Abstract
Background and Objectives
The ability to study microvessels of a beating heart in real time at the level of the capillary is essential for research. However, there are no proven methods currently available to achieve this. The conventional absorption-contrast agents have limitations for studying capillaries. Microangiography with using synchrotron phase-contrast X-ray technology and no contrast agent has recently been reported on. We tried to verify this previous report, and we wanted to visualize the microvessels of a rat heart using air as a contrast agent.
Materials and Methods
We made the Langendorff apparatus in a hutch of the Pohang Accelerator Laboratory. The images were obtained with a white beam and a monochromatic beam. The visual images were magnified using 3× and 20× optical microscope lenses, and the images were captured with a charge-coupled device camera.
Results
We could not duplicate the previously reported findings in which microvessels were visualized without the use of contrast agent. But with using air as a contrast agent, the microvasculature of rat hearts was clearly identified at a spatial resolution of 1.2 µm. Air being absorbed inside a capillary was also observed. Vessels under 10 µm diameter were unable to be visualized with using iodine as a contrast agent.
Conclusion
Phase contrast imaging already allows spatial resolution of 1 µm, which is enough to inspect capillaries. We were able to obtain images of cardiac capillaries with using air as a contrast agent. Yet air has the fatal limitations in that it causes embolism and ischemia. A more suitable contrast agent or imaging method needs to be developed in order to study the microvessels of a beating heart.
Performing phase-contrast imaging is based on the different refractive indexes of objects. A well-collimated X-ray beam is slightly deviated from the edges between different objects, and this produces a sharply enhanced image. To obtain a phase-contrast effect, a parallel beam of a sufficiently small source size and an adequate wavelength is required and it is possible to achieve this effect by using synchrotron radiation as a light source at the accelerator laboratory.1)
Conventional X-ray imaging is based on the varying absorption of X-rays between different tissues. Since there is very little difference in absorption between the vessel wall and the surrounding tissues, a contrast agent with high absorption properties is required to identify the vessels. Yet the absorption contrast method has some disadvantages in obtaining capillary level microangiography images because contrast agents have high osmolality, high viscosity and they cause allergic reactions; further, they cause overlapping effects of small vessels and they can become diluted. Additionally, the current absorption contrast techniques face a fundamental problem in that the changes inside the vessels themselves cannot be visualized. In such organs as the heart, which is thick and composed of many overlapping vessels, the X-rays tend to reflect much of the information that the rays pass through and so the use of iodine as a contrast agent does not allow for the study of specific vessels. Studies concerned with synchrotron imaging are rapidly increasing in number and quality, but the majority of these studies have used an iodine contrast agent, and they dealt with microvessels that had a diameter greater than 20 µm.2-4)
To overcome the limitations of using an absorption contrast agent, several research groups have examined another vascular imaging technique.5-7) Their research suggested the possibilities of performing microangiography without the use of a contrast agent,6) and the vessels of the eye and the auricle regions in live rats were visualized at a spatial resolution of 10 µm.
Dynamic observation of a capillary in a beating heart in real time has not yet been achieved. If we were able to do this, it would be a new unreported technology and a groundbreaking tool to study the vasculature changes associated with transplant rejection, cardiac ischemia, the effect of drugs and so on.
The Langendorff apparatus was built in a hutch of the Pohang Accelerator Laboratory (PAL). Two month old male Sprague-Dawley rats weighing approximately 250 g each were then anesthetized by an intraperitoneal injection of Zoletil 30 mg/kg (Virbac, France) and Xylazine 12 mg/kg (Bayer AG, Germany). A set amount of Krebs solution (NaCl 118 mM, KCl 4.7 mM, CaCl2 2.5 mM, MgSO4 1.2 mM, NaHCO3 25 mM, KH2PO4 1.2 mM, glucose 11.5 mM) was stored in the upper reservoir of the Langendorff circuit and this was infused with a mixture of 95% O2/5% CO2 (pH 7.4) gas 15 minutes before the experiment. A heater in the Langendorff circuit heated the solution to 38℃ before it was pumped into the rat heart (Fig. 1). We then dissected the rats using a midline sternal incision, harvested the hearts and quickly perfused the coronary arteries of the hearts with Krebs solution through a 2 mm probe via the ascending aorta. The flow rate of Krebs solution was 10 mL/min. The rat heart was secured onto the experimental board while the Krebs solution was being continuously infused. The X-rays coming through the beam line passed through the heart and scintillator to form an image via the charge-coupled device (CCD) camera.
Because the Langendorff model involves a beating moving heart, there is a need to limit this movement in order to study the microvessels of a specific small area. In order to do this, an attempt was made initially to harvest a small part of the heart. However, it was found to be very difficult to harvest a sample while leaving the coronary artery supply intact. Thus, attempts were made to remove the calcium or to add potassium to the Krebs solution. Once the experiment was repeated many times it was possible to image approximately the same area regardless of the contractions and so it was possible to study the microvessels of a certain area of the heart in its beating state.
Iodine (Telebrix, Guerbet, France) and air were used as contrast agents. The contrast agent was infused into the heart with using a three-way connector that was set just before the probe.
The microangiographic images were collected using a beam line 1B2/microprobe and a beam line 7B2/microprobe at the PAL in Korea.8) The energy level of electrons was 2.5 GeV with a beam current of about 180 mA just after starting the electron beam.
For the 1B2 beamline, a double multilayer monochromator that consisted of a pair of W/B4C multilayers was used; the unit energy was set at 8 KeV, and the length of the beamline from the energy source was about 25 m. The X-ray image was converted into a visual signal by using a 20 µm thick CsI scintillation crystal. We magnified the images using an optical lens, and the spatial resolution was approximately 1.2 µm when a ×20 lens was used. The CCD camera had an active area of 6.4×4.8 mm2 and this area consisted of 768×494 pixels. When we used a ×20 lens, a 320×240 µm2 area was covered and each pixel corresponded to approximately 0.5 µm. The output of the CCD camera was transferred to a VCR, and this data was later converted into digital files.
An unmonochromatized white beam was used for the 7B2 beamline, and the total length of the beamline was about 35 m. We used a 250 µm thick cleaved CdWO4 single crystal as a scintillator. The images on the CCD camera were directly transferred to a computer that was attached to the camera, and the number of pixels ranged up to 1,280×1,024 with a 14 bit gray scale. To limit the X-ray absorption, a single image was taken within 50 ms. To minimize the radiation damage, a shutter was installed that could reduce the exposure time to milliseconds. In most cases an exposure time of 50 ms was used in the experiments involving the rat hearts. For both beamlines, we placed the specimens 25 cm from the detector to achieve the best contrast.
This study was approved by the Institutional Animal Care and Use Committee (IACUC) of the Clinical Research Institute, Seoul National University Hospital. This facility has been accredited by the International Association for the Assessment and Accreditation of Laboratory Animal Care.
Although the same procedure as described by Hwu et al.1) was repeated in this experiment, phase-contrast visualization of the microvessels was not possible. We found that the microvessels became visible with prolonged exposure to a white beam, and this was due to radiation induced tissue damage and intravascular evaporation changes (Fig. 2). We obtained the gross and pathologic images of the damaged rat hearts after they were continuously exposed to a white beam (Fig. 3).
The images obtained with using iodine as a contrast agent primarily relied on the absorption contrast effect. It was technically easy to obtain the images of the cardiac vasculature with good reproducibility, yet a highly concentrated solution of contrast agent was needed. Moreover, it was difficult to visualize the microvessels that were less than 20 µm in diameter because of dilution of the contrast agent, the dark background and overlapping of other vessels (Fig. 4). We found that the conventional contrast technique using iodine as a contrast agent was not adequate to observe capillaries.
When 0.5 cc of air was infused into the coronary arteries in the Langendorff circuit, the absorption contrast effect produced by the air embolus allowed visualization of the coronary arteries and their associated microvessels that were less than 20 µm in diameter with good spatial resolution (Figs. 5 and 6). Because air as a medium allows refraction of X-rays between it and the surrounding tissues, it brings about a strong phase contrast effect that allows the visualization of microvessels at the level of the capillary (Fig. 5). We also took the images of a rat toe to reveal the effect of air at places other than hearts (Fig. 6), and we could observe arteries, veins and the capillary networks.
Throughout the experiments, the movement of the air emboli was carefully studied; reabsorption of microscopic air emboli in the capillaries was observed. Though large amounts of intravascular air passed through the capillaries into the venous circulation, the microscopic air emboli were slowly reabsorbed into the surrounding tissues at the level of the capillary.
Imaging techniques that use synchrotron radiation as a light source are being developed at a rapid rate, and we can now easily obtain the X-ray presentations, the angiographic findings, the 3-dimensional reconstruction images etc of a 100 g rodent.3)7-10) The fact that we can obtain these images from rodents has important implications. Preclinical testing to examine the effects of ischemia, reperfusion and neovascularization on a specific oncogene, assessing the development of small collateral arteries after ischemia2)11) etc, can be freely carried out.
Thus the large costs and time associated with testing larger animals were avoided in our study and the tests were able to be repeated many more times while being cost effective. Furthermore, although this type of study has not been attempted on different mammals, on species with genetic mutations or on knockout species, the fact that this imaging technique has been successfully performed in rodents will encourage further experiments and investigations in this area.4)7)
Most of the studies in the current literature have relied on the absorption contrast effect of iodine as a contrast agent, and we proved that this conventional contrast agent is not appropriate to inspect microvessels that are less than 20 µm diameter. There have been a few studies that have tried to obtain synchrotron microangiographic images with using other imaging techniques and without conventional contrast agents.5)6) There has been a recent report in the literature of good phase-contrast imaging that was carried out with using normal saline;5) however, this involved equipment that was different from the ones used in the PAL and the studies used specially designed equipment such as a monolithic X-ray interferometer, which is currently unavailable in Korea. Vessels over 30 µm in diameter are currently able to be seen with that method. We have presented in our study the dynamic images of true capillaries of a beating heart for the first time in the world, and the spatial resolution was the finest that has ever been achieved. This study used only synchrotron radiation equipment and optical microscopic lenses without the use of any other specialized equipment and so this allows for superior reproducibility.
The paper by Hwu et al.6) reported on synchrotron microangiography with using no contrast agent; that paper was met with keen interest by members of the medical community. In their report, they said that it was possible to image the microscopic details of moving blood vessels in different live animals. Moreover, the study also pointed out that it will eventually be possible to obtain capillary level images of the microvasculature in real time without any contrast agent for clinical use.
However, by performing repeated experiments in this study, it was found that the results reported by Hwu et al. in their study were likely due to a phase-contrast effect by the air that was produced during the experimental process. The process used in Hwu et al.6) study, which was identical to the one used in this study, and the process involved using high energy 7B2 beamlines without any device to monochromatize the X-ray beam. In these conditions, the high-energy caused the vaporization of fluid inside the blood vessels and the resulting air produced a phase-contrast effect. As the same areas were repeatedly imaged throughout the study, it was observed that there is a relationship between the radiation exposure time, vessel twisting and the air contrast effect (Fig. 2). The fact that we could not observe this phenomenon on the 1B2 beamline with using a monochromatized beam is also reliable evidence. Hwu et al.6) in their study first used iodine contrast to image the vessels, and then they waited a few minutes for the contrast to wash out before taking the next set of images. It is believed that the vaporization process took place during the first imaging process. Although contrast was not intentionally injected into the specimens in Hwu et al.6) study, vaporization occurred due to the high energy beamline and this produced air that in turn acted as a contrast agent. So it is difficult to claim that microangiography at the level of the capillary without the use of a contrast agent is currently possible.
Concerning the radiation hazard, it was suggested that the intensity of 2.0×1010 photons mm-2 s-1 was satisfactory for clinical use.12) This irradiation dose is about 2.8-fold larger than that delivered by a conventional coronary angiography system. In this study with employing the 1B2 beamline where a monochromatic beam was used, approximately 3.4×1011 photons s-1 was delivered, and with employing the 7B2 beamline, the irradiation dose of a white beam was thought to be a thousands times more than that of a monochromatic beam. The white beam is strong enough to cause radiation damage on viable tissues and to vaporize intra-vascular blood in a relatively short period (Figs. 2 and 3). So the monochromatic beam is usually used for diagnostic procedures, and the white beam is used for radiotherapy.13) We exposed a rat heart to a continuous white beam for 15 minutes, and we could obtain the gross and pathologic images (Fig. 3). We found the gross color change of the heart along the course of the irradiation, and there was pathologically-determined loss of epicardial tissue and myocardial damage.
When air was used as a contrast agent, it was able to negate the limitations of iodine as a contrast agent. Air allowed the visualization of the internal parts of the vessel due to its positive contrasting, but even if there is overlapping of the vessels, the vessels that are closer or more distant do not impede the view. In addition, air allows sharp, clear images only of the structures that it passes through, and so it is possible to study vessels less than 10 µm and also to study specific areas of vessels. Figs. 1, 4 and 5 show the sharp delineation at the junction of the vessel wall and the air embolus. In addition to many other findings, we also observed the absorption of microscopic air emboli in a capillary, but these were dynamic images and they are not presented here.
Air as a contrast agent has many superior properties, yet the fact that it causes ischemia is a fatal limitation. Also, there is no way to confirm that the flow and distribution of air through the tissues is the same as that of blood in vivo. Many researchers are currently searching for a contrast agent that allows visualization of microvessels at the level of the capillary (for example, air containing nano-capsules), but which avoids the already-mentioned adverse effects on tissues; however, these attempts have not yet yielded any significant findings. A more suitable contrast agent or imaging method needs to be developed in order to study the microvessels of the beating heart in real time.
Figures and Tables
Fig. 1
The Langendorff apparatus built in a hutch of the Pohang Accelerator Laboratory. A rat heart was continuously perfused with oxygenated (95% O2/ 5% CO2) and warmed (38℃)
Krebs solution.
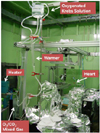
Fig. 2
Vascular torsion and vaporization of the intravascular blood, which were both due to synchrotron radiation heat. The contrast effect became more distinct as time went by.
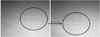
Fig. 3
Gross findings (A, B) and pathologic findings (C, D) of radiation tissue damage using the 7B2 beamline. A: when we exposed the heart to narrow-width radiation, a dark strip of tissue developed. B: we exposed the heart to broad width radiation twice for 15 minutes each time. Two bright strips were obtained. C: pathologic findings (×10) of the specimen and (B), loss of the epicardial tissue was found (large arrow). D: pathologic findings (×20) of the specimen and (B), myocardial damage was observed.
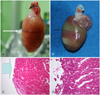
Fig. 4
Coronary angiography with iodine contrast agent in the heart of rat. The major coronary artery and its branch were visualized. However, it was difficult to get an image of the microvasculature.
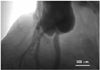
Acknowledgments
This study was supported by a scientific research grant of the Korean Society of Circulation (2006-5). The experiments at PLS were supported in part by MOST and POSTECH. We gratefully thank Jung Ho Je PhD. (Department of Materials Science and Engineering, Pohang University of Science and Technology) and Hwa-Shik Youn PhD. (Pohang Acceleratory Laboratory, Pohang University of Science and Technology) for their academic suggestions and technical support.
References
1. Hwu Y, Tsai WL, Groso A, Margaritondo G, Je JH. Coherence-enhanced synchrotron radiology: simple theory and practical applications. J Phys D Appl Phys. 2002. 35:R105–R120.
2. Morita M, Ohkawa M, Miyazaki S, Ishimaru T, Umetani K, Suzuki K. Simultaneous observation of superficial cortical and intracerebral microvessels in vivo during reperfusion after transient forebrain ischemia in rats using synchrotron radiation. Brain Res. 2007. 1158:116–122.
3. Kidoguchi K, Tamaki M, Mizobe T, et al. In vivo X-ray angiography in the mouse brain using synchrotron radiation. Stroke. 2006. 37:1856–1861.
4. Takeshita S, Isshiki T, Ochiai M, et al. Endothelium-dependent relaxation of collateral microvessels after intramuscular gene transfer of vascular endothelial growth factor in a rat model of hindlimb ischemia. Circulation. 1998. 98:1261–1263.
5. Takeda T, Momose A, Wu J, et al. Vessel imaging by interferometric phase-contrast X-ray technique. Circulation. 2002. 105:1708–1712.
6. Hwu Y, Tsai WL, Je JH, et al. Synchrotron microangiography with no contrast agent. Phys Med Biol. 2004. 49:501–508.
7. Kim JW, Seo HS, Hwu Y, et al. In vivo real-time vessel imaging and ex vivo 3D reconstruction of atherosclerotic plaque in apolipoprotein E-knockout mice using synchrotron radiation microscopy. Int J Cardiol. 2007. 114:166–171.
8. Baik S, Kim HS, Jeong MH, et al. International consortium on phase contrast imaging and radiology beamline at the Pohang Light Source. Rev Sci Instrum. 2004. 75:4355–4358.
9. Mori H, Hyodo K, Tobita K, et al. Visualization of penetrating transmural arteries in situ by monochromatic synchrotron radiation. Circulation. 1994. 89:863–871.
10. Yamashita T, Kawashima S, Ozaki M, et al. Mouse coronary angiograph using synchrotron radiation microangiography. Circulation. 2002. 105:E3–E4.
11. Takeshita S, Isshiki T, Mori H, et al. Use of synchrotron radiation microangiography to assess development of small collateral arteries in a rat model of hindlimb ischemia. Circulation. 1997. 95:805–808.
12. Ohtsuka S, Sugishita Y, Takeda T, et al. Dynamic intravenous coronary angiography using 2D monochromatic synchrotron radiation. Br J Radiol. 1999. 72:24–28.
13. Blattmann H, Gebbers JO, Bräuer-Krisch E, et al. Applications of synchrotron X-rays to radiotherapy. Nucl Instrum Methods Phys Res A. 2005. 548:17–22.