Abstract
Background and Objectives
Virtual histology-intravascular ultrasound (VH-IVUS) studies on early-stage fibroatheroma, the probable precursor lesion of progression to thin-cap fibroatheroma (TCFA), have only rarely been done in man. We investigated the progression and observational frequency of fibroatheromas, and compared plaque components between early-stage and advance-staged fibroatheromas in the general population.
Subjects and Methods
We assessed coronary fibroatheromas using VH-IVUS and histopathologic analysis of 109 coronary lesions from 40 autopsied cases that were not due to sudden cardiac death (NSCD cases). Fibroatheromas were grouped into early fibroatheroma, late fibroatheroma, thick-cap fibroatheroma (TkCFA), and thin-cap fibroatheroma.
Results
Mean patient age was 45±11 years old and 71% were males. Of 109 lesions, 27% were early fibroatheromas, 53% late fibroatheromas, 9% TkCFA, and 11% TCFA. VH-IVUS showed that there was relatively less fibrotic and fibrofatty plaque and more dense calcium deposits as fibroatheromas progressed. Furthermore, the relative amounts of fibrotic and fibrofatty plaque decreased (r=0.773, p<0.001 and r=0.538, p<0.001, respectively) as the necrotic core increased, while the relative area of dense calcium increased (r=0.665, p<0.001) as the size of the necrotic core increased.
Studies of atheromatous plaques based on histopathologic analysis are frequently performed at autopsy following sudden cardiac death (SCD).1-3) With advances in medical technology, the progression of atheromatous plaque has recently been evaluated based on a serologic examination of biomarkers.4-6) Various imaging modalities have also been used in clinical practice.7-11) Based on clinical usefulness, the imaging modalities most frequently used are intravascular ultrasound (IVUS) or virtual histology-IVUS (VH-IVUS) along with coronary angiography and percutaneous coronary intervention. Through these modalities, the rupture and erosion of atheromatous plaque, the cause of thrombosis within the coronary artery, can be confirmed. Furthermore, vulnerable plaque, which can obstruct the coronary artery, can be detected as early as possible. Thus, these modalities help the treatment strategy to be determined.9) Of the previous studies which have been conducted using IVUS or VH-IVUS, however, there are few about the early stage of atheromatous plaques. In addition, the definition of atheromatous plaque remains unclear. Also, studies which have been conducted using histopathologic analysis are also rare in the literature. In recent years, VH-IVUS, which can go beyond the scope of the gray scale IVUS, and which can definitively evaluate the composition of atheromatous plaque, has been used in the clinical setting. However, results of its use have been reported for patients with stable angina or acute coronary syndrome (ACS), and those who had risk factors or angina symptoms. To date, there have not been a great number of studies conducted to examine the progression of atheromatous plaque using VH-IVUS in a general population. It is commonly encountered in the clinical setting in Korea that the prevalence of cardiovascular diseases has been continually increasing with the westernization of life style. However, where doing autopsies has not been universalized, there is still insufficient objective data about atheromatous plaque in Koreans whose ethnic characteristics are considered different from Caucasians.
Given the above, we performed VH-IVUS in Korean that were not due to sudden cardiac death (NSCD) cases of various ages and examined the distribution pattern and frequency of atheromatous plaque at various growth stages. We also compared the composition of atheromatous plaque at both early and advanced stages using VH-IVUS and then attempted to histopathologically demonstrate confirm our results.
Early fibroatheroma was diagnosed if the amount of plaque accounted for >40% of the vessel lumen, the area of necrotic core was <0.1 mm2, and the amount of necrotic core was >10%. Late fibroatheroma was diagnosed if the amount of plaque accounted for >40% of the vessel lumen, the area of necrotic core ranged from 0.1-1.0 mm2, and the amount of necrotic core was >10%.12)13) Cases in which the amount of plaque accounted for >40% seen on VH-IVUS, the amount of necrotic core was >10% and there were more than three consecutive necrotic cores attached to the vascular lumen, were identified as a thin-cap fibroatheroma (TCFA). Similar to TCFA, in cases in which the amount of plaque was >40%, and the amount of necrotic core was >10%, but there was a fibrous cap extending from the vascular lumen to a necrotic core, and the necrotic core was not attached to but remote from the vascular lumen, we identified the lesion as thick-cap fibroatheroma (TkCFA).13) TCFA and TkCFA, defined herein, were considered advance-staged fibroatheromas and were compared with early or late fibroatheroma.
Our study was approved by the Institutional Review Board of Chung-Ang University Hospital Center. Coronary arteries were collected from 43 cadavers (mean age 45±11 years old, male 71%) whose autopsies were requested by the National Institute of Scientific Investigation. Three cases where the cause of death proved to be sudden cardiac death were excluded from the current analysis. Of 120 coronary arteries which were collected from the remaining 40 cases, six coronary arteries to which VH-IVUS could not be applied were also excluded from our analysis. Of the remaining 114 coronary arteries, the following cases were also excluded from our analysis: 1) an inability to interpret the findings seen on VH-IVUS due to the presence of an artifact, 2) a loss of the coronary arteries during the preparation of tissue samples for histopathologic analysis, and 3) ineligibility because of inadeguate criteria for determining early fibroatheroma and late fibroatheroma. For the remaining 82 coronary arteries, 109 atheromatous plaques were analyzed.
VH-IVUS was performed using a 20 MHz, 2.9 F monorail, electronic Eagle Eye Gold IVUS catheter (Volcano Therapeutics, Rancho Cordova, CA, USA). First, the heart was extracted from the cadaver and the coronary artery was dissected. Dissected coronary arteries were placed in saline in a 37℃ water bath. To minimize vascular damage, a floppy-type 0.014 inch guide wire was inserted and VH-IVUS was performed for all three coronary arteries based on conventional methods. With the use of a motorized pullback system, images were obtained at a rate of 0.5 mm/sec from the ostium of the left and right coronary arteries to a point 50 mm distal. All images were stored on a DVD-ROM. Offline analysis of the images, recorded from the ostium of the coronary artery to a point 30 mm distance, was conducted.
All tissues were fixed using a buffered-formalin solution immediately after VH-IVUS was performed. At a 3 mm gap, the coronary artery was dissected perpendicular to the long axis. Then, paraffin-embedded blocks were prepared. Tissue sections were then prepared at a thickness of 4-5 µm. This was followed by dual staining with the use of Myer's hematoxylin and 0.5% eosin solution, and sections were examined using a light microscope. At the same time, to examine the degree of atheromatous plaque and intimal fibrosis, Masson-trichrome staining was performed. To assess the degree of calcification, vonKossa staining was performed.
Paraffin-embedded tissue samples were deparaffinized and treated with 0.5% hydrogen peroxide (H2O2) to inhibit the activity of intrinsic peroxidases. To restore antigenicity, samples were immersed in a 10 mM citrate (pH 6.0) buffer solution and rinsed with a 50 mM Tris buffer solution (TBS, pH 7.2-7.4). To inhibit non-specific reactions, the reaction was performed using 3% normal goat serum. Primary antibodies such as anti-CD68 (Lab Vision Corp., Fremont, CA, USA), anti-CD31 (Lab Vision Corp., Fremont, CA, USA) and anti-matrix metalloproteinase-9 (MMP-9; Lab Vision Corp., Fremont, CA, USA) were reacted with tissue samples overnight at room temperature. The second reaction was performed using a Biotin-conjugated secondary antibody (Zymed Laboratories Inc., South San Francisco, CA, USA) at room temperature. Then, the peroxidase-labelled streptoavidin complex was added. AEC chromogen (3-amino-9-ethylcarbazole) was added to a buffer solution and used as a substrate solution. Counter-staining was performed using Myer's hematoxylin. This was followed by light microscopy. Tissues stained with normal serum served as controls.
Findings seen on VH-IVUS were analyzed by two independent cardiologists. Atheromatous plaques were classified as belonging to one of four groups: early fibroatheroma, late fibroatheroma, TkCFA or TCFA. In each group, the composition of plaque was divided into fibrous plaque, fibro-fatty plaque, necrotic core and dense calcium.
Following this, quantitative analysis was done. Histopathologic analysis was done by two independent pathologists who were blinded to the IVUS findings. Histopathologic characteristics and immunohistochemical properties of the different groups were compared.
Continuous variables were expressed as mean±standard deviation (SD). For parametrical analysis between two groups, an independent t-test was used. When there were three or more groups, one-way analysis of variance (ANOVA) was used. For a non-parametrical analysis, the Mann-Whitney U-test was used. For categorical variables, statistical significance was analyzed using the chi-square test. A correlation analysis of continuous variables was done using Pearson's correlation. All statistical data were analyzed using version 11.5 of the Statistical Package for the Social Science (SPSS Inc., Chicago, IL, USA). P<0.05 was considered statistically significant.
Of the 109 atheromatous plaques analyzed using VH-IVUS, early fibroatheroma was detected in 29 cases (27%), late fibroatheroma in 58 cases (53%), TkCFA in 10 cases (9%) and TCFA in 12 cases (11%). Mean age was 39±8 years, 45±12 years, 46±10 years and 52±7 years, respectively (p=0.001) (Fig. 1).
There were no significant sex-related differences in atheromatous plaques at each stage. But age differences were significantly different (p=0.002). The 12 cases of TCFA were all detected in men aged >40 years (Fig. 1).
Early fibroatheroma was mainly found in the left circumflex artery (LCX) and the right coronary artery (RCA). Late fibroatheroma was detected at similar frequencies in all three coronary arteries. Also, 58% (7/12) of TCFA cases were found in the left anterior descending artery (LAD) (p=0.084).
The distribution of atheromatous plaque was evaluated across a range subdivided by 5 mm gaps. This showed that atheromatous plaque at all stages was distributed proximal to the ostium of each coronary artery (p=0.930) (Fig. 2). The distribution of TCFA cases was evenly observed within an overall 30 mm range in the LAD, predominantly distributed in the distal 15 mm range in the LCX and observed within a 15 mm range in the proximal RCA (Fig. 3).
For atheromatous plaque at the different phases, the external elastic lamina areas and lumen areas showed significant differences (p<0.001, p=0.043, respectively). Changes in composition of atheromatous plaque were examined and showed that among phases there were significant differences in fibrous area, fibro-fatty area, necrotic core area and dense calcium area (p<0.001) (Table 1).
Regarding the plaque composition in progression, as the size of the necrotic core area was increased, that of the fibrous area (r=-0.773, p<0.001) and fibro-fatty area (r=-0.538, p<0.001) decreased. In contrast, the dense calcium area (r=0.665, p<0.001) increased (Fig. 4). In summary, compared with early fibroatheroma, the dense calcium area increased in proportional to the necrotic core area in late fibroatheroma and TCFA.
We analyzed differences in histopathologic findings between phases of atheromatous plaque. There was a significant difference in the size of the necrotic core area between early fibroatheroma, late fibroatheroma and advanced fibroatheroma. This is in agreement with the findings we saw on VH-IVUS (p<0.001) (Table 2). Fibrosis was decreased and calcium deposition was significantly increased (p=0.022) (Fig. 5) (Table 2). Also, the concordance rate between the histopathologic findings and findings seen on VH-IVUS was 94.4% (p<0.001).
Moreover, immunohistochemistry revealed that the infiltration of CD68-positive macrophages was significantly increased in late fibroatheroma and advanced fibroatheroma as compared with early fibroatheroma (p<0.001) (Fig. 5) (Table 2). It was also observed that matrix metalloprotease-9 (MMP-9) activity was significantly increased (p=0.006) (Fig. 6) (Table 2). To identify the correlation between neovascularization and the growth of atheromatous plaque, CD31 staining was done. This showed that the expression of CD31 was significantly increased in advanced fibroatheroma as compared with early fibroatheroma (p=0.045) (Fig. 6) (Table 2).
In the present study, the progression of atheromatous plaque was assessed using VH-IVUS in the coronary arteries of Korean NSCD autopsy cases. Plaque composition of the groups was compared. Atheromatous plaques were divided into early fibroatheroma, late fibroatheroma, TkCFA and TCFA, and the frequency and distribution pattern for each of these groups was examined.
Through the present study, several novel findings were disclosed as shown below. First, a correlation between neovascularization and the growth of atheromatous plaque was identified. Previous histologic studies have shown that the degree to which the vasa vasorum was developed and neovascularizations were formed in the atheromatous plaque was correlated with the instability of atherosclerotic plaque and clinical symptoms.14-17) To date, however, no studies have established the specific stages of atheromatous plaque in which neovascularizations are actively formed based on VH-IVUS findings. In the present study, based on the findings seen on VH-IVUS, the expression of CD 31 was not seen in early fibroatheroma. From late fibroatheroma on, however, it was observed. In the TkCFA and TCFA stages, the neovascularization was marked (p=0.045). These results show that the classification of atheromatous plaque based on VH-IVUS is in agreement with histopathologic findings, which are also assumed to be of significance in that the histopathologic findings can be applied to an actual clinical practice. Kolodgie et al.18) reported that the growth of atheromatous plaque was accelerated by the presence of plaque hemorrhage and this led to plaque rupture in SCD patients. However, in the present study, plaque hemorrhage was not observed in TkCFA and TCFA. This might be due to a small number of enrollments from NSCD.
Second, there was a clustering of early and late fibroatheroma. Previous studies reported that vulnerable plaque, such as ruptured plaque or TCFA, showed a clustering on the proximal coronary artery.19) To date, however, no studies have investigated early or late fibroatheroma in this series. In the present study, it was confirmed that early or late fibroatheroma were also observed more frequently on the proximal coronary artery. Previous studies based on quantitative coronary angiography have shown that the obstructive lesion was mainly present on the proximal coronary artery. Another study based on IVUS also confirmed a higher incidence of plaque rupture in the proximal area.19)20) In the present study, early and late fibroatheroma also clustered in the proximal area. These results indirectly illustrate a series of processes that vulnerable plaque, TCFA, is well-developed and progresses from early staged fibroatheroma in the proximal area, and this eventually leads to plaque rupture.
Third, the incidence of TCFA is higher than what has been reported. One of the vulnerable plaques, TCFA has been considered a precursor lesion of ACS.21) According to a recent report, it was detected at a frequency of 1.6% in SCD patients with and 1.1% in NSCD.22) The present study was conducted in NSCD patients, in whom the frequency of TCFA was observed to be 11%. Unlike the previous studies, the present study showed a difference in that it was conducted within a <30 mm range. Considering that the present study was conducted in an Asian population whose eating habits are different from those of Caucasian populations, however, it is noteworthy that the incidence of TCFA was 11%. Following an analysis of TCFA based on findings seen on IVUS, Rodriguez-Granillo et al.13) reported that there was no significant difference in the male-to-female ratio, 66.7% were detected within a region <20 mm from the origin of the coronary artery, and the presence of TCFAs was mainly found in the LAD. Interestingly, in the present study all 12 cases of TCFA were observed in male patients. These results can be associated with differences in treatment response and clinical characteristics between male and female patients,23)24) but this deserves further study. In our study, however, 82% of TCFAs were detected within the first <20 mm range of coronary artery when three coronary arteries were analyzed and 58% were distributed in the LAD. These findings are in agreement with previous reports.13)
The clinical significance of the present study includes the following. First, atheromatous plaques were easily re-classified by a method that could be applied to clinical practice. To date, the classification of atheromatous plaque has been based on the American Heart Association (AHA) classification, which was proposed by autopsy findings or findings for experimental animal models.25) Because this classification is complicated and its theoretical extension was depicted as histopathological findings, however, its clinical application has been considered to be obscure. Although the AHA classification has been simplified by the recent widespread use of IVUS or VH-IVUS, obscurely-classified atheromatous plaque is still found. Given this background, and based on previous studies using histopathology12) and IVUS,13) we attempted to establish new criteria, classifying fibroatheromas as belonging to several phases, phases which are observed during the application of VH-IVUS, on a real-time basis. Also, definitions of early and late fibroatheroma, which had been obscure up to now, were established in the present study.
Second, the present study provides data about the accuracy of VH-IVUS compared to histopathologic findings. VH-IVUS was introduced in a clinical setting and then widely used, although disagreements still exist regarding its accuracy. This is somewhat confusing. In an animal experiment, Granada et al.26) reported that VH-IVUS had a diagnostic sensitivity of 76.1% for fibrous, 46% for fibro-fatty and 41.1% for necrotic core components. Nasu et al.,27) however, reported that the accuracy of VH-IVUS was 87.1% for fibrous, 87.1% for fibro-fatty, 88.3% for necrotic core and 96.5% for calcium components in 30 patients with stable angina or ACS. In the present study, the concordance rate between VH-IVUS and histopathologic findings was 94.4%. In cases of early fibroatheroma, the size of the necrotic core was 0.08±0.04 mm2 on VH-IVUS and 0.08±0.09 mm2 on histopathology. In cases of late fibroatheroma, it was 0.44±0.22 mm2 and 0.33±0.57 mm2, respectively. In cases of advanced fibroatheroma, calcium components had areas of 0.54±0.33 mm2 on VH-IVUS and 0.52±0.93 mm2 on histopathology. Because NSCD cases were included and their number was not so great in the present study, there could be some discrepancies from previous reports. Through the present study, it was confirmed that there was a higher concordance rate between VH-IVUS and histopathologic findings.
Third, the rate of atheromatous plaque progression was assessed in the Korean population. Mean age was 39 years in cases of early fibroatheroma and 52 years in those of TCFA. TCFA was not found in patients aged less than 40 years. A 13-year difference in the mean age does not mean that it would take early fibroatheroma 13 years to progress to TCFA. Nevertheless, the growth rate of atheromatous plaque was estimated in Koreans. Considering that this growth phase reflects ethnic or cultural factors,28) these results for Koreans are of great clinical significance. Also, the present study was done using NSCD cases rather than SCD ones. This implies that the growth of atheromatous plaque was examined in a general population rather than in people at increased risk of developing atherosclerosis. Therefore, it is important for understanding the natural course of atherosclerosis.
Limitations of the present study are as follows. First, it is probable that coronary arteries extracted for an autopsy have degenerated. Previous studies have also pointed out that constriction of arteries occurs after death and during the fixation of tissue sample.29) In contrast, it has also been maintained that the process of tissue fixation does not affect the absolute wall area which was calculated by the subtraction of the cross-sectional area of the vascular lumen from the total surface area of blood vessels.30) Thus, results of the present study could therefore be deserve. Second, clinical data such as concurrent diseases (hypertension, diabetes mellitus, dyslipidemia), cholesterol levels and the use of anti-hyperlipidemic drugs could not be obtained for our study cases. Third, there is a possibility that intravascular blood clots or thrombi were removed by the infusion of saline into the autopsied coronary arteries, or that the vascular wall was damaged due to manipulation of the wire.
Despite these limitations, through the present study, vascular age as a function of the growth phase of the atheromatous plaque was determined for Koreans. The current study also provides criteria for easily classifying the growth phase of atheromatous plaque based on findings seen on VH-IVUS. A confirmation of the changes in composition of atheromatous plaque based on VH-IVUS and histopathologic findings would be a valuable baseline for understanding the pathophysiology of atherosclerosis in the Korean population.
In conclusion, the incidence of atheromatous plaque seen in NSCD Korean cases show that 27% were early fibroatheroma, 53% late fibroatheroma, 9% TkCFA and 11% TCFA. Mean age was 39±8 years, 45±12 years, 46±10 years and 52±7 years, respectively. These differences were significantly different. Also, growth and progression of atheromatous plaque can be said to be due to increased concentration of serum calcium as well as an increased amount of necrotic core. The role of calcium in this series deserves further studies.
Figures and Tables
Fig. 1
Observed frequencies of plaques by age and gender. There was a significant difference in observed frequency between groups by age (p=0.002) (A). TCFA was not observed in cases under 40 years. There was no significant difference in observed frequency between groups by gender (p=0.128) (B). TCFA was only noted in males. FA: fibroatheroma, TkCFA: thick-cap fibroatheroma, TCFA: thin-cap fibroatheroma.
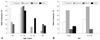
Fig. 2
Cumulative frequency distribution of plaques. The proximal clusterings of plaques at various stages were observed to be a function of the distance from the coronary artery ostium. However, there was not a significant difference in frequency distribution between groups (p=0.93). FA: fibroatheroma, TkCFA: thick-cap fibroatheroma, TCFA: thin-cap fibroatheroma.
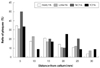
Fig. 3
Spatial distribution of plaques in each of the coronary arteries. We noted the number of plaques at the various stages in millimeters from the coronary vessel ostium. There was no significant difference in distribution between groups in each of the coronary arteries (A through C, p=0.619, p=0.547, p=0.756, respectively). LAD: left anterior descending artery, LCX: left circumflex artery, RCA: right coronary artery, NC: necrotic core, TkCFA: thick-cap fibroatheroma, TCFA: thin-cap fibroatheroma.
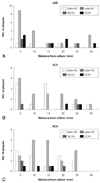
Fig. 4
Correlations between necrotic core area and other plaque components. The relative amounts of fibrotic (A) and fibro-fatty (B) plaque decreased (r=0.773, p<0.001 and r=0.538, p<0.001, respectively) as necrotic core increased, while the relative amount of dense calcium area (C) increased (r=0.665, p<0.001) as the size of the necrotic core increased. F: fibrous, FF: fibro-fatty, DC: dense calcium.
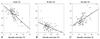
Fig. 5
Microscopic findings stained by Masson-trichrome, vonKossa, and expression of CD68 as plaque progressed. Top panel: the densities of Masson-trichrome stains were decreased as plaques progressed from early (A) to advanced (C) fibroatheroma. Middle panel: the calcifications were not noted at early (D) and late (E) fibroatheroma stages, while they were observed at advanced (F) fibroatheroma. Bottom panel: immunohistochemical staining demonstrated that infiltration of numerous CD68-positive macrophages into plaques was increased as the stage of the plaque increased. Left column (A, D and G): early fibroatheroma, middle column (B, E and H): late fibroatheroma, right column (C, F and I): advanced fibroatheroma (×40).
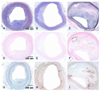
Fig. 6
Expression of MMP-9 and CD31 as a function of plaque growth. Top panel: the expression of MMP-9 was increased more in advanced fibroatheroma (B) rather than in early fibroatheroma (A). Bottom panel: the number of CD31-positive microvessels in plaque was not observed in early fibroatheroma (C), while they were increased in advanced fibroatheroma (D). Left column (A and C): early fibroatheroma, right column (B and D): advanced fibroatheroma (A: ×200, B through D: ×100).
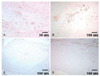
Table 1
Gray scale IVUS and VH-IVUS parameters as plaque progression
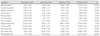
Values are mean±SD. ANOVA was used to compare data between groups. Values of p<0.05 were considered statistically significant. IVUS: intravascular ultrasound, VH-IVUS: virtual histology-intravascular ultrasound, FA: fibroatheroma, TkCFA: thick-cap fibroatheroma, TCFA: thin-cap fibroatheroma, EEL: external elastic lamina, F: fibrous, FF: fibro-fatty, NC: necrotic core, DC: dense calcium, ANOVA: analysis of variance
References
1. Kolodgie FD, Narula J, Burke AP, et al. Localization of apoptotic macrophages at the site of plaque rupture in sudden coronary death. Am J Pathol. 2000. 157:1259–1268.
2. Arbustini E, Dal Bello B, Morbini P, et al. Plaque erosion is a major substrate for coronary thrombosis in acute myocardial infarction. Heart. 1999. 82:269–272.
3. Schmermund A, Schwartz RS, Adamzik M, et al. Coronary atherosclerosis in unheralded sudden coronary death under age 50: histo-pathologic comparison with 'healthy' subjects dying out of hospital. Atherosclerosis. 2001. 155:499–508.
4. Tardif JC, Heinonen T, Orloff D, Libby P. Vascular biomarkers and surrogates in cardiovascular disease. Circulation. 2006. 113:2936–2942.
5. Park SH. Risk stratification of acute coronary syndrome. Korean Circ J. 2002. 32:735–755.
6. Koenig W, Khuseyinova N. Biomarkers of atherosclerotic plaque instability and rupture. Arterioscler Thromb Vasc Biol. 2007. 27:15–26.
7. Motoyama S, Kondo T, Sarai M, et al. Multislice computed tomographic characteristics of coronary lesions in acute coronary syndromes. J Am Coll Cardiol. 2007. 50:319–326.
8. Kawasaki M, Bouma BE, Bressner J, et al. Diagnostic accuracy of optical coherence tomography and integrated backscatter intravascular ultrasound images for tissue characterization of human coronary plaques. J Am Coll Cardiol. 2006. 48:81–88.
9. DeMaria AN, Narula J, Mahmud E, Tsimikas S. Imaging vulnerable plaque by ultrasound. J Am Coll Cardiol. 2006. 47:8 Suppl. C32–C39.
10. Bae JH, Rihal CS, Lerman A. Tissue characterization of coronary plaques using intravascular ultrasound/virtual histology. Korean Circ J. 2006. 36:553–558.
11. Nair A, Kuban BD, Tuzcu EM, Schoenhagen P, Nissen SE, Vince DG. Coronary plaque classification with intravascular ultrasound radiofrequency data analysis. Circulation. 2002. 106:2200–2206.
12. Virmani R, Kolodgie FD, Burke AP, et al. Atherosclerotic plaque progression and vulnerability to rupture: angiogenesis as a source of intraplaque hemorrhage. Arterioscler Thromb Vasc Biol. 2005. 25:2054–2061.
13. Rodriguez-Granillo GA, Garcia-Garcia HM, Mc Fadden EP, et al. In vivo intravascular ultrasound-derived thin-cap fibroatheroma detection using ultrasound radiofrequency data analysis. J Am Coll Cardiol. 2005. 46:2038–2042.
14. Bae JH, Kwon TG, Kim KH, Hyun DW, Kim KY, Kim DS. Invivo coronary plaque composition in patients with acute coronary syndrome: a virtual histology intravascular ultrasound study. Korean Circ J. 2007. 37:437–442.
15. Doyle B, Caplice N. Plaque neovascularization and antiangiogenic therapy for atherosclerosis. J Am Coll Cardiol. 2007. 49:2073–2080.
16. Langheinrich AC, Kampschulte M, Buch T, Bohle RM. Vasa vasorum and atherosclerosis: quid novi? Thromb Haemost. 2007. 97:873–879.
17. Fleiner M, Kummer M, Mirlacher M, et al. Arterial neovascularization and inflammation in vulnerable patients: early and late signs of symptomatic atherosclerosis. Circulation. 2004. 110:2843–2850.
18. Kolodgie FD, Gold HK, Burke AP, et al. Intraplaque hemorrhage and progression of coronary atheroma. N Engl J Med. 2003. 349:2316–2325.
19. Hong MK, Mintz GS, Lee CW, et al. Plaque ruptures in stable angina pectoris compared with acute coronary syndrome. Int J Cardiol. 2007. 114:78–82.
20. Wang JC, Normand SL, Mauri L, Kuntz RE. Coronary artery spatial distribution of acute myocardial infarction occlusions. Circulation. 2004. 110:278–284.
21. Kolodgie FD, Burke AP, Farb A, et al. The thin-cap fibroatheroma: a type of vulnerable plaque: the major precursor lesion to acute coronary syndromes. Curr Opin Cardiol. 2001. 16:285–292.
22. Cheruvu PK, Finn AV, Gardner C, et al. Frequency and distribution of thin-cap fibroatheroma and ruptured plaques in human coronary arteries: a pathologic study. J Am Coll Cardiol. 2007. 50:940–949.
23. Kardys I, Vliegenthart R, Oudkerk M, Hofman A, Witteman JC. The female advantage in cardiovascular disease: do vascular beds contribute equally? Am J Epidemiol. 2007. 166:403–412.
24. Mosca L, Banka CL, Benjamin EJ, et al. Evidence-based guidelines for cardiovascular disease prevention in women: 2007 update. J Am Coll Cardiol. 2007. 49:1230–1250.
25. Stary HC, Chandler AB, Dinsmore RE, et al. A definition of advanced types of atherosclerotic lesions and a histological classification of atherosclerosis: a report from the Committee on Vascular Lesions of the Council on Arteriosclerosis, American Heart Association. Circulation. 1995. 92:1355–1374.
26. Granada JF, Wallace-Bradley D, Win HK, et al. In vivo plaque characterization using intravascular ultrasound-virtual histology in a porcine model of complex coronary lesions. Arterioscler Thromb Vasc Biol. 2007. 27:387–393.
27. Nasu K, Tsuchikane E, Katoh O, et al. Accuracy of in vivo coronary plaque morphology assessment: a validation study of in vivo virtual histology compared with in vitro histopathology. J Am Coll Cardiol. 2006. 47:2405–2412.
28. Nasir K, Shaw LJ, Liu ST, et al. Ethnic differences in the prognostic value of coronary artery calcification for all-cause mortality. J Am Coll Cardiol. 2007. 50:953–960.
29. Fishbein MC, Siegel RJ. How big are coronary atherosclerotic plaques that rupture? Circulation. 1996. 94:2662–2666.
30. Kawasaki M, Takatsu H, Noda T, et al. In vivo quantitative tissue characterization of human coronary arterial plaques by use of integrated backscatter intravascular ultrasound and comparison with angioscopic findings. Circulation. 2002. 105:2487–2492.