Abstract
Background and Objectives
Subjects and Methods
Results
Figures and Tables
Fig. 1
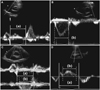
Fig. 2
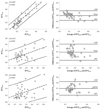
Fig. 3
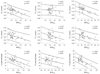
Table 1
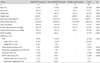
Data are presented as the means±SD or as numbers (%). *p were calculated using an independent t-test or Chi-squared test between groups A and B. Fisher,s exact tests were used when applicable. One-way analysis of variance with post-hoc analysis using the Bonferroni correction method was also used for comparison of the parameters between three groups, †p<0.05 compared with the healthy control group in post-hoc analysis, ‡Patients that developed chronic pulmonary regurgitation after surgical treatment of tetralogy of Fallot (TOF). RVSP: right ventricular systolic pressure, ASD: atrial septal defect, PAH: pulmonary arterial hypertension, RVOT: right ventricular outflow tract
Table 2
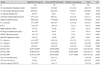
Data are presented as the means±SD or as numbers (%). *p calculated using the independent t-test or one-way analysis of variance with posthoc analysis by the Bonferroni correction method, †p<0.05 compared with the healthy control group, ‡p<0.05 compared with group B. RVSP: right ventricular systolic pressure, LV: left ventricle, E: early diastolic mitral inflow velocity, E'MV: early diastolic mitral annular velocity, E/E'MV: E to E'MV ratio, RV: right ventricle, S'TV: peak systolic tricuspid annular velocity, E'TV: peak early diastolic tricuspid annular velocity, A'TV: peak late diastolic tricuspid annular velocity, RTX: RV Tei index, CFD: conventional flow Doppler, TDE: tissue Doppler echocardiography, DPD: dual pulsed-wave Doppler, VO2 max: peak oxygen consumption rate, METS: metabolic equivalents
Table 3
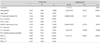
N=41, dependent variable as METS. R2=0.79, Adjusted R2=0.75, standard error of the estimate=1.32. The backward stepwise approach was used to select best model fits to predict the METS. *RTXCFD and RTXTDE were excluded for the multiple regression model in this table (see text for details). LV: left ventricle, E'MV: early diastolic mitral annular velocity, E/E'MV: E to E'MV ratio, RV: right ventricle, S'TV: systolic tricuspid annular velocity, E'TV: early diastolic tricuspid annular velocity, RTX: RV Tei index, DPD: dual pulsed-wave Doppler, CFD: conventional flow Doppler, TDE: tissue Doppler echocardiography