Abstract
Background and Objectives
Angiopoietin-1 (Ang1) is a regulator of blood vessel growth and maturation, and prevents radiation-induced or serum deprivation-induced apoptosis. Phosphatase and tensin homologue deleted from chromosome ten (PTEN), a well-known tumor suppressor, regulates cell cycle arrest and apoptosis. Hypoxia induces apoptosis by increasing the expression of PTEN. We hypothesized that Ang1 may regulate PTEN expression and, thus, reduce endothelial apoptosis under hypoxia in vitro and in vivo.
Materials and Methods
In vitro, human umbilical vein endothelial cells (HUVECs) were treated with Ang1, and signaling pathways were investigated. In vivo, eight-week-old C57BL/6 mice were used for a hind limb ischemia model. Ang1 or normal saline was intramusculary injected. Blood flow was evaluated by a laser Doppler perfusion analyzer and tissue histology.
Results
The expression of PTEN was markedly upregulated in HUVECs after hypoxic stimulation, whereas Ang1
suppressed PTEN expression. Tie2-Fc, a soluble form of Tie2 (sTie2) that blocks Ang1, reversed the Ang1 effect on PTEN reduction under hypoxia. Ang1 inhibited the nuclear translocation of nuclear transcription factor-kB (NF-kB), a binding factor for the PTEN promoter and Foxo1. Hypoxia-induced p27 expression and apoptosis were also suppressed by Ang1. In the mouse hind limb ischemia model, we observed a high capillary density, numerous proliferating cells and diminished cell death in skeletal muscle tissue in the Ang1 injected group.
Conclusion
Ang1 enhanced endothelial cell survival by reducing apoptosis via PTEN down-regulation in HUVECs under hypoxia. Local injection of Ang1 significantly reduced apoptotic cells in vivo, and prevented limb loss for ischemic hind limb mice. Thus, Ang1 may be an effective therapeutic for protection from ischemic-endothelial cell injury.
Phosphatase and tensin homologue deleted from chromosome ten (PTEN), a tumor suppressor protein, plays an important role in cell cycle arrest and apoptosis. 1) Mutations or deletions of the PTEN gene are involved in a variety of cancers, including glioblastoma, melanomas and advanced prostate adenocarcinomas.1)2) The primary function of PTEN is as a lipid phosphatase. PTEN hydrolyzes phosphatidylinositol 3,4,5-triphosphate {PI(3,4,5)P3}, a product of phosphatidylinositol 3-kinase (PI3K), to phosphatidylinositol 4,5 bisphosphate, thereby suppressing the PIP3-mediated Akt activation and survival signaling pathway.3)
The over-expression of PTEN has been shown to induce apoptosis in ovarian cancer.4) PTEN suppresses tumorigenicity and induces cell cycle arrest by the down-regulation of PI3K/Akt signaling pathway in gliomblastoma cells, and a Cyclin Dependent Kinases (CDK) inhibitor, p27, is a target of PTEN.2) Inhibition of PTEN activity suppresses neuron apoptosis and, thus, shows neuroprotective effects.5) Although molecular roles for PTEN have been widely studied in cancer cells, little is known regarding its effects in endothelial apoptosis.
Angiopoietin-1 (Ang1) plays essential roles in regulating vascular growth, development, maturation and vascular permeability.6) During developmental stages, Ang1 and vascular endothelial growth factor (VEGF) collaborate to regulate vascular formation.7) VEGF initiates the proliferation and migration of endothelial cells and promotes the formation of new blood vessels.6-8) In contrast, Ang1 is required for the stabilization of contacts between endothelial and surrounding mural cells to form mature vessels.6)9) Ang1 binds to the endothelial-specific Tie2 receptor tyrosine kinase and has a role in the reduction of vascular permeability.6)
Pathological conditions, including ischemia, tumors and inflammation, cause damage to the endothelium by inducing endothelial death and increasing the permeability of the microvasculature.10) Ang1 has an anti-apoptotic effect as well as anti-permeability activity. Ang1 inhibits radiation-induced endothelial cell apoptosis via Tie2 receptor binding and PI3K activation.11) Ang1 mediates cell survival in cardiac and skeletal myocytes through integrins, and then activates the Akt and mitogen activated protein kinase (MAPK) survival pathway.12)
In this study, we demonstrated that Ang1 suppressed PTEN expression, and inhibited the nuclear translocation of forkhead transcription factor Foxo1 and p27 activity by reducing nuclear transcription factor-kB (NF-kB) activity. This resulted in a reduction of endothelial apoptosis in vitro. In a mouse hind limb ischemia model, Ang1 injection reduced apoptosis, improved blood flow, and promoted prominent limb salvage. Therefore, Ang1 has a protective role against endothelial and non-endothelial apoptosis in vivo.
Human umbilical vein endothelial cells (HUVECs) (Clonetics™) were plated on 1.5% gelatin (Sigma)-coated dishes and cultured in endothelial basal medium (EBM) or endothelial growth medium (EGM) (Clonetics) supplemented with 5% fetal bovine serum at 37℃. Four to six passage cells were used in this study.
For a recombinant Ang1 protein, we used cartilage oligomeric matrix protein-angiopoietin-1 (COMP-Ang-1), which is a potent Ang1 variant.11) Tie2/FC (R&D Systems), a soluble form of Tie2, was used at 10 µg/mL and added to the cells 30 minutes before exposure to hypoxic conditions. Hypoxic conditions were achieved using BBL GasPac (Becton Dickinson), which catalytically reduces oxygen levels to <1% oxygen within 15 minutes.
HUVECs were washed with cold phosphate-buffered saline (PBS) and lysed in protein lysis buffer containing protease inhibitors (50 mM Tris-pH 7.2, 250 mM NaCl, 1% NP40, 0.05% SDS, 2 mM EDTA, 0.5% Deoxycholic acid, 10 mM beta-glycerol phosphate, 1 mM sodium orthovanadate, 100 mM NaF) and protease inhibitor cocktail (Roche). For nuclear protein isolation, cells were harvested and lysed in low salt buffer (10 mM HEPES (pH7.9), 1.5 mM MgCl2, 0.02 M KCl, 0.2 mM EDTA, 0.5 mM DTT, 0.2 mM PMSF). Cell pellets were resuspended in high salt buffer (10 mM HEPES, pH 7.9, 1.5 mM MgCl2, 1.2 M KCl, 0.2 mM EDTA, 0.5 mM DTT, 0.2 mM PMSF), centrifuged and supernatants were collected to be used as nuclear extracts.
Protein concentration was determined with BCA protein assay kit (Pierce). Total protein (15 µg) or nuclear protein was separated on SDS-PAGE, transferred to a PVDF membrane (Millipore) and the blot was used for immunostaining with specific primary antibodies overnight at 4℃. Immuno-reactions were detected using an ECL kit (Amersham Bioscience).13) Antibodies were used against: PTEN, pp38, t-p38, p-AKT, t-AKT, Foxo1, p-Foxo1, Foxo3a (Cell signaling); IkB (Santa Cruz); α-tubulin (Calbiochem).
Total cellular RNA was isolated from HUVECs by Trizol (Invitrogen) according to the manufacturer's instructions. cDNA was synthesized with an AMLV-RT system (Promega). Oligonucleotide primers for PCR were designed as follows: PTEN, forward, 5'-GATTTCTATGGGGAAGTAAGGA-3'; reverse, 5'-GATACGGCTGAGGGAACTC-3'; p27, forward, 5'-ATGTCAAACGTGCGAGTGTCT-3'; reverse, 5'-TTACGTTTGACGTCTTCTGA-3'; GAPDH, forward, 5'-CCTCTGGAAAGCTGTGGCGT-3'; reverse, 5'-TTGGAGGCCATGTGGCCAT-3'.
A chromatin immunoprecipitation (ChIP) assay was performed with a commercial kit (Upstate Biotechnology) following manufacturer's instructions. Anti-Foxo1 antibody was used for immunoprecipitation of the DNA fragments. The DNA fragments were analyzed by PCR with primers specific for p27.
Apoptosis was evaluated by fluorescence-activated cell sorter (FACS). HUVECs were cultured with or without Ang1 under hypoxia. Cells were harvested at indicated time points, and fixed at 40 hours for analysis of apoptosis. Flow cytometric analysis was performed on a FACStar Plus (BD Biosciences). Histograms of DNA contents were analyzed using Modfit LT software (Verity Software) to characterize population fractions in each phase of the cell cycle. For trypan blue exclusion assay, HUVECs were harvested at indicated time points. Cell suspensions were mixed with an equal volume of trypan blue and the numbers of stained and unstained cells were counted in 3 randomly selected high-power fields.
All animal experiments were performed with the approval of the Institutional Animal Care and Use Committee of Seoul National University Hospital. Male C57 BL/6 (8 weeks old) mice were anesthetized with a 4:1 mixture of ketamine & xylazine (50 mg/kg) by intraperitoneal injection. To induce muscle ischemia, a unilateral femoral artery was removed as previously described.14) Mice in the angiopoietin group (n=20) were intramuscularly injected with recombinant Ang1 (total 100 µg) at 3 times in sequence: 30 minutes before, soon after, and 30 minutes after the surgery. Mice in the control group (n=20) were treated with normal saline by the same procedure. For evaluation of Ang1 on the therapeutic blood flow recovery, laser Doppler perfusion imaging (LDPI) analysis was performed. Serial blood flow measurements were recorded using an LDPI analyzer (Moor Instruments).
HUVECs were washed with PBS, fixed with 100% methanol for 20 minutes at -20℃, and washed 3 more times with 0.05% PBS-T. After blocking with 1% of BSA/PBS for 1 hour, cells were labeled with anti-NF-kB (Santa Cruz) overnight at 4℃. After 3 washes with 0.05% PBS-T, cells were incubated with goat anti-mouse PE for 1 hour at room temperature. The nuclei were stained with DAPI (1 µg/mL in PBS) for 10 minutes, washed and mounted using fluorescent mounting medium (DAKO Cytomation). To analyze tissues of hind limb ischemia mice, the calf muscle was excised, rinsed with PBS, fixed with 4% PFA and embedded in OCT compound.
Ten µm-thick histological sections were prepared from snap-frozen tissue samples. Sections were washed, and incubated with FITC-labeled BS-1 lectin (Sigma) or platelet/endothelial cell adhesion molecule-1 (PECAM-1) (Santa Cruz) for capillary density, and rabbit anti-Ki67 (Abcam) for proliferating cells. Tissues were washed and stained with goat anti-rabbit TRITC or anti-rabbit FITC (Dako). Fluorescent images were obtained with a fluorescence microscope (Olympus IX71, Japan). Ki67/BS-1 lectin positive cells were counted in 10 different microscopic fields of at least 3 different sections for each animal.
To evaluate apoptosis, the terminal deoxynucleotidyltransferase-mediated dUTP nick-end labeling (TUNEL) assay was performed.15) TUNEL-positive cells were counted in 10 different microscopic fields of at least 3 different sections from each animal.
It has been reported that the expression of Ang1 is decreased under hypoxia.,16) and that Ang1 suppresses radiation-induced or serum deprivation-induced apoptosis.11) So, we first tested the possibility that Ang-1 might regulate the expression of PTEN, which is a tumor suppressor and hypoxia-responsive molecule, under hypoxia in HUVECs. Hypoxic conditions significantly induced cellular apoptosis (p<0.01) in a time-dependent manner (Fig. 1A). After 36 hours exposure to hypoxic conditions, apoptotic cells were increased about 3 times compared to normoxic conditions as determined by trypan blue exclusion assay.
The expression of PTEN was increased under hypoxia (Fig. 1B), and showed maximal alterations at 12 hours and 24 hours. However, Ang1 (200 ng/mL) markedly reduced the expression of PTEN that was induced by hypoxic conditions (Fig. 1B). In the presence of Ang1, PTEN mRNA and protein levels were significantly decreased after 24 hours under hypoxia (Fig. 1C and D). Tie2/FC, a soluble Tie2 (sTie2) that acts as an Ang1 blocker, significantly reversed the Ang1 effect on PTEN expression (Fig. 1C and D). This indicates that Ang1 reduced the hypoxia-induced PTEN expression in HUVECs.
Because PTEN expression is regulated by NF-kB, and because p38 has been shown to regulate NF-kB activity under hypoxia/reoxygenation,17)18) we tested if Ang1 regulated p38 and NF-kB signaling under hypoxic conditions. We found that p38 phosphorylation was strongly increased under hypoxia, while Ang1 clearly down regulated hypoxia-induced pp38 (Fig. 2A). Interestingly, treatment with sTie2 reversed the effect of Ang1 on p38-phosphorylation. To allow NF-kB activation, NF-kB must be dissociated from its inhibitor, IkB, and translocated to the nucleus.19) In contrast to pp38 protein, IkB was decreased by hypoxia, but Ang1 increased IkB protein expression (Fig. 2A). IkB expression was inversely regulated by sTie2 pre-treatment under hypoxia (Fig. 2A).
In order to confirm the NF-kB activity, we performed immunofluorescence staining for NF-kB after hypoxic stimulation for 24 hours (Fig. 2B). NF-kB stained red and the nucleus stained blue. NF-kB was translocated to the nucleus under hypoxia, indicating that NF-kB was activated. When HUVECs were treated with Ang1, NF-kB staining was observed in the cytosol under hypoxic conditions. After sTie2 treatment, NF-kB was detected in the nucleus, even in the presence of Ang1 (Fig. 2B).
Forkhead transcription factors, including Foxo1 and Foxo3a, are downstream of PTEN, and are important regulators of PTEN-mediated apoptosis.20) We investigated if Ang1 affected Foxo1 and Foxo3a. As shown in Fig. 3A, nuclear protein was isolated and analyzed for protein levels of Foxo1 and Foxo3a. Foxo1 was strongly increased under hypoxic conditions, but Ang1 decreased the Foxo1 protein level under hypoxia (Fig. 3A). Total Foxo1 protein in whole cell lysates was not changed (Fig. 3B). Because p-Foxo1 is an inactive form of Foxo1, the trend for the level of p-Foxo1 in whole cell lysates was opposite to that for nuclear Foxo1 protein. That is, it was decreased under hypoxia, but increased in the presence of Ang1. sTie2 reversed the effect of Ang1 on p-Foxo expression (Fig. 3B). It is well known that p-Akt is downstream in the pathway of Ang1. Therefore, the increased level of pAkt indicated that HUVECs responded well to Ang1 (Fig. 3B).
Next, we checked p27expression, the downstream effector of PTEN and Foxo1.20) The expression of p27 was increased by hypoxic stimulation for 24 hours. Ang1 treatment decreased the hypoxia-induced p27 level, but sTie2 reversed its expression (Fig. 4A). Chromatin immunoprecipitation analysis also confirmed the transcriptional regulation of p27 by Foxo1 (Fig. 4B). We confirmed the anti-apoptotic effect of Ang1 using apoptotic FACS analysis and trypan blue exclusion assay (Fig. 5).
Hypoxic stimulation markedly increased the apoptotic fraction (22.15% vs. 17.1% in normoxia) (Fig. 5A). However, Ang1 decreased hypoxia-induced apoptosis (14.22% vs. 22.15% in hypoxia). sTie2 pre-treatment prior to adding Ang1 strongly reversed the Ang1 effect.
By trypan blue exclusion assay, Ang1-treated HUVECs also showed fewer apoptotic cells in hypoxic conditions, and sTie2 blocked this Ang1 activity (Fig. 5B). These results suggest that Ang1 can regulate Foxo1 and p27, and then suppress hypoxia-induced apoptosis in HUVECs.
Using a mouse hind limb ischemia model, we tested local injections to assess the impact of Ang1 in vivo. One day after unilateral femoral artery excision, mice received local injections (via direct muscular injection) of Ang1 or normal saline as a control.
We observed the fate of ischemic limbs and serial tissue perfusion by LDPI (Fig. 6A). Serial laser Doppler perfusion imaging of the ischemic left hind limb showed that blood flow was recovered in the Ang1-treated group (Fig. 6A).
By histological examination, skeletal muscle sections at day 14 were analyzed by TUNEL assay. TUNEL positive cells (brown) were significantly decreased in Ang1-injected ischemic tissue (Fig. 6B). TUNEL positive cells were significantly decreased in the Ang1 injection group (p<0.05) in ischemic hind limb tissues (Fig. 6C). Immunohistochemical staining for the endothelial marker PECAM-1 was performed on skeletal muscle sections from ischemic limbs at day 14 to quantify capillary density (Fig. 6D). PECAM-1 positive immnoreactivity showed greater capillary density in the Ang1 treated group (Fig. 6D and E). In addition, immunofluorescene staining was performed with antibodies for a blood vessel marker, BS-1 lectin, and a proliferation marker, Ki67 (Fig. 6F). Both BS-1 lectin and Ki67 positive cells were significantly increased by Ang1 (p<0.05) (Fig. 6F and G).
Ang1 is an important molecule in blood vessel biology, and its regulatory mechanisms in vasculogenesis are well characterized. We examined the role of Ang1 on signaling pathways for endothelial survival and protection from apoptosis. Ang1 inhibits endothelial cell apoptosis through several pathways, which include the PI3K pathway by Tie2 receptor binding, inhibition of caspase-9, -7, and -3, suppression of Smac release and upregulation of Survivin protein.11)21) Ang1 can act directly on cardiac and skeletal myocytes through integrins and promotes myocyte survival via specific pathways, such as Akt and MAPK phosphorylation.12)
We found that Ang1 suppressed endothelial apoptosis by PTEN down regulation. PTEN expression was strongly increased by hypoxic stimulation, but Ang1 could decrease PTEN expression in HUVECs (Fig. 1). Endothelial apoptosis was enhanced under hypoxia, whereas Ang1 treatment rescued hypoxia-induced apoptosis (Fig. 5). The function of Ang1 was significantly blocked by pre-treatment with sTie2 (Fig. 5). Due to the down-regulation of Ang1 expression in hypoxia,16) we suggest that PTEN activation is probably mediated by Ang1 reduction. Thus, addition of Ang1 under hypoxic conditions might be effective for anti-apoptosis of both endothelial cells and non-endothelial cells.
PTEN is a well-known tumor suppressor. It induces apoptosis in ovarian cancer, and suppresses tumorigenicity by inducing cell cycle arrest in glioblastoma cells.2)4) Inhibition of PTEN suppresses neuron apoptosis and, thus, shows neuroprotective effects.5) The PTEN gene is activated by NF-kB through the p38MAPK pathway in cardiac endothelial cells.17)
NF-kB is inhibited by IkB, which binds to NF-kB and traps it in the cytoplasm.19) Cytokines activate IkB kinase (IKK) that phosphorylates IkB, which causes IkB degradation by the proteasome and allows translocation of NF-kB to the nucleus to activate transcription. 17)19) In a recent report, hypoxia/reoxygenation activated p38MAPK and inhibition of p38MAPK abolished NF-kB activity in aortic endothelial cells.18) p38MAPK knock-down, using small interfering RNA (siRNA), significantly reduced hypoxia/reoxygenation-induced NF-kB activity.18) In our experiments, hypoxia upregulated pp38 protein. However, hypoxia decreased IkB (Fig. 2A). Interestingly, Ang1 clearly down regulated hypoxiainduced pp38, but it increased IkB protein levels under hypoxia. Furthermore, NF-kB staining was found in the nucleus under hypoxic conditions. When HUVEC cells were treated with Ang1, NF-kB staining was found in the cytosol under hypoxic conditions (Fig. 2B). Thus, Ang1 inactivates NF-kB, at least in part, via inactivation of p38MAPK.
Forkhead transcription factors, Foxo1 and Foxo3a, are downstream of PTEN and have a role in PTEN-mediated cell cycle arrest.20) Inactive forms of Foxo1 and Foxo3a are localized in the cytoplasm. PTEN activates nuclear translocation of Foxo1 from the cytoplasm, thereby enhancing Foxo1 transcriptional activity.20) Foxo1 regulates cardiomyocyte apoptosis, and Foxo3a inhibits vascular smooth muscle cell proliferation and neointimal hyperplasia.22)23)
In our results, Foxo1 moved to the nucleus under hypoxia (Fig. 3A), then moved out of the nucleus by Ang1 treatment. p-Foxo1 is an inactive form of Foxo1. The level of p-Foxo1 shows a reciprocal regulation to that of nuclear Foxo1 protein levels (Fig. 3B). The nuclear translocation of Foxo3a was not changed by hypoxic conditions and Ang1 treatment (Fig. 3A).
Cell-cycle inhibitor protein, p27, was also regulated by Ang1, and the Foxo1 binding level to the p27 promoter was reduced by Ang1 (Fig. 4). The expression of p27 is regulated at several steps, such as protein stability and transcription.3)24) PTEN increases p27 protein accumulation, and AFX-like forkhead transcription factors stimulate p27 mRNA.3)24) Our results suggest that Ang1 might inactivate Foxo1 by inhibiting nuclear translocation of Foxo1. This down regulates p27 and suppresses PTEN-mediated apoptosis.
It has been reported that Ang1 prevents radiationinduced endothelial cell apoptosis.11) Radiation therapy is useful for preventing restenosis to treat coronary artery disease patients and is essential for cancer patients.11)13) However, radiation can damage vascular endothelial cells. Thus, it has been suggested that pre-treatment with Ang1 might prevent endothelial apoptosis and enhance therapeutic efficacy for these patients. In addition, adenoviral-Ang1 overexpression results in smaller infarct zones and preserves cardiac function in rat myocardial infarction.25) Enhanced muscle survival and skin flaps have also been reported in rat.26)
We observed diminished cell death, higher capillary density and more proliferating cells in skeletal muscle tissue of Ang1-injected mice. In the Ang1 local injection group, remarkable limb salvage occurred by day 14 (Fig. 6), suggesting that increases in perfusion and/or capillary density by Ang1 might lead to protective effects for endothelial and other tissues.
Collectively, our results suggest that Ang1 enhances cell survival by reducing PTEN-mediated apoptosis in endothelial cells under hypoxic conditions. It improves blood flow recovery, and thereby prevents limb loss of ischemic hind limb mice. A better understanding of the regulatory mechanisms for endothelial anti-apoptosis by Ang1 could provide useful therapeutics for recovery from ischemic disease, including myocardial infarction.
Figures and Tables
Fig. 1
Ang1 reduced the expression of PTEN that was upregulated in HUVEC under hypoxic conditions. A: trypan blue exclusion assay showed that endothelial apoptosis increased in a time-dependent manner (*p<0.01). B: the expression of PTEN was upregulated under hypoxic conditions. Ang1 markedly reduced PTEN expression in HUVECs after 24 hours and 36 hours under hypoxia. C and D: RT-PCR and Western blot showed that Ang1 decreased hypoxia-induced PTEN expression. C: control, A: Ang1 (200 ng/mL), sTie2 (10 µg/mL). Ang1: angiopoietin-1, PTEN: phosphatase and tensin homologue deleted from chromosome ten, HUVECs: human umbilical vein en-dothelial cells, RT-PCR: reverse transcriptase-polymerase chain reaction.
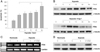
Fig. 2
Ang1 inhibits p38MAPK and NF-kB activity in HUVECs under hypoxia. A: p38MAPK signaling pathways were tested by Western blot. p38 phosphorylation was strongly increased after 24 hours hypoxia, while Ang1 (200 ng/mL) clearly down regulated phospho-p38 (pp38). sTie2 (10 µg/mL) reversed Ang1 effect. Quantification of pp38 and IkB immunoblots from 2 independent experiments are shown at right. B: immunofluorescence staining for NF-kB in HUVECs after hypoxic stimulation for 24 hours. NF-kB stained red, the nucleus stained blue. C: control, A: Ang1 treatment (200 ng/mL), sTie2 (10 µg/mL). Ang1: angiopoietin-1, MAPK: mitogen activated protein kinase, NF-kB: nuclear factor-kappa B, HUVECs: human umbilical vein en-dothelial cells, IkB: inhibitory kappa B.
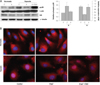
Fig. 3
Ang1 regulates forkhead transcription factor, Foxo1, but not Foxo3a. A: western blot using the nuclear fraction showed Foxo1 nuclear translocation. Ang1 (200 ng/mL) inhibited Foxo1 nuclear translocation after 24 hours hypoxia. There was no difference for Foxo3a translocation. B: western blot using whole cell lysates showed that the expression of Foxo1 was consistent. p-Foxo1 decreased under hypoxia, but increased with Ang1. C: control, A: Ang1 (200 ng/mL), sTie2 (10 µg/mL). Ang1: angiopoietin-1.
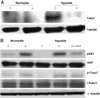
Fig. 4
Ang1 decreased the expression of p27 under hypoxia. A: the expression of p27 in HUVECs treated with 200 ng/mL Ang1 for 24 hours hypoxia was analyzed by RT-PCR. B: chromatin immunoprecipitation assay of p27 in specific genomic sequences from Ang1 treated HUVECs with anti-Foxo1 monoclonal antibody. Foxo1 binding activity in the p27 promoter region was strongly reduced by Ang1. C: control, A: Ang1 (200 ng/mL), sTie2 (10 µg/mL). Ang1: angiopoietin-1, HUVECs: human umbilical vein endothelial cells, RT-PCR: reverse transcriptase-polymerase chain reaction.
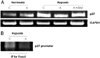
Fig. 5
Ang1 protected against hypoxia-induced HUVECs apoptosis. A: apoptotic fluorescence-activated cell sorting analysis measuring the hypo-diploid DNA contents, showing a significantly increased apoptotic fraction in the hypoxia group. However, Ang1 reduced endothelial apoptosis. B: trypan blue exclusion assay showed HUVECs viability at 24 hours and 48 hours after hypoxia with Ang1 treatment (*p<0.01). C: control, A: Ang1 (200 ng/mL), sTie2 (10 µg/mL). Ang1: angiopoietin-1, HUVECs: human umbilical vein endothelial cells, DNA: deoxyribonucleic acid.
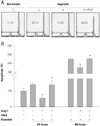
Fig. 6
Ang1 showed greater efficacy to improve perfusion and reduce limb loss in mouse hind limb ischemia model. A: representative laser Doppler perfusion imaging (LDPI) analyses of hind limb blood perfusion for each group showing enhanced blood flow recovery in the Ang1 group. Local administration of 100 µg Ang1 by 3 injections into tight muscle (n=7) was evaluated by LDPI. B: representative figure of TUNEL staining at day 14. There were fewer TUNEL (+) cells (brown) in Ang1 injection group. C: quantitative analysis for apoptosis. TUNEL (+) cells were significantly decreased in Ang1 injection group (n=10; *p<0.05). D: immunofluorescence staining for PECAM-1 (green) in ischemic limb tissues at post-operative day 14. E: capillaries positive for PECAM-1 staining were significantly increased in Ang1 injection group (*p=0.016). F: immunofluorescene staining for BS-1 lectin and Ki67 in ischemic limb tissues at day 3, day 7 and day 14. There were more capillaries positive for BS-1 lectin (green) and Ki67 (red) in the Ang1 treatment group than in the saline group. G: Ki67/BS-1 lectin double positive cells were counted in 10 different microscopic fields. At least 3 different sections from each animal were used for counting (*p<0.05). Ang1: angiopoietin-1, TUNEL: terminal deoxynucleotidyltransferase-mediated dUTP nick-end labeling, PECAM: platelet endothelial cell adhesion molecule.
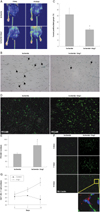
Acknowledgments
This study was supported by a grant from the Innovative Research Institute for Cell Therapy (A0622).
References
1. Di Cristofano A, Pandolfi PP. The multiple roles of PTEN in tumor suppression. Cell. 2000. 100:387–390.
2. Li DM, Sun H. PTEN/MMAC1/TEP1 suppresses the tumorigenicity and induces G1 cell cycle arrest in human glioblastoma cells. Proc Natl Acad Sci U S A. 1998. 95:15406–15411.
3. Haas-Kogan D, Shalev N, Wong M, Mills G, Yount G, Stokoe D. Protein kinase B (PKB/Akt) activity is elevated in glioblastoma cells due to mutation of the tumor suppressor PTEN/MMAC. Curr Biol. 1998. 8:1195–1198.
4. Yan X, Fraser M, Qiu Q, Tsang BK. Over-expression of PTEN sensitizes human ovarian cancer cells to cisplatin-induced apoptosis in a p53-dependent manner. Gynecol Oncol. 2006. 102:348–355.
5. Delgado-Esteban M, Martin-Zanca D, Andres-Martin L, Almeida A, Bolaños JP. Inhibition of PTEN by peroxynitrite activates the phosphoinositide-3-kinase/Akt neuroprotective signaling pathway. J Neurochem. 2007. 102:194–205.
6. Jain RK. Molecular regulation of vessel maturation. Nat Med. 2003. 9:685–693.
7. Yancopoulos GD, Davis S, Gale NW, Rudge JS, Wiegand SJ, Holash J. Vascular-specific growth factors and blood vessel formation. Nature. 2000. 407:242–248.
8. Kim HJ, Kim DK. Angiogenic gene therapy in patients with ischemic cardiovascular diseases. Korean Circ J. 2003. 33:7–14.
9. Lee SW, Kim WJ, Choi YK, et al. SSeCKS regulates angiogenesis and tight junction formation in blood-brain barrier. Nat Med. 2003. 9:900–906.
10. Nag S. The blood-brain barrier and cerebral angiogenesis: lessons from the cold-injury model. Trends Mol Med. 2002. 8:38–44.
11. Cho CH, Kammerer RA, Lee HJ, et al. Designed angiopoietin-1 variant, COMP-Ang1, protects against radiation-induced endothelial cell apoptosis. Proc Natl Acad Sci U S A. 2004. 101:5553–5558.
12. Dallabrida SM, Ismail N, Oberle JR, Himes BE, Rupnick MA. Angiopoietin-1 promotes cardiac and skeletal myocyte survival throuth integrins. Circ Res. 2005. 96:e8–e24.
13. Park SJ, Kim DH, Seo JB, et al. Thalidomide as a potent inhibitor of neointimal hyperplasia after balloon injury in rat carotid artery. Korean Circ J. 2004. 34:346–355.
14. Freedman SJ, Sun ZY, Kung AL, France DS, Wagner G, Eck MJ. Structural basis for negative regulation of hypoxia-inducible factor-1 alpha by CITED2. Nat Struct Biol. 2003. 10:504–512.
15. Hwang KK, Kim HS, Seo JU, et al. Apoptosis in the human neonatal vascular remodeling. Korean Circ J. 2001. 31:681–700.
16. Choi YK, Kim JH, Kim WJ, et al. AKAP12 regulates human blood-retinal barrier formation by downregulation of hypoxiainducible factor-1 alpha. J Neurosci. 2007. 27:4472–4481.
17. Chandrasekar B, Valente AJ, Freeman GL, Mahimainathan L, Mummidi S. Interleukin-18 induces human cardiac endothelial cell death via a novel signaling pathway involving NF-kappaB-dependent PTEN activation. Biochem Biophys Res Commun. 2006. 339:956–963.
18. Ryan S, McNicholas WT, Taylor CT. A critical role for p38 map kinase in NF-kappaB signaling during intermittent hypoxia/reoxygenation. Biochem Biophys Res Commun. 2007. 355:728–733.
19. DiDonato JA, Hayakawa M, Rothwarf DM, Zandi E, Karin M. A cytokine-responsive IkappaB kinase that activates the transcription factor NF-kappaB. Nature. 1997. 388:548–554.
20. Nakamura N, Ramaswamy S, Vazquez F, Signoretti S, Loda M, Sellers WR. Forkhead transcription factors are critical effectors of cell death and cell cycle arrest downstream of PTEN. Mol Cell Biol. 2000. 20:8969–8982.
21. Harfouche R, Hasséssian HM, Guo Y, et al. Mechanisms which mediate the antiapoptotic effects of angiopoietin-1 on endothelial cells. Microvasc Res. 2002. 64:135–147.
22. Morris JB, Kenney B, Huynh H, Woodcock EA. Regulation of the proapoptotic factor FOXO1 (FKHR) in cardiomyocytes by growth factors and alpha1-adrenergic agonists. Endocrinology. 2005. 146:4370–4376.
23. Lee HY, Chung JW, Youn SW, et al. Forkhead transcription factor FOXO3a is a negative regulator of angiogenic immediate early gene CYR61, leading to inhibition of vascular smooth muscle cell proliferation and neointimal hyperplasia. Circ Res. 2007. 100:372–380.
24. Medema RH, Kops GJ, Bos JL, Burgering BM. AFX-like Forkhead transcription factors mediate cell-cycle regulation by Ras and PKB through p27kip1. Nature. 2000. 404:782–787.
25. Takahashi K, Ito Y, Morikawa M, et al. Adenoviral-delivered angiopoietin-1 reduces the infarction and attenuates the progression of cardiac dysfunction in the rat model of acute myocardial infarction. Mol Ther. 2003. 8:584–592.
26. Jung H, Gurunluoglu R, Scharpf J, Siemionow M. Adenovirus-mediated angiopoietin-1 gene therapy enhances skin flap survival. Microsurgery. 2003. 23:374–380.