Abstract
Background and Objectives
The purpose of this study was to evaluate the relationship between myocardial strain and coronary flow reserve (CFR) in the prediction of myocardial functional recovery after acute myocardial infarction (AMI).
Subjects and Methods
Consecutive patients with anterior ST elevation AMI were analyzed. Left ventricular (LV) strain, determined by 2-dimensional speckle tracking imaging and CFR, determined by intracoronary flow measurement, were obtained on the same day, 3-5 days after primary percutaneous coronary intervention. A-strain was defined as the mean systolic longitudinal strain of 11 LV segments (out of 18) assumed to be supplied by the left anterior descending coronary artery (LAD). Functional recovery was defined as improved wall motion >1 grade seen in at least 2 contiguous dysfunctional segments by echocardiography at the 6-month follow-up.
Results
Of 20 patients, 8 patients had preserved CFR (>2.0) and 12 patients had impaired CFR (≤2.0). There were no differences between the 2 CFR groups in LV ejection fractions and wall motion score indices in the LAD territory. However, A-strain was greater in patients with preserved CFR than in patients with impaired CFR (-6.4±2.0% vs. -4.6±1.4%, p=0.03). A-strain and CFR correlated well with each other (r=-0.49, p=0.03). Ten of 20 patients showed functional recovery at 6 months. Of clinical and echocardiographic parameters, A-strain was the only predictor of recovery (odds ratio 2.02, 95% confidence interval=1.03-3.97, p=0.04). For predicting recovery, the sensitivity and specificity were 80.0% and 80.0%, respectively, for CFR (cutoff=1.60), and 60.0% and 90.0%, respectively, for A-strain (cutoff=-6.13%).
In patients with acute myocardial infarction (AMI), primary percutaneous coronary revascularization has been widely performed to achieve early myocardial tissue reperfusion. However, successful revascularization of the infarct-affected coronary artery does not necessarily guarantee adequate myocardial salvage.1)2) Myocardium reperfused by the patent infarct-affected coronary artery may remain akinetic because of transient stunning or persistent nonviability, even with successful reperfusion therapy,3) and the infarct core may undergo limited reperfusion because of injury to the microvasculature and its subsequent obstruction. Therefore, it is difficult to predict whether reperfused myocardium will be viable until improvement of regional wall motion is observed during follow-up.
Previous studies have suggested that coronary flow reserve (CFR) can be used to evaluate microvascular function and that it may also help in assessing myocardial viability. Consequently, it may predict additional recovery of left ventricular (LV) wall motion in patients after AMI.4)5)
Recently-developed 2-dimensional speckle tracking (2DS) imaging technology enables quantification of regional myocardial function with high temporal resolution and without the tethering effects and angle dependence of Doppler measurements.6)7) Longitudinal myocardial strain measured immediately after reperfusion by percutaneous coronary intervention (PCI) has been shown to predict infarct size, LV remodeling, and adverse events in anterior wall AMI.8)9) However, the clinical relationship between myocardial strain and CFR in assessing myocardial functional recovery after AMI has not been well studied. Therefore, the purpose of this study was to evaluate and compare measurements of myocardial strain and CFR as predictors of myocardial functional recovery after AMI in patients treated by primary percutaneous coronary revascularization.
This prospective study enrolled consecutive patients admitted with their first anterior AMI. Study inclusion criteria were: 1) ST-elevation MI on electrocardiography (diagnosis based on standard criteria)10); 2) successful primary PCI, defined as residual stenosis <30% and thrombolysis in myocardial infarction (TIMI) flow grade >2, using a single stent in the left anterior descending coronary artery (LAD), and performed within 12 hours from the onset of symptoms or between 12 and 24 hours for persistent chest pain; 3) no significant stenosis of other coronary arteries (<50% stenosis); 4) presence of akinetic walls with more than 2 contiguous segments in 1 wall supplied by the LAD and normal remote walls on conventional 2-dimensional echocardiography on the day of the study.
Patients with significant arrhythmias, valvular heart disease, or cardiogenic shock were not included. Informed consent was obtained from every patient before cardiac catheterization.
Conventional 2-dimensional Doppler echocardiography (2DE) and 2DS imaging were performed during the 3-5 day period after primary PCI. An intracoronary Doppler study to determine blood flow velocities and CFR was also obtained on the same day. A follow-up 2DE was performed 6 months after primary PCI.
A commercially available echocardiography system (Vivid 7; GE Medical Systems, Horten, Norway) with a 3.5 MHz phased-array transducer was used for both conventional 2DE and 2DS imaging. LV volume and ejection fraction were calculated from the apical 4- and 2-chamber views using the biplane Simpson's method. Mitral inflow during diastole was obtained by pulsed-wave Doppler echocardiography with the sample volume placed between mitral leaflet tips and mitral annulus velocities were obtained from the septal annulus by tissue Doppler imaging. LV regional wall motion was assessed by 2DE and the wall motion score index (WMSI) was calculated and scored according to the American Society of Echocardiography 16-segment model11) as follows: 1=normal, 2=hypokinesia, 3=akinesia, 4=dyskinesia. Nine of 16 LV wall segments were assumed to be supplied by the LAD and included the entire anterior wall, the apical segment of the inferior wall, basal and midsegments of the anteroseptum, apical and midsegments of the septum and the apical segment of the lateral wall. The A-WMSI was defined as a WMSI of the 9 segments supplied by the LAD. Recovery was considered to be functional on the 6-month follow-up 2DE if the patient's wall motion improved in at least 2 contiguous dysfunctional segments by >1 grade. Echocardiographic parameters, including LV ejection fraction and regional WMSs were measured by 2 independent observers who were blinded to the results of CFR and strain measurements. All parameters were measured 3 times and mean values were calculated.
Second-harmonic B-mode images of apical 4-chamber, 2-chamber and long-axis LV views were obtained and digitally stored in cineloop format for offline analysis. Three cardiac cycles were stored during breath-hold. Grayscale images were obtained at 69±10 frames per second. All recordings were stored digitally. Echocardiographic recordings were analyzed using dedicated software (EchoPAC-PC version 10.0; GE Medical Systems). 2DS software identified the endocardial border, and myocardial motion was automatically tracked in each imaging view. Segmental longitudinal strain was automatically calculated as the mean strain within each segment. In segments with poor tracking, the observer readjusted the endocardial trace line until a better tracking score was achieved. If this was not possible, the segment was excluded. An 18-segment LV model was automatically obtained from the apical 4-chamber, 2-chamber and long-axis views in off-line analysis. Peak systolic strain was defined as the peak negative strain value during systole. End of systole was defined as aortic valve closure in the apical long-axis view and peak negative strain was the maximum negative strain value during systole (Fig. 1). Eleven of the 18 segments were assumed to be supplied by the LAD, and A-strain was defined as the mean systolic longitudinal strain calculated from the measured segments supplied by the LAD. Mean peak systolic longitudinal strain values from 18 apical segments were calculated to determine LV global strain. All parameters were determined from 3 consecutive beats to minimize measurement variability. Cardiac cycles with extrasystolic beats, postextrasystolic beats, or any disturbance of rhythm were excluded.
Intracoronary flows were measured using a 0.014 inch Doppler probe-tipped guide wire (12 MHz, FloWire; Cardiometrics Inc., Mountain View, CA, USA) in the infarct-affected coronary artery, with the tip at the distal extremity of the stent.12) Average peak velocities were measured at baseline and after intracoronary administration of adenosine (12-18 mg). CFR was obtained from the ratio of baseline velocity to hyperemic average peak velocity (Fig. 2). All measurements were repeated 3 times and mean values were calculated. A cut-off value of 2.0 was regarded as preserved CFR after primary PCI, based on previous our study.13)
Data are presented as mean±standard deviation. Comparisons of continuous variables between groups were tested using the Wilcoxon signed rank test. Categorical data were compared by chi-square analysis. Relationships between different parameters were assessed by correlation analysis (Spearman method). To analyze independent predictors of myocardial functional recovery, univariate factors with p<0.1 were analyzed by forward stepwise logistic regression (multivariate analysis). All analyses were performed using commercially-available statistical software {Statistical Package for the Social Sciences (SPSS), version 13.0; SPSS Inc., Chicago, IL, USA}. A p less than 0.05 was considered to indicate statistical significance.
Twenty-three consecutive patients were enrolled and 20 patients (17 men and 3 women; mean age, 59±13 years) completed this study.
One patient did not return for follow-up 2DE and 2 patients did not have eligible 2DS images for the analysis. Primary PCI with stenting was successfully performed and TIMI >2 reflow was seen in all patients.
Of 20 patients, 8 patients had preserved CFR (>2.0) and 12 patients had impaired CFR (≤2.0). Creatine kinase (CK)-MB was higher in patients with impaired CFR than in patients with preserved CFR (Table 1). There were no significant differences seen between the 2 groups (impaired versus preserved CFR) in LV ejection fractions, A-WMSIs and other echocardiographic parameters (Table 2). However, A-strain was greater in patients with preserved than in patients with impaired CFR (-6.4±2.0% vs. -4.6±1.4%, p=0.03). A-strain and CFR were correlated with each other (r=-0.49, p=0.03) (Fig. 3), but neither correlated with baseline A-WMSI (r=-0.01, p=0.97, and r=-0.16, p=0.49, respectively).
Ten of 20 patients showed functional recovery at 6 months. In patients with recovery, LV end diastolic and systolic volumes were decreased (65.5±10.8 mL/m2 to 59.9±10.3 mL/m2, p=0.05 and 41.0±12.8 mL/m2 to 30.0±8.2 mL/m2, p=0.01, respectively) and LV ejection fraction was increased (38.8±10.4% to 55.9±10.3%, p<0.001). A-WMSI (2.44±0.38 to 1.76±0.40, p<0.001) and A-strain (-6.5±2.3% to -12.4±2.6%, p<0.001) were also improved. However, in patients with no recovery, LV end diastolic and systolic volumes were increased (56.8±12.2 mL/m2 to 68.7±15.6 mL/m2, p=0.05 and 35.2±9.8 mL/m2 to 41.0±14.6 mL/m2, p=0.05, respectively) and LV ejection fraction was not improved (38.0±9.1% to 39.0±10.8%, p=0.74). A-WMSI (2.54±0.30% to 2.55±0.28%, p=0.86) and A-strain (-5.5±2.0% to -6.6±3.3%, p=0.21) were also not improved.
CFR was higher in the 10 patients with recovery than in the 10 patients with no recovery (2.0±0.5 vs. 1.6±0.3, respectively, p=0.04). Of the clinical and echocardiographic parameters, the univariate predictors of myocardial functional recovery after AMI were CK-MB (p=0.02), early diastolic mitral velocity deceleration time (p=0.03) and A-strain (p=0.02). By multivariate analysis, A-strain remained the only predictor of myocardial functional recovery as determined by 6-month follow-up echocardiography (odds ratio 2.02, 95% confidence interval 1.03 to 3.97) (p=0.04). Based on receiver operating characteristic curve analysis,14) the sensitivity and specificity for predicting myocardial recovery were 80.0% and 80.0%, respectively, for CFR (cutoff=1.60), and 60.0% and 90%, respectively, for A-strain (cutoff=-6.13%).
Inter- and intraobserver reproducibilities of A-strain measurements were obtained for analysis by Bland-Altman methods from 10 randomly chosen patients.15) The inter- and intraobserver variabilities were 3.9% and 2.2%, respectively.
We investigated the relationship between myocardial strain and CFR 3-5 days after successful primary PCI for anterior AMI. Myocardial strain measured by 2DS imaging correlated well with CFR determined by intracoronary measurement and can be used noninvasively to predict myocardial functional recovery.
Extensive transmural scarring is a poor prognostic marker, and a region with extensive transmural scarring is unlikely to show improvement in function after revascularization. However, subendocardial infarcts or stunned myocardium may show improved function after revascularization.
Even if the recovery of contractile function is not observed soon after reperfusion, the presence of viable myocardium in the outer layers of the ventricular wall may contribute to preventing infarct expansion and LV remodeling.16) LV remodeling after AMI is associated with the development of heart failure and mortality.17)18) Therefore, assessment after AMI to determine whether there are optimal microvascular flow, tissue perfusion and sufficient contractile performance in the dysfunctional myocardial segment is important.
Recently-developed 2DS imaging is based on tracking ultrasonic speckles from 2-dimensional echocardiographic images. Reflected ultrasound from tissue is the result of interference by numerous reflected wavelets from an inhomogeneous medium. The interference pattern (resulting in bright and dark pixels in a B-mode image) remains relatively constant for any small region in the myocardium. This unique pattern (fingerprint) is called "speckle". Speckles are followed over a number of successive frames and myocardial velocity is calculated by measuring frame-to-frame changes. The technique is angle-independent, as it is based on the displacement of speckles, defined in respect to the wall. Therefore, 2DS imaging enables quantification of regional myocardial function without tethering effects and with high temporal resolution by semiautomated processing.19) Several studies have examined the utility of strain imaging for assessment of myocardial viability and compared its accuracy with that of cardiac magnetic resonance imaging or dobutamine echocardiogram.20-22) These studies demonstrated that there is a gradual decrease in strain with increasing transmurality of chronic myocardial infarction and ischemia. In AMI, longitudinal strain measured immediately after reperfusion with PCI has been shown to predict infarct size, LV remodeling and adverse events.8)9)
Microvascular injury is associated with greater infarct size and poorer myocardial function23-25) and the evaluation of microvascular function by CFR can assess myocardial viability.4)5) To assess microvascular dysfunction without the effects of no reflow, which has been reported to increase in the first 48 hours after reperfusion,23)26) we measured coronary blood flow velocities and CFR at least 3 days after primary PCI. An experimental study using cardiac magnetic resonance imaging demonstrated that the infarcted myocardium 48 hours after reperfusion had reduced systolic myocardial strain in regions of microvascular obstruction, compared to infarcts with patent microvasculature;27) and Rosen et al.28) showed that a lower myocardial flow reserve is related to reduced regional myocardial strain in asymptomatic individuals.
In our study, dysfunctional myocardium after AMI showed higher CFR, suggesting less impaired microvascular function and had less impaired myocardial strain, suggesting better myocardial deformation function. Therefore, myocardial strain can be used as a noninvasive method to predict myocardial functional recovery after AMI.
It is clinically important to predict myocardial functional recovery for further treatment and prognosis in AMI patients. Myocardial damage and microvascular dysfunction in AMI can be demonstrated quantitatively and noninvasively by observation of impaired myocardial deformation in the clinical setting using simple 2DS imaging, which could also be very helpful in assessing myocardial functional recovery at the bedside after acute coronary reperfusion.
First, the study population was small and this method was only applied to the LAD in patients with anterior AMI. Second, we obtained coronary blood flow velocities and CFR by intracoronary measurements. Although coronary flow velocity data can be obtained by transthoracic 2DE, it is known that in some patients there are difficulties in obtaining adequate spectral Doppler recordings of coronary flow for coronary flow velocity analysis. We were able to confirm stent patency, in addition to obtaining direct measurements of coronary blood flow velocities by use of intracoronary Doppler-tipped guide wires. Third, we only considered hypokinetic or normally-contracting segments as functional recovery by visual assessment at follow-up because improvement in wall motion is clinically important.
However, better quantitative methods, such as single photon emission computed tomography or dobutamine stress echocardiography may be considered to provide a more accurate evaluation of viable myocardium. Fourth, we only assessed longitudinal strain, which is considered to be the primary cardiac motion, but radial, circumferential, and rotational motion may provide additional information about functional recovery. And finally, we did not assess clinical events, because none of the patients developed heart failure, died, or were readmitted during the 6-month follow up.
Figures and Tables
Fig. 1
Measurement of myocardial strain from apical 4- and 2-chamber and apical long axis views by 2-dimensional speckle tracking imaging.
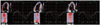
Fig. 2
Intracoronary flow measurement of coronary blood flow velocity and coronary flow reserve (CFR). APV: average peak flow velocity, S: systolic, D: diastolic.
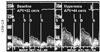
Table 2
Baseline echocardiographic findings in patients with preserved and impaired CFR
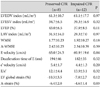
CFR: coronary flow reserve, LVEDV and LVESV: left ventricular end diastolic and systolic volume, LVEF: left ventricular ejection fraction, LAV: left atrial volume, A-WMSI: wall motion score index (WMSI) in the segments supplied by the left anterior descending coronary artery (LAD), E velocity: early diastolic mitral inflow velocity, e'velocity: early diastolic mitral annular velocity, A-strain: mean strain in the segments supplied by the LAD
References
1. Bates ER, Krell MJ, Dean EN, O'Neill WW, Vogel RA. Demonstration of the "no-reflow" phenomenon by digital coronary arteriography. Am J Cardiol. 1986. 57:177–178.
2. Morishima I, Sone T, Mokuno S, et al. Clinical significance of no-reflow phenomenon observed on angiography after successful treatment of acute myocardial infarction with percutaneous transluminal coronary angioplasty. Am Heart J. 1995. 130:239–243.
3. Ito H, Okamura A, Iwakura K, et al. Myocardial perfusion patterns related to thrombolysis in myocardial infarction perfusion grades after coronary angioplasty in patients with acute anterior wall myocardial infarction. Circulation. 1996. 93:1993–1999.
4. Suryapranata H, Zijlstra F, MacLeod DC, van den Brand M, de Feyter PJ, Serruys PW. Predictive value of reactive hyperemic response on reperfusion on recovery of regional myocardial function after coronary angioplasty in acute myocardial infarction. Circulation. 1994. 89:1109–1117.
5. Yoon MH, Tahk SJ, Choi SY, et al. Coronary flow reserve as a predictor of long-term clinical outcome after acute myocardial Infarction. Korean Circ J. 2002. 32:756–765.
6. Cho KI, Park JH, Park JR, et al. Assessment of left ventricular function in symptomatic patients with myocardial bridge using two-dimensional strain. Korean Circ J. 2006. 36:617–625.
7. Pirat B, Khoury DS, Hartley CJ, et al. A novel feature-tracking echocardiographic method for the quantitation of regional myocardial function: validation in an animal model of ischemia-reperfusion. J Am Coll Cardiol. 2008. 51:651–659.
8. Park YH, Kang SJ, Song JK, et al. Prognostic value of longitudinal strain after primary reperfusion therapy in patients with anterior-wall acute myocardial infarction. J Am Soc Echocardiogr. 2008. 21:262–267.
9. Vartdal T, Brunvand H, Pettersen E, et al. Early prediction of infarct size by strain Doppler echocardiography after coronary reperfusion. J Am Coll Cardiol. 2007. 49:1715–1721.
10. Alpert JS, Thygesen K, Antman E, Bassand JP. Myocardial infarction redefined: a consensus document of The Joint European Society of Cardiology/American College of Cardiology Committee for the redefinition of myocardial infarction. J Am Coll Cardiol. 2000. 36:959–969.
11. Schiller NB, Shah PM, Crawford M, et al. American Society of Echocardiography Committee on Standards, Subcommittee on Quantitation of Two-Dimensional Echocardiograms. Recommendations for quantitation of the left ventricle by two-dimensional echocardiography. J Am Soc Echocardiogr. 1989. 2:358–367.
12. Doucette JW, Corl PD, Payne HM, et al. Validation of a Doppler guide wire for intravascular measurement of coronary artery flow velocity. Circulation. 1992. 85:1899–1911.
13. Lim DS, Kim YH, Lee HS, et al. Coronary flow reserve is reflective of myocardial perfusion status in acute anterior myocardial infarction. Catheter Cardiovasc Interv. 2000. 51:281–286.
14. Erdreich LS, Lee ET. Use of relative operating characteristic analysis in epidemiology: a method for dealing with subjective judgement. Am J Epidemiol. 1981. 114:649–662.
15. Bland JM, Altman DG. Statistical methods for assessing agreement between two methods of clinical measurement. Lancet. 1986. 1:307–310.
16. Irimpen AM, Tenaglia AN, Shin DJ, Buda AJ. Lack of ventricular remodeling in non-Q-wave myocardial infarction. Am Heart J. 1996. 131:466–471.
17. Pfeffer MA, Braunwald E. Ventricular remodeling after myocardial infarction: experimental observations and clinical implications. Circulation. 1990. 81:1161–1172.
18. St John Sutton M, Pfeffer MA, Plappert T, et al. Quantitative two-dimensional echocardiographic measurements are major predictors of adverse cardiovascular events after acute myocardial infarction: the protective effects of captopril. Circulation. 1994. 89:68–75.
19. Perk G, Tunick PA, Kronzon I. Non-Doppler two-dimensional strain imaging by echocardiography: from technical considerations to clinical applications. J Am Soc Echocardiogr. 2007. 20:234–243.
20. Chan J, Hanekom L, Wong C, Leano R, Cho GY, Marwick TH. Differentiation of subendocardial and transmural infarction using two-dimensional strain rate imaging to assess short-axis and long-axis myocardial function. J Am Coll Cardiol. 2006. 48:2026–2033.
21. Sachdev V, Aletras AH, Padmanabhan S, et al. Myocardial strain decreases with increasing transmurality of infarction: a Doppler echocardiographic and magnetic resonance correlation study. J Am Soc Echocardiogr. 2006. 19:34–39.
22. Becker M, Lenzen A, Ocklenburg C, et al. Myocardial deformation imaging based on ultrasonic pixel tracking to identify reversible myocardial dysfunction. J Am Coll Cardiol. 2008. 51:1473–1481.
23. Wu KC, Kim RJ, Bluemke DA, et al. Quantification and time course of microvascular obstruction by contrast-enhanced echocardiography and magnetic resonance imaging following acute myocardial infarction and reperfusion. J Am Coll Cardiol. 1998. 32:1756–1764.
24. Nijveldt R, Beek AM, Hofman MB, et al. Late gadolinium-enhanced cardiovascular magnetic resonance evaluation of infarct size and microvascular obstruction in optimally treated patients after acute myocardial infarction. J Cardiovasc Magn Reson. 2007. 9:765–770.
25. Lim DS, Kim YH, Lee HS, et al. Relation between perfusion status of dysfunctional myocardium and coronary flow reserve in acute myocardial infarction. Korean Circ J. 1998. 28:164–172.
26. Rochitte CE, Lima JA, Bluemke DA, et al. Magnitude and time course of microvascular obstruction and tissue injury after acute myocardial infarction. Circulation. 1998. 98:1006–1014.
27. Gerber BL, Rochitte CE, Melin JA, et al. Microvascular obstruction and left ventricular remodeling early after acute myocardial infarction. Circulation. 2000. 101:2734–2741.
28. Rosen BD, Lima JA, Nasir K, et al. Lower myocardial perfusion reserve is associated with decreased regional left ventricular function in asymptomatic participants of the multi-ethnic study of atherosclerosis. Circulation. 2006. 114:289–297.