Abstract
Background and Objectives
Vascular perturbation induced by advanced glycation end-products (AGEs) leads to progression of atherosclerosis, plaque instability, and vascular inflammation, which results in a higher risk of neointimal proliferation. Here we investigated the inhibitory effect of alagebrium chloride (ALT-711), a breaker of AGE-based cross links, on neointimal proliferation in a carotid artery balloon injury model in diabetic rats induced by streptozotocin (STZ).
Materials and Methods
Rat aortic vascular smooth muscle cells (RASMCs) were treated with 1-100 µM of alagebrium added 24 hours before the addition of AGEs. This in vivo study was done using 8-week-old male rats that were injected intraperitoneally with 80 mg/kg STZ. Sixteen weeks later, the diabetic rats were treated with 10 mg/kg alagebrium for 4 weeks, after which carotid artery balloon injury was induced. After 4 weeks, the animals were sacrificed for histological analysis.
Results
Proliferation of RASMCs was significantly inhibited in alagebrium-treated cells. Alagebrium dose-dependently inhibited AGE-mediated formation of reactive oxygen species (ROS), extracellular signal-regulated kinase phosphorylation, and cyclooxygenase-2 expression. The cellular mechanisms of AGE-induced connective tissue and extracellular matrix expression were decreased in the alagebrium-treated group. This in vivo study shows that expression of AGE receptors and neointima hyperplasia are significantly suppressed in balloon-injured rats treated with alagebrium.
Although coronary artery restenosis has decreased significantly in the era of drug-eluting stents, it remains a significant clinical problem, one that affects 10% of patients with stents and a significantly higher restenosis rate in diabetics.1-3) Therefore, further improvement in drug eluting stent (DES) delivery and optimal supportive pharmacological therapy may be required to improve clinical outcomes in diabetic patients.4) It is assumed that excessive neointimal formation in diabetes is due to exaggerated inflammatory reactions at the site of injury, increased migration and proliferation of vascular smooth muscle cells, and excessive synthesis of extracellular matrix.2)3)5) However, the pathogenic mechanisms leading to the increased restenosis rate in diabetics are poorly understood.
A recent report showed that long-standing hyperglycemia leads to generation of advanced glycation end-products (AGEs), which are the products of nonenzymatic glycation and oxidation of proteins and lipids, and their accumulation repairs vessel wall formation and vascular perturbations that lead to progression of atherosclerosis and plaque instability.6-11) Moreover, AGEs are reported to have a role in neointimal formation in animal models of arterial stenosis.12-14) In these reports, receptors for AGEs play a key role in neointimal formation after vascular injury, irrespective of diabetes status, and these findings suggest a novel target for minimizing neointimal hyperplasia.13-18) These processes lead to progression of atherosclerosis, plaque instability, and the emergence of clinical events. AGE-mediated cross-link formation in proteins such as collagen may result not only in stiffening of the arteries, but also in increased extracellular matrix formation and increased vascular inflammation, which results in increased risk of neointimal proliferation.7)19-21) AGE accumulation and formation of cross links are associated with increased proinflammatory cytokine levels and increased expression of AGE receptors (RAGEs), resulting in increased vascular inflammation.16)21)22)
In this study, we hypothesized that alagebrium chloride (ALT-711), an AGE cross link breaker, would inhibit neointimal proliferation through the above-mentioned mechanisms in a streptozotocin (STZ)-induced diabetic rat carotid artery balloon injury model.
Healthy male Sprague-Dawley rats that weighed 200-250 g received intraperitoneal injections of STZ (Sigma, St Louis, MO, USA) at a dose of 80 mg/kg. The rats, which develop diabetes, then received long-acting insulin (2 U/day daily) after the STZ injection. Sixteen weeks later, rats were randomized into two study groups: an alagebrium (10 mg/kg) treatment group (n= 4) and a control group (n=4). The alagebrium chloride was mixed with pulverized standard chow to a final alagebrium concentration of 0.015% (wt/wt). Treatment of rats with drug mixed with chow was continued for 4 weeks. Then, carotid artery balloon injury was induced. After 4 weeks, animals were sacrificed for histological analysis of the injured carotid artery.
Rat aortic vascular smooth muscle cells (RASMCs) were isolated as previously described.23) RASMCs were cultured in DMEM supplemented with 10% fetal bovine serum, 100 IU/mL penicillin, and 100 µg/mL streptomycin in 75-cm2 flasks at 37℃ in a humidified atmosphere of 95% air and 5% CO2 (Therno Scientific Inc., Asheville, NC, USA).
RASMCs were plated in triplicate wells of 96-well plates at a density of 1×104 cells per well. Pretreatment with alagebrium was done 3 hours prior to exposure to AGEs (50 µg/mL) for 3 hours. After treatment of cells, 3-(4,5-Dimethylthiazol-2-yl)-2,5-diphenyltetrazolium bromide (MTT) solution (Sigma, USA) was added to each well to a final concentration of 0.5 mg/mL and cells were incubated at 37℃ for 3 hours to allow MTT reduction. The formazan crystals were dissolved by adding dimethylsulfoxide (DMSO) and absorbance was measured at 570 nm with a spectrophotometer.
To measure reactive oxygen species (ROS) generation, RASMCs were labeled with 2', 7'-dichlorodihydrofluorescein diacetate (H2DCFDA; of 5 mM, Invitrogen, Carlsbad, CA, USA). Quiescent cells were treated with alagebrium and/or AGEs before labeling with H2DCFDA. Labeled cells were incubated at 37℃ in a cell culture incubator for 10 minutes. Cells were then analyzed by fluorescence using an excitation wavelength of 480 nm and emission light at 535 nm and a confocal microscope (Carl Zeiss, Inc., Thornwood, NY, USA).
Expression levels of various proteins were analyzed by reverse transcription polymerase chain reaction (RT-PCR). Total ribonucleic acid (RNA) was prepared by the Ultraspect™-II RNA method (Biotecx Laboratories, Inc., Houston, TX, USA) and single-stranded complementary deoxyribonucleic acid was synthesized from isolated total RNA by Avian Myeloblastosis virus (AMV) reverse transcriptase. Twenty µL of reverse transcription reaction mixture containing 1 g of total RNA, 1X reverse transcription buffer (10 mM Tris-HCl, pH 9.0, 50 mM KCl, 0.1% Triton X-100), 1 mM deoxynucleoside triphosphates (dNTPs) 0.5 unit of RNase inhibitor, 0.5 g of oligo (dT)15 and 15 units of AMV reverse transcriptase were incubated at 42℃ for 15 minutes, heated to 99℃ for 5 minutes, and then incubated at 4℃ for 5 minutes. PCRs were done for 35 cycles with 3' and 5' primers based on the sequences of various genes. Primers were as follows: RAGE: 5'-GGCCTTCCTCGGCGCAGACC-3', 5'-TAGATGCCCTCATCCTCATGC-3' (260 bp); COX-2: 5'-TCCAATCGCTGTACAAGCAG-3', 5'-T CCCCAAAGATAGCATCTGC-3' (230 bp); Collagen type III: 5'-AGATGCTGGTGCTGAGAAG-3', 5'-TGGAAAGA AGTCTGAGGAAGG-3' (312 bp); Fibronectin: 5'-GTGAAGAACG-AGGAG-GATGT-G-3', 5'-GTGATGGCGGATGATGTAGC-3' (300 bp); Connective tissue growth factor (CTGF): 5'-AAGAAGACTCAGCCAGACC-3', 5'-AGAGGAGGAGCACCAAGG-3' (235 bp). Glyceraldehyde phosphate dehydrogenase (GAPDH): 5'-ACCACAGTCCATGCCATCAC-3', 5'-TCCACCACCCTGTTGCTGTA-3' (450 bp). The signal intensity of the amplification product was normalized to its respective GAPDH signal intensity.
Cells were washed once in phosphate buffered saline and lysed in a lysis buffer (Cell Signaling Technology, Inc., Danvers, MA, USA) containing 20 mM Tris (pH 7.5), 150 mM NaCl, 1 mM Na2 ethylenediaminetetraacetic acid, 1 mM ethyleneglycoltetraacetic acid, 1% Triton, 2.5 mM sodium pyrophosphate, 1 mM β-glycerophosphate, 1 mM Na3VO4, 1 mg/mL leupeptin and 1 mM PMSF. Protein concentrations were determined using the Bradford protein assay kit (BioRad, Hercules, CA, USA). Proteins were separated in a 12% SDS-polyacrylamide gel and transferred to polyvinyliclene membrane (Millipore Co., Billerica, MA, USA). After blocking the membrane with Tris-buffered saline-Tween 20 (TBS-T, 0.1% Tween 20) containing 5% non-fat dried milk for 1 hour at room temperature, membranes were washed twice with TBS-T and incubated with primary antibodies for 1 hour at room temperature or for overnight at 4℃. The following primary antibodies were used: rabbit anti-extracellular signal-regulated kinase (ERK), mouse anti-phospho ERK (Santa Cruz Biotechnology, USA). The mem-branes were washed three times with TBS-T for 10 minutes, and then incubated for 1 hour at room temperature with horseradish peroxidase-conjugated secondary antibodies. After extensive washing, the bands were detected by enhanced chemiluminescence reagent (Santa Cruz Biotechnology, Santa Cruz, CA, USA). The band intensities were quantified using a Photo-Image System (Molecular Dynamics, Sweden).
Animals were anesthetized with intraperitoneal ketamine (80 mg/kg). After anesthesia, the left carotid artery was exposed by cervical midline excision. The proximal portion of the left common carotid artery and the origin of the left internal carotid artery were temporarily ligated with surgical threads to prevent excessive bleeding during the balloon catheter insertion. Part of the external carotid artery wall was excised after which a 2-Fr Fogarty balloon catheter (Baxter Healthcare Corp., City, State, Deerfield, IL, USA) was inserted. After the insertion of the balloon catheter, the temporarily-ligated proximal left common carotid artery was untied and the catheter was advanced towards the direction of the ascending aorta. The balloon was inflated just enough to develop a mild resistance against the arterial wall (0.2 mL). The expanded balloon catheter was than pulled back to inflict uniform injury along the entire common carotid artery wall. This procedure was repeated 3 times for complete denudation of the intima, after which the catheter was removed and the proximal external carotid artery was ligated. Sham operations were performed on the right common carotid arteries.
The vessels were perfusion-fixed overnight with 10% (v/v) neutral-buffered formaldehyde, transversely sectioned into serial thick sections, and embedded in paraffin using routine methods. Sections that were 2 µm thick were mounted on gelatin-coated glass slides to ensure that different stains could be used on successive sections of tissue cut through the areas of balloon injury. After deparaffinization and rehydration, the sections were analyzed with mouse anti-RAGE (Santa Cruz Biotechnology). Expression of RAGE was visualized by adding 3,3-diaminobenzidine (Vector Laboratories, Burlingame, CA, USA) for 5 minutes. All images were made using virtual microscopy (Universal Imaging Corp., USA). Neointimal areas were visualized by Hematoxylin and eosin staining and quantified using Image J Program (NIH, USA).
Data are presented as mean±SEM of more than three separate experiments each done in triplicate. Where results of blots and RT-PCR are shown, a representative experiment is depicted. Comparisons between multiple groups were done with one-way analysis of variance and Bonferroni's test. Statistical significance was defined as *p<0.05 and **p<0.01.
AGE-treatme nt of cells (50 µg/mL) caused a marked increase (about 30%) in the number of cells. Alagebrium treatment along with AGE inhibited the proliferation of RASMCs. Pretreatments using 1 to 100 µM alagebrium significantly inhibited RASMC proliferation in a dose-dependent manner (Fig. 1). This demonstrated the antiproliferative effect of alagebrium on AGE-stimulated proliferation of RASMCs.
Following a 3 hours incubation of RASMCs with AGEs, ROS production was dose dependently increased, indicating that AGEs could be a growth stimulator of RASMCs. There was a significant increase in ROS formation in cells treated with 50 to 100 µg/mL AGEs (Fig. 2A). In order to determine whether alagebrium modifies the effect of AGEs on ROS production by RASMCs, cells were pretreated with various concentrations of alagebrium for 30 minutes prior to exposure to AGEs (50 µg/mL). Alagebrium-treatment of RASMCs reduced the formation of ROS in a dose-dependent manner (Fig. 2B).
Cells exposed to 1 and 10 µM of alagebrium were treated with 50 µg/mL of AGEs. Mitogen-activated protein kinase (MAPK) signaling was inhibited-by 35% and 42%, respectively-by pretreatment with 1 and 10 µM of alagebrium (Fig. 3A). To determine the relationship between ROS production and ERK activation in RASMCs, COX-2 expression was determined by RT-PCR. The results showed that COX-2 mesenger ribonucleic acid (mRNA) expression increased by 2.15-fold after incubation with 50 µg/mL of AGEs. However, pretreatment with alagebrium resulted in significant inhibition (up to 50%) of the expression of COX-2 in AGE-stimulated cells in the 10 µM alagebrium treatment group (Fig. 3B).
As illustrated in Fig. 4, AGE (50 µg/mL) treatment for 24 hours markedly increased mRNA expression for collagen type III, fibronectin, and CTGF. However, alagebrium-treated cells showed dose-dependent abolition of collagen type III, fibronectin and CTGF mRNA levels at each dose of alagebrium. The mRNA expression level of collagen type III decreased significantly in the alagebrium-treated group compared to AGE alone (Fig. 4A).
Also, the expression of fibronectin was significantly diminished in the alagebrium treatment group by about 27% and 47% each, compared to the AGE treatment group (Fig. 4B). Finally, CTGF expression was down-regulated by approximately 12.5% and 46%, respectively, after pretreatment with 1 µM and 10 µM of alagebrium (Fig. 4C).
The carotid balloon injury rat model was associated with a markedly increased expression of RAGE, which was significantly attenuated in alagebrium-treated rats (Fig. 5A). Also, analysis of RAGE expression in neointimal tissue, as determined by RT-PCR, showed that alagebrium (1 and 10 µM) dose-dependently inhibited the expression of RAGE (by approximately 17% and 84%, respectively) (Fig. 5B).
Alagebrium was administered daily for 4 weeks prior to balloon injury using the carotid artery balloon injury diabetic rat model. Withdrawal of the inflated balloon resulted in vascular injury leading to neointima formation. Four weeks after injury, the inner vessel surface was covered with one or several layers of ovoid, irregularly-shaped cells forming the neointima. Fig. 6 shows that neointimal formation in the alagebrium treatment group after balloon injury was significantly lower than in controls, in STZ-treated rat given alagebrium after balloon injury there was no further neointimal growth. At this time, the neointimal area in STZ-treated rat given alagebrium was reduced significantly by about 69% compared with control (Fig. 6B).
Our study demonstrates that alagebrium chloride inhibits neointimal proliferation in a STZ-induced diabetic rat carotid artery balloon injury model. To our knowledge, this is the first report to show that alagebrium can directly inhibit the pro-atherosclerotic effect of AGE on RASMCs. These effects of alagebrium were mediated by inhibition of ERK signaling and down-regulation of COX-2 mRNA expression via reduction of intracellular ROS levels that had been induced by AGE. Furthermore, we found that the anti-proliferative effect of alagebrium resulted from inhibition of RAGE expression.
Macrovascular complications develop in >50% of the diabetic population and account for 50-60% of the mortality in this high-risk group.1) Dyslipidemia, hypertension, and hyperglycemia all play roles in the development of diabetes-associated atherosclerosis, although the specific contributions from each of these independent risk factors remain controversial. Previous studies that used exogenous administration of AGEs to mimic diabetic serum concentrations indicated that AGEs can induce atherosclerosis.9-11) Furthermore, AGEs interact with endothelial cells to induce the expression of atherogenic adhesion molecules implicated in atherogenesis.
Studies in experimental obese Zucker rats, a model of insulin resistance induced by the AGE formation inhibitor pyridoxamine, have shown marked attenuation of the characteristic hyperlipidemia, reduced AGEs, and hypertension, indicating interactions between AGEs and lipids.22) One of the seminal in vivo studies performed in experimental diabetes-associated atherosclerosis demonstrated attenuation of plaque formation by the soluble receptor for AGEs (sRAGE).21)
More recently, therapeutic approaches have involved the use of a thiazolium derivative, alagebrium, a compound that suggested to be capable of breaking AGE cross-links, and thereby removing preformed AGEs.6-8) Alagebrium has proved successful as an intervention for established diabetic microvascular complications, and has also been reported to improve vascular compliance in aging humans. Other studies of diabetics have shown that alagebrium attenuates both cardiac and renal accumulation of AGEs in association with benefits for patients with renal and cardiac injury.11)15-17) AGE formation may contribute to the progression of atherosclerosis, particularly in those with diabetes. AGEs can pass through cell membranes via RAGE and stimulate MAPKs to cause proliferation. The present study shows the inhibitory effects of alagebrium on RASMCs proliferation (Fig. 1) and ROS production (Fig. 2). The mechanism of increased cell survival and proliferation may involve phosphorylation of ERKs (42 and 44 kDa). Our results represent the inhibitory effects of alagebrium on ERK activation and COX-2 inhibition (Fig. 3). The activation of ERKs plays an important role in gene regulation, and ERK is one of several dual-specificity kinases in the MAPK family. Thus, the inhibition of ERK may a main mechanism in the anti proliferative effect of alagebrium. Because COX-2 expression is associated with ROS production and ERK activation, the inhibition of COX-2 expression with the subsequent attenuation of oxidative stress and ERK activation may also be an important mechanism to explain the efficacy of alagebrium on attenuating neointimal hyperplasia. Alagebrium also inhibited the increase in formation of connective tissue and extracellular matrix (Fig. 4). Immunohistochemical staining of newly formed neointima showed a significant reduction in RAGE expression (Fig. 5) with significant suppression of neointima formation in alagebrium-treated balloon injured diabetic rats (Fig. 6). The breakdown of AGEs in the vascular wall may reduce the expression of RAGE, which is known to be positively regulated, which may result in reduced activation of ERK and a decrease in oxidative stress.
In conclusion, alagebrium inhibits neointimal hyperplasia after carotid balloon injury because it blocks intracellular ROS synthesis and ERK activation, resulting in inhibition of RASMCs proliferation. The inhibitory effect of alagebrium on neointimal formation may also be mediated by reduction of ECM production.
Figures and Tables
Fig. 1
Anti-proliferative effect of alagebrium in AGE-stimulated RASMCs. AGE promotes proliferation of RASMCs, but alagebrium dose-dependently inhibits proliferation of RASMCs (*p<0.05 vs. control; †p<0.01 vs. AGE-treated group). AGE: advanced glycation end-product, RASMCs: rat aortic vascular smooth muscle cells.
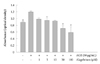
Fig. 2
Effect of AGE and alagebrium on the formation of intracellular ROS in RASMCs. AGEs dose-dependently stimulated growth of RASMCs. Alagebrium reduced the formation of reactive oxygen species (*p<0.05 vs. CTL; vs. AGE-treated group). AGE: advanced glycation end-product, ROS: reactive oxygen species, RASMCs: rat aortic vascular smooth muscle cells, DCF: 2'-7'-dichlorofluorescin, CTL: control.
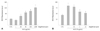
Fig. 3
Effect of alagebrium on phosphorylation of ERK and mRNA level of COX-2. A: MAPK activation was seen within 30 minutes following 50 µg/mL of AGEs. Alagebrium significantly inhibited expression of COX-2 mRNA in AGE-stimulated RASMCs. B: these signaling activations were significantly inhibited by pretreatment with 1 and 10 µM of alagebrium (*p<0.05 vs. AGE-treated group). ERK: extracellular signal-regulated kinase, COX-2: cyclooxygenase-2, mRNA: messenger ribonucleic acid, MAPK: mitogen-activated protein kinase, AGE: advanced glycation end-product, RASMCs: rat aortic vascular smooth muscle cells.
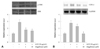
Fig. 4
Effects of alagebrium on expression of CTGF and ECM in AGE-stimulated RASMCs. AGE (50 µg/mL) treatment for 24 hours markedly increased messenger ribonucleic acid expression of collagen type III (A), fibronectin (B) and CTGF (C) in RASMCs. Alagebrium (1 and 10 µM) dose-dependently abolished this effect (*p<0.05 vs. AGE-treated group, **p<0.01 vs. AGE-treated group). CTGF: connective tissue growth factor, ECM: extra-cellular matrix, AGE: advanced glycation end-products, RASMCs: rat aortic vascular smooth muscle cells.
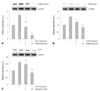
Fig. 5
Effect of alagebrium on the expression of RAGE in balloon-injured rats. A: RAGE expression was significantly decreased by alagebrium treatment in vivo, which was confirmed by immunohistochemistry. All sections were observed by virtual microscopy (magnification: left, ×200; right, ×400). B: AGE group increased expression of messenger ribonucleic acid for RAGE. This effect was significantly attenuated by alagebrium (*p<0.05 vs. AGE-treated group). RAGE: advanced glycation end-product receptor.
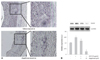
Fig. 6
The effect of alagebrium on extracellular matrix and neointima formation at the site of balloon-injury in STZ-treated rats. A: neointima hyperplasia was decreased by alagebrium treatment in diabetic rats (H&E stain). B: extracellular matrix in STZ-treated rats treated with alagebrium was reduced to below control levels (*p<0.05 vs. Control). STZ: streptozotocin.
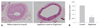
Acknowledgments
This study was made possible by the generous donation of Alagebrium by Alteon (Now Synvista).
References
1. Kannel WB, McGee DL. Diabetes and cardiovascular disease: the Framingham Study. Circulation. 1979. 59:8–13.
2. Ha JK, Han DC, Hwang KW, et al. Metabolic syndrome and risk of in-stent restenosis: clinical outcomes in patients undergoing percutaneous coronary intervention. Korean Circ J. 2007. 37:567–573.
3. Park JS, Seok JH, Hong GR, Shin DG, Kim YJ, Shim BS. Types of in-stent restenosis and predictive factors for diffuse type in-stent restenosis. Korean Circ J. 2001. 31:1135–1141.
4. Nielsen TT, Bøtker HE. Percutaneous coronary intervention in diabetic patients: a problem? Horm Metab Res. 2005. 37:Suppl 1. 83–89.
5. Serruys PW, de Jaegere P, Kiemeneij F, et al. A comparison of balloon-expandable-stent implantation with balloon angioplasty in patients with coronary artery disease. N Engl J Med. 1994. 331:489–495.
6. Vaitkevicius PV, Lane M, Spurgeon H, et al. A cross-link breaker has sustained effects on arterial and ventricular properties in older rhesus monkeys. Proc Natl Acad Sci U S A. 2001. 98:1171–1175.
7. Candido R, Forbes JM, Thomas MC, et al. A breaker of advanced glycation end products attenuates diabetes induced myocardial structural changes. Circ Res. 2003. 92:785–792.
8. Little WC, Zile MR, Kitzman DW, Hundley WG, O'Brien TX, Degroof RC. The effect of alagebrium chloride(ALT-711), a novel glucose cross link breaker, in the treatment of elderly patients with diastolic heart failure. J Card Fail. 2005. 11:191–195.
9. Cooper ME. Impotance of advanced glycation end products in diabetes associated cardiovascular and renal disease. Am J Hypertens. 2004. 17:31S–38S.
10. Yan SF, Ramasamy R, Naka Y, Schmidt AM. Glycation, inflammation, and RAGE: a scaffold for the macrovascular complications of diabetes and beyond. Circ Res. 2003. 93:1159–1169.
11. Kass DA, Shapiro EP, Kaawaguchi M, et al. Improved arterial compliance by a novel advanced glycation end product crosslink breakers. Circulation. 2001. 104:1464–1470.
12. Wolffenbuttel BH, Boulanger CM, Crijns FR, et al. Breakers of advanced glycation end products restore large artery properties in experimental diabetes. Proc Natl Acad Sci U S A. 1998. 95:4630–4634.
13. Ettenson DS, Gotlieb AI. Centrosomes, microtubules, and microfilaments in the reendothelialization and remodeling of double-sided in vitro wound. Lab Invest. 1992. 66:722–733.
14. Villa AE, Guzman LA, Chen W, Golomb G, Levy RJ, Topol EJ. Local delivery of dexamethasone for prevention of neointimal proliferation in a rat model of balloon angioplasty. J Clin Invest. 1994. 93:1243–1249.
15. Clowes AW, Reidy MA, Clowes MM. Kinetics of cellular proliferation after arterial injury: I. smooth muscle growth in the absence of endothelium. Lab Invest. 1983. 49:327–333.
16. Sakaguchi T, Yan SF, Yan SD, et al. Central role of RAGE-dependent neointimal expansion in arterial restenosis. J Clin Invest. 2003. 111:959–972.
17. Naka Y, Bucciarelli LG, Wendt T, et al. RAGE axis: animal models and novel insights into the vascular complications of diabetes. Arterioscler Thromb Vasc Biol. 2004. 24:1342–1349.
18. Shim CY, Park S, Yoon SJ, et al. Association of RAGE gene polymorphisms with in-stent restenosis in non-diabetic Korean population. Cardiology. 2007. 107:261–268.
19. Susic D, Varagic J, Ahn J, Frohlich ED. Crosslink breakers: a new approach to cardiovascular therapy. Curr Opin Cardiol. 2004. 19:336–340.
20. Forbes JM, Yee LT, Thallas V, et al. Advanced glycation end product interventions reduce diabetes-accelerated atherosclerosis. Diabetes. 2004. 53:1813–1823.
21. Zhou Z, Wang K, Penn MS, et al. Receptor for AGE (RAGE) mediates neointimal formation in response to arterial injury. Circulation. 2003. 107:2238–2243.
22. Yue TL, Bao W, Gu JL, et al. Rosiglitazone treatment in Zucker diabetic Fatty rats is associated with ameliorated cardiac insulin resistance and protection from ischemia/reperfusion-induced myocardial injury. Diabetes. 2005. 54:554–562.
23. Park S, Lim S, Chang W, et al. The inhibition of insulin-stimulated proliferation of vascular smooth muscle cells by rosiglitazone is mediated by the Akt-mTOR-P70S6K pathway. Yonsei Med J. 2008. 49:592–600.