 4) respectively. However, there was no significant difference between groups 2 and 3 (p>0.05).
Histological examination of porcine myocardium showed typical features of HAR, characterized by marked hemorrhage, congestion, interstitial edema, platelet aggregation, thrombi, capillary obstruction and myocardial cell destruction. The changes in group 1 began within 10 minutes after perfusion, and the myocytes were almost morphologically destroyed within 60 minutes (Fig. 2). In groups 2 and 3, myocyte destruction developed as in group 1, but these destructive changes were delayed and weaker compared to group 1. Particularly in group 3, destructive changes were delayed more than the group 1 and 2, although the appearance of congestion, changes of the myocyte shape were increased as perfusion time elapsed (Figs. 2 and 3). In group 4, the shape of the myocyte was maintained relatively well, but hemorrhage increased gradually, similar to that in group 3. We observed that changes in myocytes in group 3 were similar to that observed in group 4 (Fig. 3).
Among CBC results, leukocyte count was reduced at the beginning of perfusion in all groups. There was no difference in hemoglobin, hematocrit levels in the xenograft groups (group 1, 2 and 3). Platelets, which form thrombus in organ rejection, was decreased at the beginning of perfusion, particularly more pronounced in group 1 (from 46.5±37.4 /L at the beginning of the perfusion to 9.5±4.9/L at 15 minutes later) among the xenograft groups, but there was no statistical significance (data not shown).
Troponin I levels, which reflect myocardial destruction most distinctively, were gradually increased soon after perfusion commencement in all groups, particularly more pronounced in group 1 (Fig. 4A), but there was no statistical significance among the groups (p=0.25). CK-MB levels were gradually increased in all groups, including group 4, and early increase was also drastic in group 1, but not statistically significant (Fig. 4B). However, we found the tendency of increase in cardiac enzymes to be the least in group 3.
We measured serum Hb levels to investigate the degree of hemolysis of perfused blood in each group. As shown in Fig. 5A, serum Hb in group 1 demonstrated the lowest levels, and plasmapheresis, GAS914, CVF, and steroid (group 3) accelerated hemolysis more than group 1 (p=0.018). Of interest, the serum Hb level in group 4 was markedly increased 30 minutes after perfusion without affecting heart beat (Fig. 5A).
Potassium levels that reflect myocardial cell injury and perfused blood cells were increased sharply at the beginning of perfusion in all xenograft groups (group 1, 2 and 3), but there was no difference between these xenograft groups. The potassium level of group 4 was increased only mildly at the end of reperfusion, the degree of increase was significantly different from the other xenograft groups (p=0.043) (Fig. 5B).
We measured the levels of cardiac enzymes, including CK-MB and Troponin I, to evaluate the extent of myocardial cell injury. There are several reports about plasmapheresis, GAS 914, CVF or other agent on myocardial function preservation against HAR,1)8)12)28) but there are few studies that analyzed cardiac enzyme to determine the extent of myocardial injury.
Our results of heart beating time, morphologic changes and cardiac enzyme levels reflect that the use of plasmapheresis, GAS914, CVF, and steroid has a protective effect on xenoperfused piglet heart, particularly in the earlier period of xenoperfusion. These treatments delayed and weakened xenoperfused myocardial injury. Although individual application of plasmapheresis, GAS914, CVF and steroid may have exerted protective effect on the xenotransplanted heart against HAR, applying a combination of these treatments may have exerted more protective effects than would have conferred by monotherapy with one treatment. Depletion of natural antibodies by various methods has resulted in prolonged survival of xenografted organs in the pig-to-primate combination.6)22) In our previous experiment,1) the duration of porcine heart beat in pig-to-dog heterotopic transplantation model without plasmapheresis was on average 5 minutes, which was prolonged to an average of 90 minutes after plasmapheresis. In another study,23) survival of the xenotransplanted heart in which IgM and IgG were respectively reduced to 95.9%, 80.2% by plasmapheresis, was prolonged to 107±47 minutes, copared to hearts without plasmapheresis, which failed in 9±5 minutes. The survival time of the transplanted heart extended to 240±141 minutes from 3 minutes when the natural antibodies were removed by plasmapheresis and immunoadsorption simultaneously.24) In this report, IgM and IgG were reduced to 93.9%, and 85.5% respectively.24) In our study, IgM and IgG were reduced respectively to 65.3%, 77.4% by plasmapheresis. These relatively low elimination rate of immunoglobulin is the limitation of ex vivo experiment. The total titer of natural antibodies were eliminated to a higher extent in group 3 treated with GAS914. Although we did not know the amount of anti-Gal specific antibody removed by GAS914 precisely, qw presume the effect of GAS914 on the antibody removal because the total elimination rates of IgM and IgG were increased from 65.3% and 77.4% in group 2 to 83.7% and 84.8% in group 3, respectively.
Hemolysis is considered a sign of graft vs. host rejection. However, the mechanisms of hemolysis in organ xenotransplantation have not yet been fully elucidated.9) Two factors may be implicated in hemolysis in our study. First, is mechanical hemolysis induced by the circuit itself, and the second is related to immunologic reactions. When we think the circuit conditions were identical to all groups, immunologic reaction can be considered a main cause of hemolysis.25) Endothelial damage and dysfunction of the xenoperfused organ may lead to deposition of fibrin and formation of platelet thrombi.25) This fibrin might have directly damage the perfused erythrocytes, resulting in intravascular hemolysis.9) We had expected that the extent of hemolysis would be lowest in the combined treatment group (group 3) among the xenograft groups before commencing this study. However, the results showed that more pretreatment administered, the more hemolysis occurred. Therefore, we inferred that procedures used in group 3 might have rendered the perfused erythrocyte membrane susceptible to hemolysis by the circuit, or by immunologic reaction. A report discussed that the major complication of plasmapheresis using membrane filtration was hemolysis.26) In another study, CVF was proven to be a proteolytic enzyme, thrombin-like substance and exerted hemorrhagic inclination.27) Thus, plasmapheresis and CVF could be used to suppress myocardial injury against HAR, but it is necessary to determine ways of minimizing hemolysis before applying these agents. We pondered if hemolysis impose a major risk on xenoperfused cardiac function, similar to that in kidney, liver and lung functions. However, our results showed that hemolysis exerted less impact on cardiac function than on other organs. Because the hearts in group 4 beat strongly for over 3 hours, despite the occurrence of hemolysis after 30 minutes, and the hearts in group 3 beat longer than that in group 1, even though hemolysis in group 3 was much more severe than in group 1 (Fig. 5A).
The most important risk factor determining cardiac function was the potassium level, rather than hemolysis in our study. Potassium levels sharply upsurged to 10-14 mmol/L in the early perfusion period in all xenograft groups (Fig. 5B). This increased extracellular potassium disturbed heart beat in the early perfusion period. Although the levels of released cardiac enzymes in group 3 were similar or even lower than that in group 4, and although the morphological changes of the myocardium in group 3 was similar to that in group 4, the duration of transplanted heart beat in group 3 was much shorter than that in group 4. This remarkable difference in heartbeat resulted from difference in extracellular potassium levels (Fig. 5B). Increased extracellular potassium levels in the early perfusion period were regarded as a side effect of hemolyzed perfused RBC or myocyte destruction. However, the increased potassium levels were not correlated with the extent of hemolysis in all groups (Fig. 5A). The main source of increased potassium is likely from injured piglet myocytes, rather than hemolyzed RBC. Thus, potassium level could be a parameter demonstrating myocardial cell injury in ex vivo cardiac perfusion models.
In this study, we investigated the major risk factors of xenoperfused cardiac function. Our data, including duration of heart beat, histological findings, and cardiac enzyme levels show that therapy with plasmapheresis, GAS914, CVF and steroid may reduce and delay the destruction of xenoperfused porcine myocardium by HAR. However, these therapy did not ameliorate myocardial function because of increased extracellular potassium caused by HAR.
In conclusion, one major risk factor that impairs graft function in xenoperfused heart was the high level of extracellular potassium, and not hemolysis, in our ex vivo study.
Acknowledgments
This study was supported by grants No.04-2006-094-0 and 04-2007-091-0 from the Seoul National University Hospital Research Fund, and by the Korean Society of Cardiology (Project No.: 06-2004-149-0).
References
1. Lee JR, Kim HK, Kim JS, et al. Effect of antibody titer on xenograft survival in pig-to-dog heterotropic cardiac xenotransplantation. Korean J Thorac Cardiovasc Surg. 2004; 37:391–400.
2. Sachs DH. The pig as a xenograft donor. Pathol Biol (Paris). 1994; 42:217–219. PMID: 8090569.
3. Sykes M, Lee LA, Sachs DH. Xenograft tolerance. Immunol Rev. 1994; 141:245–276. PMID: 7868155.
4. Tonomura N, Shimizu A, Wang S, et al. Pig islet xenograft rejection in a mouse model with an established human immune system. Xenotransplantation. 2008; 15:129–135. PMID: 18447886.
5. Rydberg L, Hallberg E, Bjorck S. Studies on the removal of anti-pig in the human by plasmapheresis/immunoadsorption. Xenotransplantation. 1995; 2:253–259.
6. Leventhal JR, John R, Fryer JP, et al. Removal of baboon and human antiporcine IgG and IgM natural antibodies by immunoadsorption: results of in vitro and in vivo studies. Transplantation. 1995; 59:294–300. PMID: 7839454.
7. Shimizu A, Hisashi Y, Kuwaki K, et al. Thrombotic microangiopathy associated with humoral rejection of cardiac xenografts from alpha 1, 3-galactosyltransferase gene-knockout pigs in baboons. Am J Pathol. 2008; 172:1471–1481. PMID: 18467706.
8. Kimikawa M, Agishi T, Teraoka S, Suga H, Hayasaka Y, Ota K. Prolongation of cardiac xenograft survival by double filtration plasmapheresis and ex vivo xenoantibody adsorption. Transplant Proc. 1992; 24:447. PMID: 1566383.
9. Nishitai R, Ikai I, Matsuo K, et al. Influence of extracorporeal porcine liver perfusion on nonhuman primates: minimizing hemolysis improves subsequent survival. Liver Transpl. 2001; 7:615–622. PMID: 11460229.
10. Macchiarini P, Mazmanian GM, Oriol R, et al. Ex vivo lung model of pig-to-human hyperacute rejection. J Thorac Cardiovasc Surg. 1997; 114:315–325. PMID: 9305182.
11. Baek SH. The current concept of cell therapy for heart failure. Korean Circ J. 2005; 35:415–423.
12. Kroshus TJ, Bolman RM, Dalmasso AP. Selective IgM depletion prolongs organ survival in an ex vivo model of pig-to-human xenotransplantation. Transplantation. 1996; 62:5–12. PMID: 8693544.
13. Katopodis AG, Warner RG, Duthaler RO, et al. Removal of anti-Galα1,3Gal xenoantibodies with an injectable polymer. J Clin Invest. 2002; 110:1869–1877. PMID: 12488437.
14. Tanemura M, Yin D, Chong AS, Galili U. Differential immune responses to α-gal epitopes on xenografts and allografts: implications for accommodation in xenotransplantation. J Clin Invest. 2000; 105:301–310. PMID: 10675356.
15. Brandl U, Michel S, Erhardt M, et al. Administration of GAS914 in an orthotopic pig to baboon heart transplantation model. Xenotransplantation. 2005; 12:134–141. PMID: 15693844.
16. Walpen AJ, Mohacsi P, Frey C, Roos A, Daha MR, Rieben R. Activation of complement pathways in xenotransplantation: an in vivo study. Transpl Immunol. 2002; 9:271–280. PMID: 12180841.
17. Kim CH, Zo JH, Seo JD, Lee YW, Brooks E. Evolutional change of vasoactive substances in rat model of chronic heart failure. Korean Circ J. 1997; 27:767–773.
18. Kobayashi T, Taniguchi S, Neethling FA, et al. Delayed xenograft rejection of pig-to-baboon cardiac transplants after cobra venom factor therapy. Transplantation. 1997; 64:1255–1261. PMID: 9371665.
19. Vogel CW, Fritzinger DC, Hew BE, Thorne M, Bammert H. Recombinant cobra venom factor. Mol Immunol. 2004; 41:191–199. PMID: 15159065.
20. Grimm H, Mages P, Lindemann G, et al. Evidence against a pivotal role of preformed antibodies in delayed rejection of a guinea pig-to-rat heart xenograft. J Thorac Cardiovasc Surg. 2000; 119:477–487. PMID: 10694606.
21. Oberholzer J, Yu D, Triponez F, et al. Decomplementation with cobra venom factor prolongs survival of xenografted islets in a rat to mouse model. Immunology. 1999; 97:173–180. PMID: 10447729.
22. Fischel RJ, Bolman RM 3rd, Platt JL, Najarian JS, Bach FH, Matas AJ. Removal of IgM antiendothelial antibodies results in prolonged cardiac xenograft survival. Transplant Proc. 1990; 22:1077–1078. PMID: 2190373.
23. Suga H, Ishida H, Kimikawa M, et al. Prolongation of cardiac xenograft function after reduction of natural antibodies using double filtration plasmapheresis. ASAIO Trans. 1991; 37:M433–M434. PMID: 1751224.
24. Sato Y, Kimikawa M, Suga H, et al. Prolongation of cardiac xenograft survival by double filtration plasmapheresis and ex vivo immunoadsorption. ASAIO J. 1992; 38:M673–M675. PMID: 1457946.
25. Satoh S, Terajima H, Yagi T, et al. Humoral injury in porcine livers perfused with human whole blood. Transplantation. 1997; 64:1117–1123. PMID: 9355826.
26. Yeh JH, Chen WH, Chiu HC. Complication of double-filtration plasmapheresis. Transfusion. 2004; 44:1621–1625. PMID: 15504168.
27. Tambourgi DV, dos Santos MC, Furtado M de F, de Freitas MC, da Silva WD, Kipnis TL. Pro-inflammatory activities in elapsed snake venoms. Br J Pharmacol. 1994; 112:723–727. PMID: 7921595.
28. Ahn JM, Kim JJ, Song MG, et al. The efficacy and safety of an immunosuppressive regimen including the use of mycophenolate mofetil and an interleukin-2 monoclonal antibody in heart transplant patients. Korean Circ J. 2006; 36:794–801.
Fig. 1
Diagram of the isolated, non-working heart perfusion circuit. Ao: aorta, RA: right atrium, RV: right ventricle, PA: pulmonary artery, LA: left atrium, LV: left ventricle.
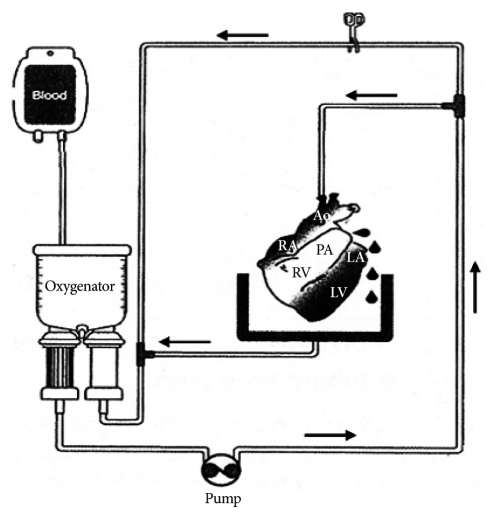
Fig. 2
Histologic findings of xenoperfused porcine myocardium following perfusion in group 1 (A: 10 minutes, B: 60 minutes) and group 2 (C: 10 minutes, D: 60 minutes). Typical features of HAR were noted and myocardial destruction began within 10 minutes of perfusion (A), and almost all cells were destroyed within 60 minutes in group 1 (B). Morphological changes and congestion were noted in group 2 also (C and D), but delayed and milder than in group 1 (A and B) (H&E, Magnification ×400).
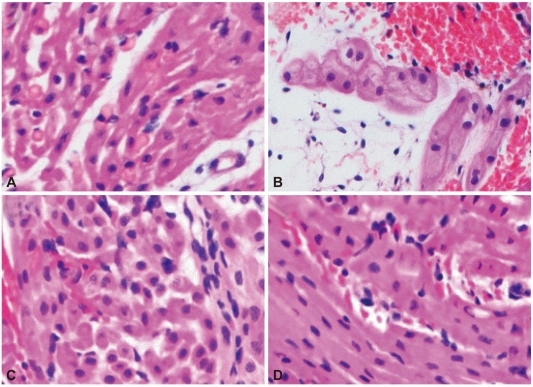
Fig. 3
Histologic findings of xenoperfused porcine myocardium after perfusion in group 3 (A: 60 minutes, B: 180 minutes) and group 4 (C: 60 minutes, D: 180 minutes). The degree of destruction was less and delayed in group 3, compared to groups 1 and 2 (Fig. 2B and D). The myocardial cell contour was maintained relatively well in group 4, and the changes in myocytes in group 3 was similar to that in group 4 (H&E, Magnification ×400).
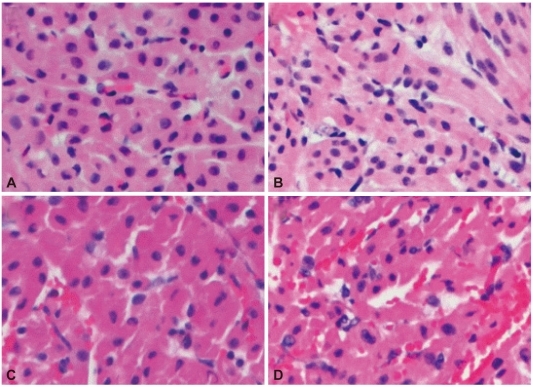
Fig. 4
The levels of Troponin I (A) and CK-MB (B). They increased gradually in all groups, including group 4, especially after perfusion began in group 1, and were lowest in group 3.
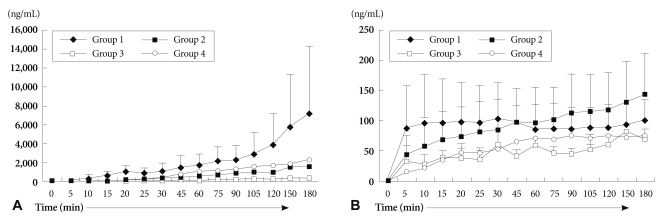
Fig. 5
The levels of serum hemoglobin (A) and potassium (B). Serum hemoglobin levels were higher in group 3 than in groups 1 and 2 (p=0.018). Hemolysis also occurred similarly in group 4. Extracellular potassium levels were increased sharply from the beginning of perfusion in xenograft groups. Notice that the potassium level of the group 4 was increased mildly (p=0.043).
