Abstract
Prior clinical studies have demonstrated that a family history of coronary artery disease (CAD) is associated with future cardiovascular events. Although there are several Mendelian disorders that are associated with CAD, most common forms of CAD are believed to be multifactorial and the result of many genes with small individual effects. The identification of these genes and their variation would be very helpful for the prediction, prevention, and management of CAD; linkage analysis or candidate gene case-control studies have been largely unsuccessful. On the contrary, recent advances in genomic techniques have generated a large amount of deoxyribonucleic acid (DNA)-based information. The link between CAD and inflammation and biological pathways has been highlighted. In particular, several genome-wide association studies have replicated a novel gene marker on chromosome 9p21. The information gained from genomic studies, in combination with clinical data, is expected to refine personalized approaches to assess risk and guide management for CAD. Genetic risk scores derived from several functional single nucleotide polymorphisms (SNPs) or haplotypes in multiple genes may improve the prediction of CAD. Despite the complexity of CAD genetics, steady progress is expected.
Atherosclerotic coronary artery disease (CAD) is a major health problem worldwide. Intensive studies have been reported and are currently ongoing for appropriate risk assessment, prevention, and treatment of CAD. A small proportion of CAD cases may be attributed to rare, monogenic effects with high penetrance, but most CAD cases are multifactorial in etiology. Development of CAD is only roughly defined by traditional cardiovascular risk factors. In the Framingham Heart Study, a parental history of premature vascular disease was a strong risk factor (adjusted odds ratio=1.7-2.0).1) The INTERHEART study confirmed that a family history of CAD is an important risk factor (adjusted odds ratio=1.5).2) However, because the Framingham risk scoring, which is the most widely used risk assessment tool, does not include family history, thus refining a CAD prediction model is needed. At the same time, with the advances in genomic research, the potential for translating genomic information to clinical practice is greater now than ever before.
During the last 50 years, we have witnessed a remarkable progression of genomic studies, from the initial description of the deoxyribonucleic acid (DNA) double helix by Watson and Crick in 1953 to the completion of the Human Genome Project in 2003. Indeed, genomics is now being used to identify newly discovered pathogens in an effort to stratify patients according to the risk of a disease by genotyping, and to assess the effects and guide therapy. Technological breakthroughs in genetics and genomics have facilitated an understanding of disease pathogenesis.3) The Human Genome Project and International HapMap Project have provided a large amount of DNA-based information. In addition, current technology can assess the expression of thousands of genes from different tissues.
The term, personalized medicine, indicates disease prevention and treatment based on knowledge of individual genetic susceptibilities. By combining information gained through genomic methodologies with traditional methodologies, we expect to advance our ability to assess and treat CAD better. In particular, because DNA-based genetic markers remain unchanged throughout one's life, genetic tests for disease prediction could be performed at a young age to promote early intervention. This review considers basic concepts and approaches, recent genomic studies on CAD, and the clinical implications for personalized medicine.
The 20,000-25,000 protein coding genes that comprise the human genome represent only 30% of its sequence. The remainder is intergenic sequences that may contain elements for the regulation of gene expression. In a typical human gene, 5% of the sequence is coding exons that are partly translated into a protein, and the remainder is composed of introns and regulatory regions. The most common human sequence variations are differences in individual base pairs, termed single nucleotide polymorphisms (SNPs). Other variations consist of short or long repetitions of the same motif, such as mini- and micro-satellites, insertion or deletions, and variants that affect large chromosomal regions.
Genetic variation predisposing to CAD spans from rare and highly deleterious mutations related to Mendelian disease to common polymorphisms with weak effects, that alone or in combination, are associated with the risk of common diseases (common variants-weak effect-common disease model).4) Rare deleterious mutations (e.g., mutations that cause familial hypercholesterolemia) are related to an important risk for CAD; however, the impact at the population level is low. In contrast, frequent polymorphisms, such as the apolipoprotein E polymorphism, may have a population impact despite the weak effect at the individual level. Because polymorphisms have common alleles, numerous combinations of susceptibility alleles at several loci in an individual are possible. Furthermore, some of the combinations of susceptibility alleles can affect the risk for CAD in a way that cannot be predicted from the separate effect of each variant. Since this is the major problem when one characterizes the genetics of complex traits, it is rational and justified to explore systems of genes rather than a single gene.
Almost all polymorphisms identified by association studies have a modest effect, even when combined. Furthermore, there is a marked disparity between the extent of familial aggregation and those collectively accounted for by known variants in common diseases. One emerging hypothesis suggests that a significant proportion of this unknown area of heritability can be explained by low-frequency intermediate penetrance variants. To date, however, these variants have been unyielding to conventional gene-seeking approaches (Fig. 1).5)
Two basic approaches have been used to discover genetic influence on diseases:6) genetic linkage and candidate gene association studies. Genetic linkage studies use genetic and phenotypic data from families. Linkage analysis intends to localize genomic regions that might contain genes influencing a trait. On the contrary, candidate gene association studies compare genotypic frequencies between cases and control groups. A statistical difference in frequencies between the groups suggests that a genotype is associated with the trait. Genetic association studies rely on the existence of linkage disequilibrium (LD) among polymorphic sites which are physically close within the genome. It is referred to as LD when two alleles at different loci occur together on the same chromosome more often than would be predicted. Even if a causal involvement of a polymorphism in the disease is not proven, the association may be suggested by a measured proxy polymorphism in LD. Because of LD identified in most regions of the genome, the combination of alleles at neighboring SNPs (haplotypes) generates less diversity than would be expected. By re-sequencing the genome of 270 individuals from populations from African, Asian, and European ancestries, the HapMap Project has demonstrated a set of SNPs that tag most common haplotypes in humans. This resource can be used to search for polymorphisms associated with susceptibility to common diseases. Recent technological advances have allowed relatively low-cost dense genotyping arrays of 500,000 or more SNPs that cover most of the genome. For complex diseases, by typing hundreds of thousands of variants, genome-wide association studies provide excellent power. Specifically, genome-wide association studies are expected to help to resolve the questions regarding contribution of multiple variants to common diseases.
Until recently, most studies on the genetics of CAD were based on candidate genes. Accordingly, reports of genetic variations in CAD came from established risk factors, such as hyperlipoproteinemia, hypertension, and thrombosis. Nevertheless, relatively few polymorphisms in candidate genes were replicated for CAD.
Disease-causing mutations are defined as rare allelic variants that can markedly increase disease risk. Molecular genetic studies of rare, Mendelian forms of CAD have identified several mutations that cause premature CAD (Table 1). Many of the mutations affect the levels of low density lipoprotein (LDL) and high density lipoprotein (HDL)-cholesterol. Mutations in the LDL receptor gene (LDLR), which is associated with the majority of patients with familial hypercholesterolemia, is a classic example. Gain-of-function and loss-of-function variants of the proprotein convertase subtilisin/kexin 9 (PCSK9) gene, which modulates LDL receptor levels, has been reported to be associated with a reduction of LDL and CAD.7) Both variants are inherited in an autosomal dominant fashion with increased plasma LDL-cholesterol. Tangier disease is characterized by the absence of HDL-cholesterol and low plasma apoA-1. This disease results from mutations in the ATP-binding cassette transporter type 1 (ABCA1) gene and is associated with premature myocardial infarction.
Before the recent introduction of high-density SNP genotyping arrays, linkage analysis, also called positional cloning, using families with multiple affected individuals was considered the most useful way to identify susceptibility loci. Very few genes have been identified from genetic linkage studies, and the lack of replication emphasizes the difficulties in performing and interpreting these studies. The identification of the arachidonate 5-lipoxygenase-activating protein (ALOX5AP) gene encoding 5'-lipoxygenase activating protein (FLAP) represents one success in detecting a novel candidate from a linkage study.8) A few genome-wide linkage studies conducted for subclinical forms of atherosclerotic vascular disease have exhibited linkage evidence on chromosomes 109) and 12.10) Among the observed linkage regions on chromosomes 1, 2, 3, 13, 14, 16, 17, and X, only the linkage region detected by the PROCARDIS consortium on chromosome 17 was clearly replicated.11) It can be inferred from genome-wide linkage studies that there are no CAD loci with strong effects. In the future, putative loci that are implicated from genome-wide linkage scans are likely to help select regions for large-scale association studies.
Candidate gene studies have attempted to investigate variations in genes that are already implicated in the pathophysiology of disease. A large number of candidate gene association studies have been performed for myocardial infarction and atherosclerotic vascular disease, but few studies have been replicated. In addition, because risk ratios have largely been modest in association studies, meta-analyses often have been necessary to obtain statistical significance (Table 2). These analyses were shown in a large number of studies evaluating variants of the apolipoprotein E (APOE),19) plasminogen activator inhibitor 1 (PAI1),22) angiotensin-1 converting enzyme (ACE),20) and methylene tetrahydrofolate reductase (MTHFR) genes.12) Recently, high-throughput genotyping has been used in case-control studies. Variations in lymphotoxin-alpha (LTA),23) gap junction protein, a-4 (GJA4 or connexin 37),24) matrix metalloproteinase-3 (MMP3 or stromelysin-1),24) arachidonate 5-lipolygenase (ALOX5) genes,24) and thrombospondin (THBS) genes25) are associated with myocardial infarction (MI). Among many studies on subclinical atherosclerosis, the 5A/6A polymorphism of the matrix metalloproteinase (MMP3) gene26) revealed a consistent association with carotid intima-media thickness. Two candidate gene association studies have provided evidence for a role of the leukotriene pathway in CAD susceptibility. Promoter variants in the ALOX5AP gene are associated with increased carotid intima-media thickness.27) Another component of the leukotriene pathway which is considered a candidate gene for CAD is the leukotrine A4 hydrolase (LTA4H) gene. A recent study has shown an association between LTA4H and CAD and confirmed the importance of genetic variation in an inflammatory pathway gene in the development of CAD.
Genome-wide association studies that use large numbers of tagging SNPs have now come to replace linkage studies. In 2007, the first generation of genome-wide association studies using high-density, SNP genotyping arrays demonstrated several genetic variants that are significantly associated with CAD (Table 3).28-31) The design of these studies was quite simple. The frequencies of a large number of SNPs genotyped on arrays were compared between cases and controls, and the sites that showed significant differences were then validated in independent samples. There was no prior assumption of candidate genes, rather the search for associated genes throughout the genome was unbiased.32)
Currently, the most robust susceptibility locus for CAD is on chromosome 9p21.28-31) The association of this locus and CAD has been confirmed in independent Caucasian populations comprising greater than 55,000 individuals. In all of these studies, 9p21 was confined to a 58,000 bp region and the coronary risk was independent of traditional risk factors. As there is no known gene coding for a protein in the 58,000-bp region, the neighboring cyclin-dependent kinase inhibitor 2A (CDKN2A), cyclin-dependent kinase inhibitor 2B (CDNK2B), and methylthioadenosine pohosphorylase (MTAP) genes were initially suggested as candidates. The PROCARDIS consortium conducted an analysis of this CAD/type 2 diabetes susceptibility locus on chromosome 9 and demonstrated co-location of a high-risk haplotype with ANRIL, a large antisense non-coding RNA gene.33) It was shown to be expressed in tissues and cells affected by atherosclerosis. ANRIL has now been identified as the most probable candidate gene for the 9p21 locus. The 9p21 58,000 bp region is a target of major research and the mechanism whereby 9p21 causes CAD may imply a new pathway for cardiovascular risk independent of traditional risk factors.
A useful approach to search for candidate gene variants for CAD is to identify biological pathways in the development of CAD. Four interdependent pathways have been described:34) lipoprotein handling, endothelial integrity, arterial inflammation, and thrombosis (Fig. 2). First, as familial hypercholesterolemia is related to a LDL receptor deficiency, abnormal lipoprotein handling is known to promote atherosclerosis. In addition, protective and at-risk alleles of APOE correlate with the effects on LDL-cholesterol.19) PCSK9 loss-of-function mutations can reduce the LDL level and remarkably limit the development of CAD.7) Regarding HDL-cholesterol, rare variants of multiple genes are associated with low HDL levels. Specifically, non-synonymous variants in three genes (ABCA1, APOA1, and LCAT) were associated with the population variance of a low HDL. A series of human studies have shown how a genetic variation of apolipoprotein A-V (APOA5), in combination with apolipoprotein C-III (APOC3), influences triglyceride levels. Several recent studies support the link between the APOA1-APOC3-APOA4-APOA5 cluster and familial combined hyperlipidemia through a mechanism that involves alleles in the APOA5 and APOC3 loci.35) Another positional candidate gene, USF1 (upstream transcription factor 1), has shown evidence of an association with several familial combined hyperlipidemia phenotypes. This gene encodes a transcription factor that is implicated in the regulation of several apolipoproteins and other proteins.36) Secondly, endothelial integrity is an important factor that contributes to the pathophysiology of CAD. The thrombospondin family of extracellular matrix proteins, which affects endothelial cell adhesion and proliferation, was identified to be possibly associated with premature MIs25) and the association has been confirmed by a subsequent study.24) Connexin 37 is a gap junction protein; the C1019T gene variant has been identified to have an association with MIs. Wang et al.37) identified a variation in the myocyte enhancer factor 2a (MEF2A) gene in a family with MIs; the association with MIs has been confirmed by a subsequent study. Third, the arterial inflammation process has been implicated in several studies. LTA, lectin galactoside-binding soluble 2 (LGALS2),23) the leukotriene pathway involving ALOX5AP and LTA4,8) and the histocompatibility factor (MHC2TA) promoter, A168G SNP,38) are known to be related to inflammatory diseases, such as rheumatoid arthritis, multiple sclerosis, and MIs. Fourth, thrombosis has been implicated in CAD in previous studies. In a comprehensive meta-analysis, the 1691A variant of factor V (FV) and the 20210A variant of prothrombin were shown to be associated with CAD. The platelet glycoprotein receptor variants, vesicle-associated membrane protein 8 (VAMP-8) and PAI-1, have also been implicated as risk factors for CAD.39)
During the last decade in Korea, there has been remarkable progress in the field of genomic research. Many genomic studies on CAD were candidate gene association studies.
Several studies involving SNPs with CAD risk factors have been published. Park et al.40) showed a relationship between a cholesteryl ester transfer protein (CETP) Taq1B polymorphism and HDL-cholesterol levels and CAD. I405V, another CETP polymorphism, was reported to have a protective effect against CAD.41) The R219K variant of the ABCA1 gene was associated with high plasma HDL-cholesterol levels and a reduced severity of CAD.42) The APOA5 gene -1131T>C polymorphism has been reported to affect triglyceride levels, lipoproteins, and oxidative stress.43) A polymorphism of the adiponectin gene (AdipoQ), G276T, is associated with metabolic parameters that contribute to CAD.44) The risk for CAD based on specific folate levels required by the different MTHFR C677T genotype has been demonstrated.45) Recently, the association of the ADD1 G460W polymorphism and the risk of CAD was observed.46)
Evidence on the association between genes regulating inflammatory processes and CAD is accumulating. Associations between polymorphisms of endothelial nitric oxide synthase (eNOS),47-49) the NADH/NADPH oxidase p22phox gene,48) the C-1562T polymorphism of gelatinase B promoter, and CAD exist.50) The oxidative stress-related paraoxonase gene Q192R polymorphism is a risk factor for coronary spasm and stenosis.51) A variant of the lipoprotein-associated phospholipase A2 (Lp-PLA2) gene52) and several polymorphisms of chemokine receptor 2 (CCR2) have also been reported to be associated with CAD.53) An association of polymorphisms with the receptor for advanced glycation end products (RAGE) gene and CAD have been shown.54) On the other hand, regulated upon activation, normally T cell-expressed and presumably secreted (RANTES) -403G>A promoter polymorphism have been shown to be related to a reduced risk for CAD.55) Reports on genes related to thrombosis or MIs include: G-33A polymorphism in the thrombomodulin (TM) gene,56) human platelet antigen-3 (HPA-3),57) prostacyclin synthase C1117A,58) prothrombin (PT) T165M, FV R485K polymorphism,59) and pregnancy-associated plasma protein-A (PAPP-A) IVS6+95C allele.60) In recent case-control studies, investigators expanded the association of 9p21 SNPs with CAD in Koreans beyond Caucasian populations.61)
Recent successes in the identification of susceptibility variants have increased confidence that this information can be translated into clinical management. More and more genes and gene products are considered important in assessing patients at risk for CAD. Although single genetic polymorphisms increase individual risk, the integration of information from several polymorphisms can become clinically useful.62)
Markers of inflammation or plaque vulnerability may become part of clinical practice. However, most of the markers that have been discussed have not been validated in large-scale clinical studies. Thus, risk stratification and clinical decision-making may have the form of a combination of analysis of candidate genes, and multiple molecular and clinical factors. In addition, information regarding genomics and phenotypic markers must be considered in the context of an individual's medical history and environmental variables (Fig. 3).
The clinical goal of pharmacogenomics is to deliver the right drug to the right patient by accurate prediction of a therapeutic response and safety before prescription. In prior studies, commonly used cardiovascular medications, such as lipid-lowering agents, antihypertensives, and warfarin, have shown different effects on the basis of individual variation.
Associations between lipid response to HMG-CoA reductase inhibitors and variation in the HMG-CoA reductase gene have been found.63) In hypertension, variation in the alpha-adducin (ADD1) gene was associated with renal sodium reabsorption and salt-sensitive hypertension. Thus, variation in this gene might identify who would benefit more from diuretic therapy. Polymorphisms of the ACE pathway also show the possibility of clinical application of pharmacogenomics. Patients with the ACE DD genotype, which is associated with death or the need for transplantation in chronic heart failure, have shown the greatest benefit from beta-blocker or high-dose ACE inhibitor therapy. Variation in drug metabolism is related to implication for pharmacogenomics. Genetic variants of the enzyme that metabolizes warfarin, cytochrome P-450 2C9 (CYP2C9), and of a pharmacologic target of warfarin, vitamin K epoxide reductase (VKORC1), have been demonstrated to contribute to differences in patients' reponses to warfarin treatment.64)
In recent years, DNA sequence information, sophisticated genetic methods, and advances in statistics and bioinformatics have allowed researchers to identify some variation in genes that modify the risk for CAD. In particular, the consistently replicated locus on 9p21 related to non-coding RNA gene represents a region of high priority for further investigation.
Several limitations of current genomic approaches also need to be reviewed. First, before a personalized medicine for CAD becomes a reality, researchers need to validate novel markers in large independent cohorts, usually achieved by collaborative efforts. Second, unlike conditions such as age-related macular degeneration or diabetes, CAD has a wide range of clinical manifestations. Consequently, CAD cohorts usually have genotypic heterogeneity. Moreover, the lack of a clear definition for control subjects is also a significant problem. Third, although genomic study is to benefit an individual patient, because of the small effect size of most genetic risk factors, it may make only modest influence. Therefore, genetic information can be useful when it identifies candidates for intervention and guides more effective use of interventions.
In the coming years, we expect that the combination of genomic, clinical, and environmental risks will refine personalized approaches to treat potential CAD patients. Because DNA-based genetic markers remain static throughout life, predictive genetic tests could be performed at a young age to facilitate early intervention in high-risk individuals. Genetic tests will add to rather than replace the clinical risk stratification.
Figures and Tables
Fig. 1
Variants with different frequencies, penetrance, and disease susceptibility. GWA: genome-wide association.
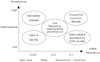
Fig. 2
Biological pathways implicated in CAD pathophysiology and examples of genes. PCSK9: proprotein convertase subtilisin/kexin 9, APOA5: apoliprotein A-V, USF1: upstream transcription factor 1, CAD: coronary artery disease, FLAP: 5'-lipoxygenase activating protein, OX40L: OX40 ligand, MEF2A: monocyte enhancer factor 2a, GP: glycoprotein, LDL: low-density lipoprotein-cholesterol, HDL: high-density lipoprotein-cholesterol, TG: triglyceride, EC: endothelial cell.
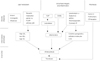
Fig. 3
Combining information from genomic, molecular, and clinical data for personalized medicine in coronary artery disease.
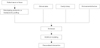
Acknowledgments
This work was supported by grant A000385 from the Ministry of Health and Welfare, Republic of Korea.
References
1. Lloyd-Jones D, Nam BH, D'Agostino RB Sr, et al. Parental cardiovascular disease as a risk factor for cardiovascular disease in middle-aged adults: a prospective study of parents and offspring. JAMA. 2004. 291:2204–2211.
2. Yusuf S, Hawken S, Ounpuu S, et al. Effect of potentially modifiable risk factors associated with myocardial infarction in 52 countries (the INTERHEART study): case-control study. Lancet. 2004. 364:937–952.
3. Miller DT, Ridker PM, Libby P, Kwiatkowski DJ. Atherosclerosis: the path from genomics to therapeutics. J Am Coll Cardiol. 2007. 49:1589–1599.
4. Cambien F, Tiret L. Genetics of cardiovascular disease: from single mutations to the whole genome. Circulation. 2007. 116:1714–1724.
5. McCarthy MI, Abecasis GR, Cardon LR, et al. Genome-wide association studies for complex traits: consensus, uncertainty and challenges. Nat Rev Genet. 2008. 9:356–369.
6. Arnett DK, Baird AE, Barkley RA, et al. Relevance of genetics and genomics for prevention and treatment of cardiovascular disease: a scientific statement from the American Heart Association Council on Epidemiology and Prevention, the Stroke Council, and the Functional Genomics and Translational Biology Interdisciplinary Working Group. Circulation. 2007. 115:2878–2901.
7. Cohen JC, Boerwinkle E, Mosley TH Jr, Hobbs HH. Sequence variations in PCSK9, low LDL, and protection against coronary heart disease. N Engl J Med. 2006. 354:1264–1272.
8. Helgadottir A, Manolescu A, Thorleifsson G, et al. The gene encoding 5-lipoxygenase activating protein confers risk of myocardial infarction and stroke. Nat Genet. 2004. 36:233–239.
9. Lange LA, Lange EM, Bielak LF, et al. Autosomal genenomewide scan for coronary artery calcification loci in sibship at high risk for hypertension. Arterioscler Thromb Vasc Biol. 2002. 22:418–423.
10. Fox CS, Cupples LA, Chazaro I, et al. Genomewide linkage analysis for internal carotid artery intimal medial thickness: evidence for linkage to chromosome 12. Am J Hum Genet. 2004. 74:253–261.
11. Farrall M, Green FR, Peden JF, et al. Genome-wide mapping of susceptibility to coronary artery disease identifies a novel replicated locus on chromosome 17. PLoS Genet. 2006. 2:e72.
12. Klerk M, Verhoef P, Clarke R, Blom HJ, Kok FJ, Schouten EG. MTHFR 677C→T polymorphism and risk of coronary artery disease: a meta-analysis. JAMA. 2002. 288:2023–2031.
13. Boekholdt SM, Sacks FM, Jukema JW, et al. Cholesteryl ester transfer protein TaqIB variant, high-density lipoprotein cholesterol level, cardiovascular risk, and efficacy of pravastatin treatment: individual patient meta-analysis of 13,677 subjects. Circulation. 2005. 111:278–287.
14. Wheeler JG, Keavney BD, Watkins H, Collins R, Danesh J. Four paraoxonase gene polymorphisms in 11212 cases of coronary heart disease and 12786 controls: meta-analysis of 43 studies. Lancet. 2004. 363:689–695.
15. Casas JP, Bautista LE, Humphries SE, Hingorani AD. Endothelial nitric oxide synthase genotype and ischemic heart disease: meta-analysis of 26 studies involving 23028 subjects. Circulation. 2004. 109:1359–1365.
16. Burzotta F, Paciaroni K, de Stefano V, et al. G20210A prothrombin gene polymorphism and coronary ischaemic syndromes: a phenotype-specific meta-analysis of 12034 subjects. Heart. 2004. 90:82–86.
17. Boekholdt SM, Peters RJ, Fountoulaki K, Kastelein JJ, Sijbrands EJ. Molecular variation at the apolipoprotein B gene locus in relation to lipids and cardiovascular disease: a systematic meta-analysis. Hum Genet. 2003. 113:417–425.
18. Di Castelnuovo A, de Gaetano G, Donati MB, Iacoviello L. Platelet glycoprotein receptor IIIa polymorphism PLA1/PLA2 and coronary risk: a meta-analysis. Thromb Haemost. 2001. 85:626–633.
19. Song Y, Stampfer MJ, Liu S. Meta-analysis: apolipoprotein E genotypes and risk for coronary heart disease. Ann Intern Med. 2004. 141:137–147.
20. Agerholm-Larsen B, Nordestgaard BG, Tybjaerg-Hansen A. ACE gene polymorphism in cardiovascular disease: meta-analyses of small and large studies in whites. Arterioscler Thromb Vasc Biol. 2000. 20:484–492.
21. Chiodini BD, Barlera S, Franzosi MG, Beceiro VL, Introna M, Tognoni G. APO B gene polymorphism and coronary artery disease: a meta-analysis. Atherosclerosis. 2003. 167:355–366.
22. Boekholdt SM, Bijsterveld NR, Moons AH, Levi M, Buller HR, Peters RJ. Genetic variation in coagulation and fibrinolytic proteins and their relation with acute myocardial infarction: a systemic review. Circulation. 2001. 104:3063–3068.
23. Ozaki K, Ohnishi Y, Iida A, et al. Functional SNPs in the lymphotoxin-alpha gene that are associated with susceptibility to myocardial infarction. Nat Genet. 2002. 32:650–654.
24. Yamada Y, Izawa H, Ichihara S, et al. Prediction of the risk of myocardial infarction from polymorphism in candidate genes. N Engl J Med. 2002. 347:1916–1923.
25. Topol EJ, McCarthy J, Gabriel S, et al. Single nucleotide polymorphisms in multiple novel thrombospondin genes may be associated with familial premature myocardial infarction. Circulation. 2001. 104:2641–2644.
26. Manolio TA, Boerwinkle E, O'Donnell CJ, Wilson AF. Genetics of ultrasonographic carotid atherosclerosis. Arterioscler Thromb Vasc Biol. 2004. 24:1567–1577.
27. Dwyer JH, Allayee H, Dwyer KM, et al. Arachidonate 5-lipoxygenase promoter genotype, dietary arachidonic acid, and atherosclerosis. N Engl J Med. 2004. 350:29–37.
28. McPherson R, Pertsemlidis A, Kavaslar N, et al. A common allele on chromosome 9 associated with coronary heart disease. Science. 2007. 316:1488–1491.
29. Helgadottir A, Thorleifsson G, Manolescu A, et al. A common variant on chromosome 9p21 affects the risk of myocardial infarction. Science. 2007. 316:1491–1493.
30. Samani NJ, Erdmann J, Hall AS, et al. Genomewide association analysis of coronary artery disease. N Engl J Med. 2007. 357:443–453.
31. The Wellcome Trust Case Control Consortium. Genome-wide association study of 14,000 cases of seven common diseases and 3,000 shared controls. Nature. 2007. 447:661–678.
32. Hirshhorn JN, Daly MJ. Genome-wide association studies for common disease and complex traits. Nat Rev Genet. 2005. 6:95–108.
33. Broadbent HM, Peden JF, Lorkowski S, et al. Susceptibility to coronary artery disease and diabetes is encoded by distinct, tightly linked, SNPs in the ANRIL locus on chromosome 9. Hum Mol Genet. 2008. 17:806–814.
34. Topol EJ, Smith J, Plow EF, Wang QK. Genetic susceptibility to myocardial infarction and coronary artery disease. Hum Mol Genet. 2006. 15:R117–R123.
35. Shoulders CC, Jones EL, Naoumova RP. Genetics of familial combined hyperlipidemia and risk of coronary artery disease. Hum Mol Genet. 2004. 13:R149–R160.
36. Pajukanta P, Lilja HE, Sinsheimer JS, et al. Familial combined hyperlipidemia is associated with upstream transcription factor 1 (USF1). Nat Genet. 2004. 36:371–376.
37. Wang L, Fan C, Topol SE, Topol EJ, Wang Q. Mutation of MEF2A in an inherited disorder with features of coronary artery disease. Science. 2003. 302:1578–1581.
38. Swanberg M, Lidman O, Padyukov L, et al. MHC2TA is associated with differential MHC molecule expression and susceptibility to rheumatoid arthritis, multiple sclerosis and myocardial infarction. Nat Genet. 2005. 37:486–494.
39. Ye Z, Liu EH, Higgins JP, et al. Seven hemostatic gene polymorphisms in coronary disease: meta-analysis of 66155 cases and 91307 controls. Lancet. 2006. 367:651–658.
40. Park KW, Choi JH, Kim HK, et al. The association of cholesteryl ester transfer protein polymorphism with high-density lipoprotein cholesterol and coronary artery disease in Koreans. Clin Genet. 2003. 63:31–38.
41. Cho EY, Bae SJ, Cho HK, et al. Association of cholesteryl ester transfer protein gene polymorphism with serum lipid concentration and coronary artery disease in Korean men. Korean Circ J. 2004. 34:565–573.
42. Ko YG, Cho EY, Park HY, Jang Y, Kim S, Lee JE. Association of R219K polymorphism in the ABCA1 gene with plasma lipid levels and coronary artery disease in Koreans. Korean Circ J. 2003. 33:44–51.
43. Jang Y, Kim JY, Kim OY, et al. The -1131T→C polymorphism in the apolipoprotein A5 gene is associated with postprandial hypertriglyceridemia: elevated small, dense LDL concentrations; and oxidative stress in nonobese Korean men. Am J Clin Nutr. 2004. 80:832–840.
44. Jang Y, Lee JH, Chae JY, et al. Association of the 276G→T polymorphism of the adiponectin gene with cardiovascular disease risk factors in nondiabetic Koreans. Am J Clin Nutr. 2005. 82:760–767.
45. Huh HJ, Chi HS, Shim EH, Jang S, Park CJ. Gene-nutrition interactions in coronary artery disease: correlation between the MTHFR C667T polymorphism and folate and homocysteine status in a Korean populatioin. Thromb Res. 2006. 117:501–506.
46. Cha SH, Kim HT, Jang Y, et al. Association of alpha-adducin Gly460Trp polymorphism with coronary artery disease in a Korean population. J Hypertens. 2007. 25:2413–2420.
47. Choi CJ, Lee KS, Baek SH, et al. Association of endothelial NO synthase gene Glu298Asp polymorphism with acute myocardial infarction. Korean Circ J. 2001. 31:973–981.
48. Lee WH, Hwang TH, Oh GT, Kwon SU, Choi YH, Park JE. Genetic factors associated with endothelial dysfunction affect the early onset of coronary artery disease in Korean males. Vasc Med. 2001. 6:103–108.
49. Kim IJ, Bae J, Lim SW, et al. Influence of endothelial nitric oxide synthase gene polymorphisms (-786T>C, 4a4b, 894G>T) in Korean patients with coronary artery disease. Thromb Res. 2007. 119:579–585.
50. Cho HJ, Chae IH, Park KW, et al. Functional polymorphism in the promoter region of the gelatinase B gene in relation to coronary artery disease and restenosis after percutaneous coronary intervention. J Hum Genet. 2002. 47:88–91.
51. Cho YS, Choi JH, Zhang SY, et al. Relationship of polymorphisms in the oxidative stress related genes-paraoxonase and p22phoxto variant angina and coronary artery stenosis in Korean. Korean Circ J. 2003. 33:104–112.
52. Jang Y, Kim OY, Koh SJ, et al. The Val279Phe variant of the lipoprotein-associated phospholipase A2 gene is associated with catalytic activities and cardiovascular disease in Korean men. J Clin Endocrinol Metab. 2006. 91:3521–3527.
53. Cha SH, Lee JK, Lee JY, et al. Association of CCR2 polyorphisms with the number of closed coronary artery vessels in coronary artery disease. Clin Chim Acta. 2007. 382:129–133.
54. Yoon SJ, Park S, Shim CY, et al. Association of RAGE gene polymorphisms with coronary artery disease in the Korean population. Coron Artery Dis. 2007. 18:1–8.
55. Jang Y, Chae JS, Hyun YJ, et al. The RANTES -403G>A promoter polymorphism in Korean men: association with serum RANTES concentration and coronary artery disease. Clin Sci. 2007. 113:349–356.
56. Park HY, Nabika T, Jang Y, Kwon HM, Cho SY, Masuda J. Association of G-33A polymorphism in the thrombomodulin gene with myocardial infarction in Koreans. Hypertens Res. 2002. 25:389–394.
57. Park S, Park HY, Park C, et al. Association of the gene polymorphisms of platelet glycoprotein Ia and IIb/IIIa with myocardial infarction and extent of coronary artery disease in the Korean population. Yonsei Med J. 2004. 45:428–434.
58. Kim JH, Jeong JO, Choi SW, Seong IW. Association of prostacyclin synthase gene C1117A polymorphism with acute coronary syndrome in Koreans. Korean Circ J. 2004. 34:761–766.
59. Cho EY, Ryu HJ, Bae SJ, et al. Prothrombin T165M and the factor V R485K polymorphism are associated with an increased risk of coronary artery disease in Koreans. Korean Circ J. 2005. 35:429–435.
60. Park S, Youn JC, Shin DJ, et al. Genetic polymorphism in the pregnancy-associated plasma protein-A associated with acute myocardial infarction. Coron Artery Dis. 2007. 18:417–422.
61. Shen GQ, Li L, Rao S, et al. Four SNPs on chromosome 9p21 in a South Korean population implicate a genetic locus that confers high cross-race risk for development of coronary artery disease. Arterioscler Thromb Vasc Biol. 2008. 28:360–365.
62. Song K, Go MJ, Park CM, Lim KS, Jang Y, Kim DK. Analysis of the relative effects of SNPs within a gene to serum lipid profiles using stepwise linear regression. Korean Circ J. 2005. 35:759–765.
63. Chasman DI, Posada D, Subrahmanyan L, Cook NR, Stanton VP Jr, Ridker PM. Pharmocogenetic study of statin therapy and cholesterol reduction. JAMA. 2004. 291:2821–2827.
64. Schwarz UI, Ritchie MD, Bradford Y, et al. Genetic determinants of response to warfarin during initial anticoagulation. N Engl J Med. 2008. 358:999–1008.