Abstract
Background and Objectives
Although human mesenchymal stem cell (hMSC) transplantation has been known to improve ventricular function, the potential proarrhythmic effects have not yet been studied.
Materials and Methods
We monitored the heart rhythm of 6 dogs for 4 weeks after transplantation of hMSC (1×107, epicardial injection) (hMSC group) and in 5 Sham dogs after the injection of the vehicle alone. Cardiac sympathetic nerve sprouting {nerve growth factor (NGF)-β; tyrosine hydroxylase (TH)} and gap junction expression {connexin (Cx) 43} were evaluated in 10 dogs (5 hMSC and 5 Sham) that survived longer than 4 weeks.
Results
The hMSC group expressed higher levels of NGF-β messenger ribonucleic acid (mRNA) (56.0±66.8 fold; p<0.01) with TH+ sympathetic nerves (0.51±0.40 vs. 0.15±0.13% area; p<0.03) than the Sham control. In contrast, the hMSC group expressed lower levels of Cx43 mRNA (0.59±0.29 fold, p<0.001) and Cx43+ (1.64±1.79 vs. 2.12±1.07% area, p<0.001) than the Sham control. The incidences of ventricular fibrillation were 33.3% and 0% in the hMSC group and Sham control, respectively. One of the dogs with ventricular fibrillation (VF) in the hMSC group died suddenly.
Mesenchymal stem cell (MSC) or progenitor cell transplantation has the potential to improve ventricular function, especially if it is administered during the acute stage of myocardial infarction.1-3) In addition, another potential benefit of MSCs is that MSCs may not provoke immune rejection.4-6) However, the safety of MSC transplantation has not yet been clearly established. It has been reported that transplanted MSCs led to poor cell to cell coupling with recipient cardiac cells and promoted potential proarrhythmia.7) But in other studies MSCs were shown to repair conduction blocks8) and express gap junctions.9) Pak et al.10) previously reported that MSC transplantation induces cardiac nerve sprouting in a swine model of myocardial infarction (MI). Cardiac sympathetic hyperinnervation may improve ventricular function. However, it has also been shown to induce lethal arrhythmias.11)12) Based on these studies, we hypothesized that human MSC (hMSC) transplantation may result in sympathetic hyperinnervation, impaired conduction, and ventricular tachyarrhythmias. Therefore, the purpose of this study was to determine the risk of ventricular tachyarrhythmias after hMSC transplantation in a canine model. We also sought to determine how hMSC transplantation affects messenger ribonucleic acid (mRNA) expression of the nerve growth factor (NGF) and connexin (Cx) 43, cardiac sympathetic hyperinnervation, and gap junctional expression.
The protocol used in this study was approved by the Institutional Review Board and the Institutional Animal Care and Use Committee of Anam Hospital of Korea University and conformed to the guidelines of the American Heart Association on human and animal studies.
Human bone marrow, which was used for subsequent transplantation, was harvested from the posterior iliac crest of healthy volunteers. The concentration of nucleated cells in the bone marrow aspirates was 26.7± 2.4 million cells per milliliter. Mononuclear cells were separated from red cells by density gradient centrifugation using Ficoll Paque (density 1.077 g/cm3, American Pharmacia Biotech, Piscataway, NJ, USA). The buffy coat was collected and washed twice by centrifugation at 1,500 rpm for 5 minutes. The cell pellet was re-suspended in culture media at 37℃ and 5% CO2. Cells were initially plated in 25 cm2 vented culture flasks (Costar, Cambridge, MA, USA) at 2×106 cells per milliliter. After 3 days, the media and non-adherent cells were discarded. Cultures were fed biweekly until the flask cultures were confluent. Fibroblast-like cells started forming 4 days after cell culture. Adherent cells were passed three times to eliminate hematopoietic stem cells, and were then progressively expanded to 10 stack cell factories (Nalgene Nunc International, Napervile, IL, USA). The cultured cells were characterized as human mesenchymal stem cells (hMSC) by flow-cytometry (CD29+, CD44+, CD90+, CD105+, CD31-, 34-, and CD45-) and quantitative Reverse Transcription Polymerase chain Reaction (qRTPCR) (Oct-4 gene expression). Prior to epicardial injection, adherent cells were trypsinized, washed and re-suspended.
A total of 11 mongrel dogs (body weight 28-35 kg) were used. We implanted an implantable defibrillator (ICD: Model 7275 VVED-DDDR, Medtronic Inc.) in all dogs using the left internal jugular transvenous approach. An ICD coil electrode was positioned on the right ventricular apex endocardially (Fig. 1A). The ICD generator was implanted in the lateral chest after closing the pericardium. The measured defibrillation threshold in 5 dogs was 15.2±5.3 J. The ventricular fibrillation (VF) was detected when the ventricular rate exceeded 210 bpm. The ICD was followed up at 24 hours, 1 week, and 2 weeks after surgery and kept in the monitoring mode.
We selected 5 sites for hMSC injections on the left ventricular (LV) mid anterior wall in the area between the left anterior descending coronary artery and the left circumflex coronary artery. We marked 5 evenly separated sites that spanned a 30 mm diameter circle with suture materials, and hMSCs (1×107 cells in 1 mL) were injected epicardially at these sites (0.2 mL in each point; Fig. 1B). In the Sham animals, culture media of the same volume as the hMSC was injected. The pericardium was then loosely closed. Dogs that survived more than 4 weeks were sacrificed 32.1±5.7 days after the first surgery. The tissues were then harvested for immunohistochemical and mRNA analyses. One dog in hMSC group died suddenly; therefore, its cardiac tissue was not included in the histological and mRNA analyses due to the time delay before necropsy.
The documented arrhythmic events in ICD were manually analyzed. 3-dimensional isochronal maps were generated by LV endocardial contact bipolar mapping (70-100 points, during high right atrial pacing, pacing cycle length 500 ms) using a NavX system (St. Jude Medical Inc. Minnetonka, MN, USA) in 2 animals injected with hMSC before sacrifice.
Hearts were fixed with 4% formalin. They were then sampled, paraffin embedded and processed routinely for immunohistological examinations.11) We performed tyrosine hydroxylase (TH) staining to detect sympathetic nerves, Cx43 immunostaining to detect gap junctions, and human nucleolin (hNC) staining to detect hMSC. 5 µm thick transmural sections that were formalinfixed and paraffin embedded were stained for TH, Cx43, and hNC using a modified immunocytochemical AB complex method as described previously.10) Primary antibody concentrations were 1 : 200 for TH (Abcam Inc., Cambridge, UK), 1 : 100 for Cx43 (Chemicon International Inc., USA.), and 1 : 400 for hNC (Abcam Inc., Cambridge, UK), respectively. The immunoreactive products were visualized by incubating the tissue sections in a DAKO Liquid DAB Substrate Chromogen system (Dako, Copenhegen, Denmark) and counterstained with diluted hematoxylin.
mRNA expression of NGF-β and Cx43 was quantified using qRT-PCR with a Perkin-Elmer ABI Prism 7,700 sequence detection system. qRT-PCR was performed with a AmpliTaq Gold polymerase (Perkin-Elmer ABI) and 20 ng of cDNA per reaction (Taqman Gene Expression Assays, Applied Biosystem Inc.).13) The cycle threshold (Ct) values for 18s rRNA and the mRNA of interest were compared and calculated using sequence detector software (Perkin Elmer ABI). Relative transcript levels were calculated as χ=2_△△Ct where ΔΔCt=ΔE-ΔC and ΔE=Ct experimental-Ct 18s rRNA; ΔC=Ct control-Ct 18s rRNA. For comparison, the Ct-value of the sham group was used as the normal control.
TH slides were evaluated for nerve density. The entire slide was scanned with a 100× objective and 4 microscopic fields with the highest intra-myocardial nerve densities at the right upper, right lower, left upper and left lower quadrants of each slide were selected. In each of the four fields, the cardiac nerves were identified as THimmunostaining-positive fibrillar structures, between myocardial cells, when they were longer than 10 µm and stained brown (RGB values: Red 22-125, Green 4-77, and Blue 4-55). In addition, the digital images at 400× magnification were saved. The gap junctions located on the cell membranes of each myocardial cell were identified by Cx43-immunostaining-positive linear structures. To evaluate the density of the gap junctions, we obtained 4 digital pictures at each quadrant of the tissue slide at 400× magnification. The percent area of the nerve cells and gap junctions {DAB responsive area/the area of digital image (1.41 µm2)×100 (%)} were calculated from the immuno-staining using Image Pro software (MediaCybernetics Inc.) by a single investigator who was blinded to the groups.14) The density of the cardiac sympathetic nerve or gap junctions were calculated by dividing the nerve or gap junction areas by the total area examined (% area), respectively. All values were expressed as mean±SD. Between-group comparison was carried out with student's t-test for the continuous variables, and Fisher's exact test for the non-continuous variables. Statistical significance was defined as a p<0.05.
Table 1 summarizes the incidences of VF and sudden cardiac death (SCD). Among the 6 animals transplanted with hMSC, one dog died suddenly 6 days after surgery. The ICD recorded VF at the time of the SCD episodes. In the necropsy, no other cardiovascular reasons for SCD other than VF were found. We programmed the ICD to the monitor mode to only prevent shock delivery. This allowed us to determine if some VF episodes could spontaneously terminate without shocks. In the hMSC group another dog also manifested non-sustained VF; however this dog did not die suddenly (Fig. 1C). The ICD electrograms revealed spontaneous VF in 2 of the 6 dogs (33.3%). In comparison, no VF or SCD episodes were documented in the sham control group (0/5; 0%).
For the immunohistochemical analyses, hNC positive hMSC were observed in the myocardium 4 weeks after injection (Fig. 2A). As a result of inflammation after surgery there were some polymorphonuclear cells at the injection sites. However, there was no evidence of lymphocytic infiltration or immune rejection (Fig. 2B). The survival rate of hMSC was approximately 3%, 4 weeks after myocardial injection.
qRT-PCR for mRNA expression of NGF-β was performed on tissue removed from the area of the hMSC injection. NGF-β mRNA expression in the hMSC group was 56.0±66.8 (p<0.01) fold higher than in the Sham group (Fig. 3A). TH-positive sympathetic cardiac nerve densities in the areas of hMSC injection were determined from 320 digital images at areas of highest cardiac nerve density in 20 slides. Fig. 3C and D show the distributions of TH-positive cardiac sympathetic nerves in each group. Hearts from the hMSC group showed very high sympathetic nerve densities. There were actively arborizing large nerves and TH positive ganglia. By contrast, dogs in the sham control group rarely had TH positive sympathetic nerve twigs. The calculated % areas of the cardiac sympathetic nerves in the tissue of hMSC group (0.51±0.40%) was significantly higher than those of the Sham group (0.15±0.13%, p<0.03; Fig. 3B).
qRT-PCR for mRNA expression of Cx43 was performed on tissue removed from the area of hMSC or vehicle injection. Cx43 expression in the hMSC group was 0.59±0.29 fold lower than that of the Sham group (p<0.001, Fig. 4A). After quantification of gap junctions from 320 digital images in 20 slides stained with Cx43 immunostaining the density of gap junction was found to be lower in hMSC group compared with the Sham control group (Fig. 4C and D). The % area of Cx43 positive gap junctions in hMSC group was significantly lower than that of the Sham group, which was consistent with the Cx43 mRNA expression (1.64±0.79% vs. 2.12±1.07%. p<0.001; Fig. 4B). Fig. 5 displays the remarkable conduction delay the we observed at the hMSC transplantation area in the isochronal map of LV endocardial contact mapping.
In this study, we successfully transplanted and engrafted hMSC in a canine model without any immune rejection. However, the hMSC transplantation did increase NGF-β expression and cardiac sympathetic hyperinnervation, and reduced the expression of Cx43 mRNA and gap junction density. In addition, VF and SCD, which are associated with potential proarrhythmia, were encountered in this model after hMSC transplantation.
Cellular transplantation is an emerging new technology that can be potentially used for the treatment of heart failure. MSCs have several merits compared with other cell sources in relation to cardiac cell therapy, including no ethical problems, better cellular coupling with recipient cells compared to skeletal myoblast,8)15) and immunological privilege.4-6)16) In addition, the immunologically privileged status of MSCs may extend to xenograft settings.17) Several clinical trials have shown that the use of hMSC precursors were effective in managing heart failure especially if used during the acute stage of myocardial infarction.1)2)18-20) However, the improvement of LV function has been shown to be minimal and may not be long lasting,21) and the mechanism of action is still unknown.
Menasche et al.22) reported that 4 out of 10 patients with ischemic cardiomyopathy, who received transplanted autologous skeletal myoblasts, experienced late sustained VT. These findings suggest that cellular transplantation has the potential to develop into cardiac arrhythmia. The observed arrhythmias may be due to the lack of gap junctions expressed in transplanted skeletal myoblasts, which results in large excitable gaps in the recipient heart and aberrant electrical activity.23) Although Beeres et al.8) demonstrated that MSCs express gap junctions with sufficient coupling. Since the cells are not excitable, they will be unable to carry the action potential over larger distances and will be unlikely to support contractility due to the absence of a well-developed functional contractile apparatus. Chang et al.7) also reported potential arrhythmia in an optical mapping study of co-cultured MSC and ventricular myocytes. This occurred as a result of reduced conduction velocity, which was due to increased tissue heterogeneity. This was observed despite the presence of functional gap junctions that involved MSCs. Consistent with previous studies, we observed a reduction in both gap junctional expression and the conduction velocity at the sites of hMSC transplantation. Even though gap junctions exist, poor differentiation to functional cardiomyocyte or insufficient ion channel expression may induce conduction delay, localized reentry, and proarrhythmia.
Several recent clinical trials showed that intracoronary injection of bone marrow derived stem cells resulted in no proarrhythmia in humans.1)2)18-20) However, endothelial progenitor cells were used in these studies, and the average LV ejection fractions was relatively high (41.0 to 48.9%), indicating that the enrolled patients did not all have significant LV dysfunction. Therefore, these studies do not rule out the possibility of stem cell proarrhythmia in hearts with more severe organic diseases. Compared with previous clinical studies that have used intra-coronary infusion of bone-marrow-derived cells, our model produced a high density of hMSC that were localized within 3 cm around the pacing electrode. The localized high density hMSC may have exacerbated the problems of cell to cell conduction and proarrhythmia.24) However, intra-coronary infusion of hMSC is not feasible due to the large size of hMSC and the risk of coronary embolism.
There are three possible cellular mechanisms by which MSCs contributed to the restorative action and improvement of ventricular function. The first potential mechanism is that the MSCs differentiate into functioning cardiac structures such as cardiomyocytes,25-31) vessels,26)28) fibroblasts,25)26) or nerve.32) The second is that the MSCs change their function by fusing with recipient cells.33)34) Finally the third potential mechanism is that stem cells induce angiogenesis or sympathetic nerve sprouting by paracrine action.35)
Sympathetic nerve sprouting, and its heterogeneity, is a known substrate for lethal ventricular arrhythmias.11-13) Sympathetic nerve activation exerts significant effects on electrophysiologic properties such as automaticity, triggered activity, refractoriness, and conduction velocity of myocardial cells.36)37) Although we have previously reported that enhanced nerve sprouting occurs in a MSC transplanted swine model,10) sympathetic hyper-innervation and its mechanism were not proven at that time. In this study, we documented the elevation of NGF-β expression and sympathetic hyperinnervation in a canine model of transplanted hMSC.
There were multiple other factors that could have been responsible for VF in hMSC transplanted hearts that were independent of sympathetic hyperinnervation or reduced gap junction. However, sympathetic hyperinnervation and reduced gap junctions after hMSC transplantation with EpiP are novel findings, and might be contributing factors that cause VF. Although a pathologist determined no evidence of immune rejection, we cannot absolutely exclude undetected proarrhythmic effects from the cellular xenograft. We did not perform long-term monitoring of the experimental animals.
Figures and Tables
Fig. 1
Experimental procedures. A: the ICD lead was positioned using the left internal jugular venous approach, and the position of the ICD coil electrode was confirmed by fluoroscopic imaging. B: hMSC injection sites were marked with suture material, and the hMSCs were transplanted by direct epicardial injection. C: the ICD electrogram revealed an episode of spontaneously induced non-sustained VF. This dog survived for 4 weeks. ICD: implantable defibrillator, hMSC: human mesenchymal stem cell, VF: ventricular fibrillation.
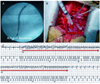
Fig. 2
Human nucleoline (hNC) immunostaining of ventricular tissue close to the area of hMSC injection. A: the brown colored nucleus represents hNC positive human cells (hMSC) at 200× magnification. B: the 400× magnification high power field shows no evidence of immune rejection (no lymphocytic infiltration, but polymorphonuclear cells due to surgical inflammation).
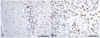
Fig. 3
A: mRNA expression levels of NGF-β was significantly higher in the hMSC group compared with Sham control group. B: calculated % area of TH positive (sympathetic) nerves was significantly higher in the hMSC transplanted tissues compared with the Sham group. C and D: tyrosine hydroxylase (TH) immunostaining of ventricular tissue close to the area of hMSC injection at 200× magnification. The hMSC group shows significant sympathetic hyperinnervation after 4 weeks of survival. By contrast, no significant increase in sympathetic nerves was observed in the Sham group. qRT-PCR: quantitative Reverse Transcription Polymerase chain Reaction, NGF-β: nerve growth factor-β, hMSC: human mesenchymal stem cell.
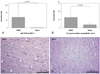
Fig. 4
mRNA expression levels. A: Cx43 was significantly lower in the hMSC group compared with Sham control group. B: calculated % area of Cx43 positive gap junction was significantly lower in the hMSC group compared with the Sham group. C and D: Cx43 immunostainings of ventricular tissue close to the area of hMSC injection at 200× magnification. The density of Cx43 positive gap junction is lower in hMSC transplanted tissue compared with the Sham after 4 weeks of survival. qRT-PCR: quantitative Reverse Transcription Polymerase chain Reaction, hMSC: human mesenchymal stem cell.
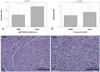
Fig. 5
Isochronal map of LV endocardium during high right atrial pacing (cycle length 500 ms) in right anterior oblique view and anterior posterior cranial view in animals that were transplanted with hMSC. As showed in the color scale bar, the impulse conducts from the white color to the purple color, and the earliest activation site is a septum. However, there is a significant conduction delay at the LV anterior wall (white dotted circle) where the hMSC was injected and Cx43 expression was reduced. LV: left ventricle, hMSC: human mesenchymal stem cell.
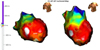
Acknowledgments
This work was supported by a The Korean Society of Circulation
Industry-University Cooperation Grant (2007) and a 21C Frontier
R&D Grant #SC3140 (2007) to H-N Pak. We thank Dr. Peng-Sheng
Chen for his kind teaching and comments, Mr. Do Young Rhiu for
technical assistance, and Mr. John Martin for his linguistic assistance.
References
1. Schachinger V, Erbs S, Elsasser A, et al. Intracoronary bone marrow-derived progenitor cells in acute myocardial infarction. N Engl J Med. 2006. 355:1210–1221.
2. Assmus B, Honold J, Schachinger V, et al. Transcoronary transplantation of progenitor cells after myocardial infarction. N Engl J Med. 2006. 355:1222–1232.
3. Lim D. Stem cells for cardiovascular disease. Korean Circ J. 2004. 34:435–440.
4. Tse WT, Pendleton JD, Beyer WM, Egalka MC, Guinan EC. Suppression of allogeneic T-cell proliferation by human marrow stromal cells: implications in transplantation. Transplantation. 2003. 75:389–397.
5. Le Blanc K, Tammik C, Rosendahl K, Zetterberg E, Ringden O. HLA expression and immunologic properties of differentiated and undifferentiated mesenchymal stem cells. Exp Hematol. 2003. 31:890–896.
6. Bartholomew A, Patil S, Mackay A, et al. Baboon mesenchymal stem cells can be genetically modified to secrete human erythropoietin in vivo. Hum Gene Ther. 2001. 12:1527–1541.
7. Chang MG, Tung L, Sekar RB, et al. Proarrhythmic potential of mesenchymal stem cell transplantation revealed in an in vitro coculture model. Circulation. 2006. 113:1832–1841.
8. Beeres SL, Atsma DE, van der Laarse A, et al. Human adult bone marrow mesenchymal stem cells repair experimental conduction block in rat cardiomyocyte cultures. J Am Coll Cardiol. 2005. 46:1943–1952.
9. Valiunas V, Doronin S, Valiuniene L, et al. Human mesenchymal stem cells make cardiac connexins and form functional gap junctions. J Physiol. 2004. 555:617–626.
10. Pak HN, Qayyum M, Kim DT, et al. Mesenchymal stem cell injection induces cardiac nerve sprouting and increased tenascin expression in a Swine model of myocardial infarction. J Cardiovasc Electrophysiol. 2003. 14:841–848.
11. Cao JM, Chen LS, KenKnight BH, et al. Nerve sprouting and sudden cardiac death. Circ Res. 2000. 86:816–821.
12. Cao JM, Fishbein MC, Han JB, et al. Relationship between regional cardiac hyperinnervation and ventricular arrhythmia. Circulation. 2000. 101:1960–1969.
13. Zhou S, Chen LS, Miyauchi Y, et al. Mechanisms of cardiac nerve sprouting after myocardial infarction in dogs. Circ Res. 2004. 95:76–83.
14. Liu YB, Wu CC, Lu LS, et al. Sympathetic nerve sprouting, electrical remodeling, and increased vulnerability to ventricular fibrillation in hypercholesterolemic rabbits. Circ Res. 2003. 92:1145–1152.
15. Kessler PD, Byrne BJ. Myoblast cell grafting into heart muscle: cellular biology and potential applications. Ann Rev Physiol. 1999. 61:219–242.
16. Liechty KW, MacKenzie TC, Shaaban AF, et al. Human mesenchymal stem cells engraft and demonstrate site-specific differentiation after in utero transplantation in sheep. Nat Med. 2000. 6:1282–1286.
17. Saito T, Kuang JQ, Bittira B, Al-Khaldi A, Chiu RC. Xenotransplant cardiac chimera: immune tolerance of adult stem cells. Ann Thorac Surg. 2002. 74:19–24.
18. Kang HJ, Kim HS, Zhang SY, et al. Effects of intracoronary infusion of peripheral blood stem-cells mobilised with granulocyte-colony stimulating factor on left ventricular systolic function and restenosis after coronary stenting in myocardial infarction. Lancet. 2004. 363:751–756.
19. Lunde K, Solheim S, Aakhus S, et al. Intracoronary injection of mononuclear bone marrow cells in acute myocardial infarction. N Engl J Med. 2006. 355:1199–1209.
20. Janssens S, Dubois C, Bogaert J, et al. Autologous bone marrowderived stem-cell transfer in patients with ST-segment elevation myocardial infarction: double-blind, randomised controlled trial. Lancet. 2006. 367:113–121.
21. Meyer GP, Wollert KC, Lotz J, et al. Intracoronary bone marrow cell transfer after myocardial infarction: eighteen months' follow-up data from the randomized, controlled BOOST (BOne marrOw transfer to enhance ST-elevation infarct regeneration) trial. Circulation. 2006. 113:1287–1294.
22. Menasche P, Hagege AA, Vilquin JT, et al. Autologous skeletal myoblast transplantation for severe postinfarction left ventricular dysfunction. J Am Coll Cardiol. 2003. 41:1078–1083.
23. Abraham MR, Henrikson CA, Tung L, et al. Antiarrhythmic engineering of skeletal myoblasts for cardiac transplantation. Circ Res. 2005. 97:159–167.
24. Fukushima S, Varela-Carver A, Coppen SR, et al. Direct intramyocardial but not intracoronary injection of bone marrow cells induces ventricular arrhythmias in a rat chronic ischemic heart failure model. Circulation. 2007. 115:2254–2261.
25. Shake JG, Gruber PJ, Baumgartner WA, et al. Mesenchymal stem cell implantation in a swine myocardial infarct model: engraftment and functional effects. Ann Thorac Surg. 2002. 73:1919–1925.
26. Kocher AA, Schuster MD, Szabolcs MJ, et al. Neovascularization of ischemic myocardium by human bone-marrow-derived angioblasts prevents cardiomyocyte apoptosis, reduces remodeling and improves cardiac function. Nat Med. 2001. 7:430–436.
27. Orlic D, Kajstura J, Chimenti S, et al. Bone marrow cells regenerate infarcted myocardium. Nature. 2001. 410:701–705.
28. Fuchs S, Baffour R, Zhou YF, et al. Transendocardial delivery of autologous bone marrow enhances collateral perfusion and regional function in pigs with chronic experimental myocardial ischemia. J Am Coll Cardiol. 2001. 37:1726–1732.
29. Lim SY, Jeong MH, Ahn YK, et al. The effects of mesenchymal stem cells transduced with Ark in a porcine myocardial infarction model. Korean Circ J. 2005. 35:734–741.
30. Piao H, Youn TJ, Kwon JS, et al. Cellular cardiomyoplasty using bone marrow derived mesenchymal stem cells transplantation in post myocardial infarction heart failure. Korean Circ J. 2004. 34:1113–1121.
31. Yang KM, Park CS, Jang SW, et al. Effect of adult bone marrow stem cells on myocardial regeneration in doxorubicin-induced mouse cardiomyopathy. Korean Circ J. 2008. 38:110–118.
32. Woodbury D, Schwarz EJ, Prockop DJ, Black IB. Adult rat and human bone marrow stromal cells differentiate into neurons. J Neurosci Res. 2000. 61:364–370.
33. Weimann JM, Johansson CB, Trejo A, Blau HM. Stable reprogrammed heterokaryons form spontaneously in Purkinje neurons after bone marrow transplant. Nat Cell Biol. 2003. 5:959–966.
34. Alvarez-Dolado M, Pardal R, Garcia-Verdugo JM, et al. Fusion of bone-marrow-derived cells with Purkinje neurons, cardiomyocytes and hepatocytes. Nature. 2003. 425:968–973.
35. Rehman J, Li J, Orschell CM, March KL. Peripheral blood "endothelial progenitor cells" are derived from monocyte/macrophages and secrete angiogenic growth factors. Circulation. 2003. 107:1164–1169.
36. Martins JB, Zipes DP. Effects of sympathetic and vagal nerves on recovery properties of the endocardium and epicardium of the canine left ventricle. Circ Res. 1980. 46:100–110.
37. Opthof T, Misier AR, Coronel R, et al. Dispersion of refractoriness in canine ventricular myocardium: effects of sympathetic stimulation. Circ Res. 1991. 68:1204–1215.