Abstract
Background and Objectives
Vascular smooth muscle cell (VSMC) proliferation is responsible for the restenosis of previously inserted coronary stents. Angiotensin II (Ang II) is known to regulate VSMC proliferation. LKB1, a serine/threonine kinase, interacts with the p53 pathway and acts as a tumor suppressor.
Materials and Methods
We assessed the association of Ang II and the expression of LKB1 in primary cultured murine VSMCs and neointima of the Sprague Dawley rat carotid artery injury model. We created carotid balloon injuries and harvested the injured carotid arteries 14 days after the procedure.
Results
Ang II increased LKB1 expression in a time-dependent manner and peaked at an Ang II concentration of 10-7 mole/L in VSMCs. In the animal experiment, neointima was markedly increased after balloon injury compared to the control group. Immunohistochemical studies showed that LKB1 expression increased according to neointima thickness. Ang II augmented LKB1 expression after the injury. Western blot analysis of LKB1 with carotid artery lysate revealed the same pattern as LKB1 immunohistochemistry. Increased LKB1 expression started at 5 days after the balloon injury, and peaked at 14 days after the injury. Although LKB1 expression was increased after the injury, LKB1 kinase activity was not increased. Ang II or balloon-injury increased the expression of LKB1 although the LKB1 activity was reduced.
Coronary artery disease is one of the leading causes of death and disability. Percutaneous coronary intervention (PCI) is one of the most widely used therapeutic procedures in the treatment of obstructive coronary artery disease. Although drug-eluting stents are commonly used to decrease in-stent restenosis (ISR), the ISR after PCI remains a major limitation of the treatment. The proliferative action of vascular smooth muscle cells (VSMCs) has been found to play an important role in restenosis through neointima formation after PCI.1-4)
Peutz-Jeghers syndrome (PJS) is a rare autosomal dominantly inherited disorder in which patients develop gastrointestinal hamartomatous polyposis, and mucocutaneous pigmentation on skin and oral mucosa. PJS is also associated with a 15-fold increased risk of developing cancer and a functional loss of serine/threonine kinase LKB1. Inactivation of germ line mutations in the LKB1 gene underlies the majority of PJS cases.5) LKB1 is therefore believed to function as a tumor suppressor.6)7) LKB1/STK11 encodes a serine/threonine protein kinase with widespread expression during murine embryonic development. LKB1-deficient mice undergo embryonic lethality during the midgestational period, showing severe vascular abnormalities due to defective vasculogenesis associated with a tissue-specific deregulation of vascular endothelial growth factor.7) Overexpression of wild-type LKB1 in vitro induces a G1 cell cycle arrest and suppresses growth in tumor cell lines. Overexpressed LKB1 increases expression of several p21 (CDK inhibitor), p53 responsive genes.8-11) This tumor suppressor protein may have an important role in atherosclerosis, especially in restenosis. Cellular stress (such as deoxyribonucleic acid damage, hypoxia, and oxidized lipoprotein) activates p53. LKB1 interacts with the p53 pathway, suppresses cellular proliferation, and has a proapoptotic effect.8)11-13) These findings support the hypothesis that LKB1 protein kinase functions regulate cellular proliferation as a tumor suppressor.
We examined whether LKB1 regulates VSMC proliferation and neointima formation in rat carotid artery injury models.
VSMCs were prepared from the aorta of 4-6 week old Sprague Dawley (SD) rats (Charles River Laboratories, Japan). Cells were grown in DMEM/F12 containing 10% (v/v) fetal bovine serum (FBS), penicillin (100 units/mL), and streptomycin (100 mg/mL) (Gibco/BRL). Cells were passaged at 90% confluence and utilized between passages 4 and 10. Cells grown confluently in growth medium were kept for 48 hours in DMEM/F12 medium containing 5×10-7 M insulin (Sigma), 5 mg/mL transferrin (Sigma), and 0.2 mM ascorbic acid (Sigma). Quiescence was induced by incubation for 72 hours in low-mitogen (0.5% FBS) medium.
Procedures involving animals were in accordance with the Guide for Experimental Animal Research from the Laboratory for Experimental Animal Research, and the Clinical Research Institute, Chungnam National University Hospital. The model of balloon injury was based on that described by Clowes et al.1)14-17) Male SD rats (mean weight 350 g) aged 8 to 10 weeks were used. Briefly, under xylazine (5 mg/kg intraperitoneally; Bayer, Korea) and ketamine hydrochloride (50 mg/kg intraperitoneally; Yuhan Corp, Korea) anesthesia, the right external carotid arteries were exposed, and the common carotid arteries were denuded of endothelium by the intraluminal passage of a 2-French embolectomy arterial catheter (Baxter Healthcare Corp), which was passed to the proximal common carotid artery and withdrawn. This procedure was repeated five times. In the angiotensin II (Ang II) group, Ang II (0.5 mg/kg/day), purchased from Sigma Aldich, was infused via miniosmotic pumps (ALZET, CA, USA), which were implanted immediately after the injury, with Ang II released over two weeks.
At the time of euthanasia, a midline abdominal incision was performed, and the distal abdominal aorta was exposed. Perfusion fixation used phosphate-buffered saline and 4% paraformaldehyde over five minutes at 120 mmHg. The injured segment of the right common carotid artery was dissected from the surrounding tissue, fixed in 10% formalin, and embedded in paraffin. Several 4 µm sections were cut from each specimen. Sections were stained with hematoxylin-eosin (H&E) for conventional light microscopic analysis. Morphometric analyses of the arterial segments were performed by an observer blinded to the study groups using computerized image processing and an analysis program (ImageJ, Version 1.41 for Windows, NIH).
Immunohistochemistry was performed with monoclonal antibodies to LKB1 (Cell Signaling, USA) using an EnVision kit (DAKO, Carpinteria, CA, USA) and hematoxylin stain. Diaminobenzidine was used to visualize the sites of primary antibody binding to the antigen. Expression of LKB1 was graded according to the following scale by two investigators blinded to the experimental conditions: negative (0), mild staining (1), moderate staining (2), and intense staining (3).
Western blot assays were performed as previously described18) with antibodies against LKB1 (Cell signaling technology, MA, USA). Briefly, VSMCs were growth arrested for 24 hours and treated with various cytokines for 48 hours. Cells were harvested in sodium dodecyl sulfate sample buffer. Western blot analysis was performed with an anti-LKB1 antibody.
After vascular injury, the carotid arterial tissue samples were homogenized with radio immunoprecipitation assay (RIPA) buffer. The carotid artery lysates containing an equal amount of protein from each condition were analyzed by western blotting for LKB1. All determinations were performed in triplicate.
Kinase assays were performed as previously described.19) After vascular injury, the carotid arterial tissue samples were homogenized with 400 µL of RIPA buffer. The carotid artery lysates containing an equal amount of protein (100 µg) were overnight incubated with anti-LKB1 antibody (Santa Cruz) and centrifuged using protein A-G agarose. LKB1 Kinsae buffer, LKB1 substrate 1 µL, Mg++/ATP 2 µL, and [γ-32P] ATP 1 µL were added. After incubation for 30 minutes at 30℃ with constant agitation, the reaction was stopped by adding 5 µL of 3% phosphoric acid. A 20 µL aliquot was spotted onto the center of a P81 paper square (Upstate Biotechnology), allowed to dry, then washed three times with 0.75% phosphoric acid and aceton. After transferring the paper square to a sealable plastic bag and adding 3 mL of scintillation cocktail, radioactivity was measured in a scintillation counter (TRI-CARB 1500, PACKARD).
All data were expressed as mean±standard error of mean. Statistical analysis was performed using commercial statistical analysis software. Continuous variables were compared using Mann-Whitney U-test and Student t-tests. Categorical variables were compared using χ2-test. A value of p<0.05 was considered to be statistically significant.
We tested various cytokines such as Ang II, tumor necrosis factor alpha, interferon gamma, tumor growth factor beta and platelet derived growth factor (data not shown). We found increased expression of LKB1 under Ang II stimulated conditions. Treatment with Ang II led to increased serine/threonine kinase LKB1 in rat VSMCs (Fig. 1A). Ang II increased the LKB1 expression showed a bell shaped curve. The LKB1 expression peaked at an Ang II concentration of 10-7 mole/L and then decreased at an Ang II concentration of 10-5 mole/L (Fig. 1B). Induction of LKB1 peaked after 48 hours in the presence of Ang II (100 nM) for the indicated times (Fig. 1C).
H-E staining showed neointima formation 2 weeks after the carotid artery balloon injury. The neointima was formed in the balloon injury group and the balloon injury with Ang II infusion group (Fig. 2A, B and C). There were no significant differences of neointima formation between the injury and the injury with Ang II infusion group. Immunohistochemistry showed increased LKB1 expression after the carotid artery balloon injury compared to the control group (Fig. 2D and E). Ang II augmented the increased expression of LKB1 (Fig. 2F).
Western blot analysis of LKB1 with carotid artery lysate revealed the same pattern as LKB1 immunohistochemistry (Fig. 2I). Western blot of LKB1 expression increased in the carotid artery balloon injury model compared to the control group. Ang II augmented the increased expression of LKB1.
Serial LKB1 staining showed LKB1 expression increased 5 days after the balloon injury and peaked 14 days after the injury according to neointima formation (Fig. 3).
We originally hypothesized that increased LKB1 might inhibit cell proliferation of VSMCs in vitro and also neointima formation in vivo. However, according to our data, Ang II-induced LKB1 actually led to increased proliferation of VSMCs. To elucidate this discrepancy between the hypothesis and the data, we measured LKB1 kinase activity in balloon-injured carotid arteries. Balloon injury increased the expression of LKB1 although LKB1 activity was reduced. This means that the amount of LKB1 protein was not correlated with LKB1 activity (Fig. 4).
In this study, we demonstrated the increased expression of LKB1 in the VSMCs by Ang II and neointima of injured rat carotid artery. Ang II stimulates VSMC proliferation. LKB1 expression was increased at Ang II concentrations of 10-7 mole/L and peaked at 48 hours in VSMCs. Post-injury neointima formation was increased in the injury group and the Ang II infusion group compared with the control group. Also, LKB1 expression was significantly increased in neointima. However, increased LKB1 expression was not correlated with LKB1 activity in the balloon-injured carotid artery. These findings support the hypothesis that the serine/threonine kinase LKB1, a tumor suppressor, increases after vascular injury and is involved in the regulation of VSMC proliferation and apoptosis.
LKB1 is an upstream kinase of AMP-activated protein kinase (AMPK), a cellular energy sensor. It is known that AMPK inhibits Ang II-stimulated VSMC proliferation.20) Although LKB1 is one of the important modulators of AMPK, Ang II did not induce AMPK phosphorylation (data not shown). VSMCs and rabbit aorta expressed the upstream AMPK kinase LKB1 protein, and rottlerin-induced AMPK activation was decreased in VSMCs by overexpression of dominant negative LKB1, suggesting that LKB1 is involved in the upstream regulation of AMPK stimulated by rottlerin.21) These data therefore imply that LKB1 mediates rottlerin-induced activation of AMPK in vascular cells and tissues.
Although LKB1 and AMPK have become attractive therapeutic targets for vascular diseases, the functions of LKB1 and AMPK activation are still not fully understood. AMPK activation suppresses protein synthesis and prevents cardiac myocyte hypertrophy by regulation of the eEF2 kinase/eEF2 axis and/or TSC2-mTOR-P70S6 pathways.22-24) LKB1 has been reported to cause G1 growth arrest in cultured cells via induction of p21WAF1/CIP111) and stimulation of Brg1 ATPase.25) LKB1 kinase activity is required for p21WAF1/CIP1 induction, and overexpression of LKB1 causes p21WAF1/CIP1-induced growth arrest in cells with undetectable or low levels of endogenous LKB1.11) In contrast to kinase activity, although Brg1-associated growth arrest also requires LKB1 kinase activity, wild-type LKB1 and the kinase-deficient form LKB1-SL26 both stimulate Brg1 ATPase.25) This suggests that some regulatory functions of LKB1 may not require its kinase activity.19) As is well known, LKB1 has an autophosphorylation activity to increased its kinase activity. Our data showed that Ang II induces LKB1 expression in VSMCs and that LKB1 expression increased after vascular injury. However, increased LKB1 expression was not correlated with LKB1 kinase activity in the balloon-injured carotid arteries. These findings suggest that some regulatory functions of increased LKB1 in VSMC and neointima do not require kinase activity. There might be some mechanisms which interfere with increased LKB1 expression and kinase activity.
There are several limitations to this study. First, we failed to transfect LKB1 to evaluate the direct effect of LKB1, with or without AII stimulation, on proliferation of VSMC in vitro and in vivo. It is unclear whether Ang II-induced LKB1 plays a positive or negative regulatory role in SMC proliferation. Second, we only checked LKB1 activity after vascular injury without exogenous Ang II infusion. Although endogenous Ang II increased after vascular injury, the mechanisms of the interaction between Ang II, increased LKB1 expression and decreased LKB1 kinase activity is still elusive. Since LKB1 activity is dependent on post-translational modification, such as phosphorylation or acetylation, further study is needed to elucidate the molecular mechanisms of how Ang II increases LKB1 expression and decreases activity in VSMC and neointima formation.
In conclusion, LKB1 was expressed in the VSMCs and neointima. Ang II increases tumor suppressor serine/threonine kinase LKB1 expression in the VSMCs and neointima of the rat carotid artery injury model. These findings were not kinase dependant. The LKB1 might be one of the regulators of VSMC proliferation and neointima formation.
Figures and Tables
Fig. 1
A: VSMCs were growth arrested for 24 hours and treated with Ang II for 48 hours. Cells were harvested in sodium dodecyl sulfate sample buffer. Western blot analysis was performed with anti-LKB1 antibody. The results are representative of 3 experiments. Ang II increases serine/threonine kinase LKB1 in rat VSMCs. B: induction of LKB1 by Ang II in VSMCs. Quiescent VSMCs were maintained under control conditions in the presence of the indicated concentration of Ang II for 48 hours. Induction of LKB1 by Ang II peaked at an Ang II concentration of 10-7 mole/L. C: time response of LKB1 by Ang II. Quiescent VSMCs were maintained in the presence of Ang II (100 nM) for the indicated times. Induction of LKB1 peaked at 48 hours. TNF-α: tumor necrosis factor alpha, Ang II: angiotensin II, VSMCs: vascular smooth muscle cells.
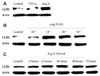
Fig. 2
A, B and C: representative microphotographs of H&E staining in balloon-injured carotid artery at day 14 (200×, n=3/each group). A: sham operation. B: balloon-injured carotid artery. C: balloon-injured and Ang II infusion via a miniosmotic pump. A neointima was found 2 weeks after carotid artery balloon injury. A marked neointima was found after balloon injury (*). D, E and F: representative microphotographs of LKB1 immunohistochemistry stain in balloon-injured carotid artery at day 14 (400×, n=3/each group). D: sham operation. E: balloon-injured carotid artery. F: balloon-injured and Ang II infusion via a miniosmotic pump. LKB1 expression was increased after carotid artery balloon injury. LKB1 expression was more greatly increased by Ang II after carotid artery balloon injury. Expression of LKB1 was graded according to the following scale by two investigators blinded to the experimental conditions: negative (0), mild staining (1), moderate staining (2), and intense staining (3). I: representative western blot of the LKB1 in balloon-injured carotid artery at day 14 (n=3/each group). The carotid artery lysates containing an equal amount of protein from each condition were analyzed by Western blotting for LKB1 using specific antibodies. Western blot of LKB1 expression was similar to immunohistochemistry. LKB1 expression increased after carotid artery balloon injury. LKB1 expression was more greatly increased by Ang II after carotid artery balloon injury.
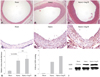
Acknowledgments
This work was supported by grants from the Korean Society of Cardiology and from Chungnam National University Hospital.
References
1. Clowes AW, Reidy MA, Clowes MM. Mechanisms of stenosis after arterial injury. Lab Invest. 1983. 49:208–215.
2. Dussaillant GR, Mintz GS, Pichard AD, et al. Small stent size and intimal hyperplasia contribute to restenosis: a volumetric intravascular ultrasound analysis. J Am Coll Cardiol. 1995. 26:720–724.
3. Hoffmann R, Mintz GS, Dussaillant GR, et al. Patterns and mechanisms of in-stent restenosis: a serial intravascular ultrasound study. Circulation. 1996. 94:1247–1254.
4. Schwartz SM, deBlois D, O'Brien ER. The intima: soil for atherosclerosis and restenosis. Circ Res. 1995. 77:445–465.
5. Hemminki A, Markie D, Tomlinson I, et al. A serine/threonine kinase gene defective in Peutz-Jeghers syndrome. Nature. 1998. 391:184–187.
6. Jenne DE, Reimann H, Nezu J, et al. Peutz-Jeghers syndrome is caused by mutations in a novel serine threonine kinase. Nat Genet. 1998. 18:38–43.
7. Ylikorkala A, Rossi DJ, Korsisaari N, et al. Vascular abnormalities and deregulation of VEGF in Lkb1-deficient mice. Science. 2001. 293:1323–1326.
8. Levine AJ. p53, the cellular gatekeeper for growth and division. Cell. 1997. 88:323–331.
9. Sherr CJ. Mammalian G1 cyclins and cell cycle progression. Proc Assoc Am Physicians. 1995. 107:181–186.
10. Tiainen M, Vaahtomeri K, Ylikorkala A, Makela TP. Growth arrest by the LKB1 tumor suppressor: induction of p21(WAF1/CIP1). Hum Mol Genet. 2002. 11:1497–1504.
11. Tiainen M, Ylikorkala A, Makela TP. Growth suppression by Lkb1 is mediated by a G(1) cell cycle arrest. Proc Natl Acad Sci U S A. 1999. 96:9248–9251.
12. Karuman P, Gozani O, Odze RD, et al. The Peutz-Jegher gene product LKB1 is a mediator of p53-dependent cell death. Mol Cell. 2001. 7:1307–1319.
13. Poon M, Marx SO, Gallo R, Badimon JJ, Taubman MB, Marks AR. Rapamycin inhibits vascular smooth muscle cell migration. J Clin Invest. 1996. 98:2277–2283.
14. Kwon JS, Park NK, Jeong IH, et al. A slight variation in the age of rats commonly used as a carotid artery injury model results in a large difference in neointima formation. Korean Circ J. 2007. 37:78–83.
15. Kwon JS, Park SS, Kim YG, et al. Perivascular delivery of paclitaxel with F-127 pluronic gel inhibits neointimal hyperplasia in a rat carotid injury model. Korean Circ J. 2005. 35:221–227.
16. Joe JH, Lim KS, Jin JY, Kim KS. Effect of udenafil vascular smooth muscle cell proliferation and neointimal hyperplasia in rat carotid artery injury model. Korean Circ J. 2008. 38:320–324.
17. Clowes AW, Reidy MA, Clowes MM. Kinetics of cellular proliferation after arterial injury: I. smooth muscle growth in the absence of endothelium. Lab Invest. 1983. 49:327–333.
18. Sharpless NE, Ramsey MR, Balasubramanian P, Castrillon DH, DePinho RA. The differential impact of p16(INK4a) or p19(ARF) deficiency on cell growth and tumorigenesis. Oncogene. 2004. 23:379–385.
19. Kim DW, Chung HK, Park KC, et al. Tumor suppressor LKB1 inhibits activation of signal transducer and activator of transcription 3 (STAT3) by thyroid oncogenic tyrosine kinase rearranged in transformation (RET)/papillary thyroid carcinoma (PTC). Mol Endocrinol. 2007. 21:3039–3049.
20. Nagata D, Takeda R, Sata M, et al. AMP-activated protein kinase inhibits angiotensin II-stimulated vascular smooth muscle cell proliferation. Circulation. 2004. 110:444–451.
21. Kojima K, Motoshima H, Tsutsumi A, et al. Rottlerin activates AMPK possibly through LKB1 in vascular cells and tissues. Biochem Biophys Res Commun. 2008. 376:434–438.
22. Chan AY, Dyck JR. Activation of AMP-activated protein kinase (AMPK) inhibits protein synthesis: a potential strategy to prevent the development of cardiac hypertrophy. Can J Physiol Pharmacol. 2005. 83:24–28.
23. Chan AY, Soltys CL, Young ME, Proud CG, Dyck JR. Activation of AMP-activated protein kinase inhibits protein synthesis associated with hypertrophy in the cardiac myocyte. J Biol Chem. 2004. 279:32771–32779.
24. Motoshima H, Goldstein BJ, Igata M, Araki E. AMPK and cell proliferation: AMPK as a therapeutic target for atherosclerosis and cancer. J Physiol. 2006. 574:63–71.
25. Marignani PA, Kanai F, Carpenter CL. LKB1 associates with Brg1 and is necessary for Brg1-induced growth arrest. J Biol Chem. 2001. 276:32415–32418.