Abstract
Background and Objectives
Reperfusion of ischemic myocardium is necessary to salvage tissue from eventual death. However, new pathophysiological changes are initiated after reperfusion. The aim of this study was to investigate one of the mechanisms of ischemia/reperfusion (I/R) injury, and we focused on transferrin.
Metrials and Methods
Male Spragre-Dawley (SD) rats were used for the I/R model. Myocardial ischemia was produced by occlusion of the left anterior descending coronary artery for 30 minutes. 99mTc Transferrin-Chitosan-hydrazino nicotinate hydrochloride (HYNIC) (Tfc) (≒37 MBq/mL) was injected once after the reperfusion was finished. Autoradiography, hematoxylin and eosin (H & E) staining and determination of the tissue myeloperoxidase (MPO) activity were performed.
Results
Autoradiography showed remarkable 99mTc-Tfc uptake in the left ventricular myocardium at the reperfusion period from 0 to 1.5 hours, whereas no uptake was demonstrated at 3 hours. The uptake was increased again at 6 and 24 hours. Western blotting showed that the transferrin receptor (TfR) proteins were increased at 0 to 1.5 hours compared with that of the control; this expression of TfR disappeared at 3 hours, and it showed up for the second time at 6 and 24 hours. The MPO activity only at 24 hours was significantly higher than that of the control and those MPO activities at 0 to 6 hours (p=0.001).
Conclusion
In the rodent model of 30 minutes occlusion and reperfusion, our study revealed, with using 99mTc-Tfc, that the TfR expression increased in the myocardium till 3 hours after reperfusion. TfR-mediated entry of iron into the cardiomyocytes may represent that this process plays a role in the I/R injury during the early reperfusion period.
Tissue injury occurs during reperfusion after ischemic damage, although a reperfusion procedure is necessary to salvage tissue from eventual cell death. This injury is the so-called ischemia/reperfusion (I/R) injury. I/R injury has been reported in the heart, kidney, liver, lung and intestine, and it is known to be mediated by reactive oxygen-derived species (ROS) and by the pools of redox active iron and copper.1-3) The role of 'free' iron and copper on oxidative stress is mainly thought to cause the conversion of low reactive species, such as ·O2-, to the highly reactive ·OH radicals.4)
The morbidity from reperfusion remains high, and this is despite the development of imaging tools, interventional techniques and new drugs. Intensive research has been focused on the various pathophysiological mechanisms related to I/R injury and on the development of potential therapeutic strategies. Some of this research has been concerned with iron and transferrin for the mechanism of I/R injury. For example, Tacchini et al.5) reported that the transferrin receptor (TfR) gene expression was increased in the post-ischemic reperfusion model of rat liver and the enhanced iron uptake into liver cells might play a role in reperfusion damage. Omori et al.6) demonstrated that the expression of TfR on the brain endothelium is increased and this is gradually decreased in a transient reperfusion rat model. However, there have been no reports on the I/R injury-related TfR expression in the myocardium.
We have developed transferrin conjugated polyethylenimine (PEI) for targeting TfRs on tumor cells, and we have also recently developed transferrin conjugated chitosan to image the TfRs on inflammatory cells in vivo.7)8) Using this transferrin conjugate imaging agent, we tried to image the extent of the TfR expression in the I/R-injured myocardium and we investigated the mechanism of this I/R injury.
This study was performed in a fashion similar to that previously described for Transferrin-chitosan conjugates.8) Briefly, water-soluble chitosan (Mw=5 kDa, Kittolife Co., Seoul, Korea) was conjugated with succinimidyl 6-HYNIC. Human apo-transferrin (Sigma-Aldrich, St. Louis, MD) in sodium acetate buffer (30 mM, pH 5.0) was subjected to gel filtration on a PD-10 desalting column (Amersham Biosciences, Upsalla, Sweden). Sodium periodate, sodium cyanoborohydride and sodium chloride were then added to the chitosan-HYNIC solution. This was followed by dialysis against HBS (150 mM sodium chloride, 20 mM Hepes, pH 7.3) at 4℃. The iron incorporation was performed by the addition of 1.25 µL 10 mM iron (III) citrate buffer per mg of transferrin content. The conjugates were divided into convenient small aliquots and then they were kept at -20℃.
99mTc sodium pertechnetate (48 MBq) in 0.9% normal saline was mixed in a vial with 5 µg of tricine, 12 µg of SnCl2 (in 0.02 N HCl) and 500 µL of Tfc (Transferrin concentration: 3 mg/mL). The vial was then incubated at room temperature for 30 minutes. The labeling yield was determined by ITLC-SG (Gelman Sciences, Ann Arbor, MI) with using acetone and saline as the mobile phase.
These experiments were performed in accordance with the guidelines for the care and use of laboratory animals of Chonbuk National University. Male Sprague Dawley rats (7-8 weeks old, 250 to 300 g each, Samtako, Osan, Korea) were anesthetized with isoflurane before surgery. Myocardial ischemia was produced by occlusion of the left anterior descending coronary artery (LAD) for 30 minutes, and this was followed by reperfusion for 0, 30, 90 minutes, 3, 6 or 24 hours, respectively. For the 24 hours reperfusion experiment, the chest was closed in layers and the animal was weaned off the respirator, and the endotracheal tube was removed when the animal recovered spontaneous breathing and began to move. The sham operation was done with the same method as above except there was no occlusion of the LAD. Once the reperfusion was finished, 99mTc-Tfc (≒37 MBq/mL) was injected through the left external jugular vein. After 30 minutes, the hearts were harvested. The excised hearts were washed with saline and frozen-sectioned for autoradiography (BAS-1000, Fujifilm). For immunohistochemical determination of TfR or for measuring the tissue myeloperoxidase (MPO) activity, the hearts were collected immediately after the reperfusion was finished and they were maintained at -70℃.
To confirm the infiltration of neutrophils, histological evaluation with hematoxylin and eosin (H & E) staining was performed on the dissected myocardia. The frozen sections were washed with tap water for 5 minutes, immersed in hematoxylin for 2 minutes and then they were checked for complete staining in tap water. Eosin staining was carried out for 3 minutes. The sections were dehydrated through a graded series of alcohol solutions (70% to 100% ethanol, 3 minutes in each solution); they were cleared in xylene, cover-slipped and observed with a light microscope. The experiments were done in triplicate at each time point.
Immediately after the reperfusion experiment, 3 rats were sacrificed at each time point. The extracted, mounted hearts were sectioned at 6 µm thickness using a cryomicrotome (Leica, Wetzlar, Germany). The sections exposed to an image plate for 2-5 minutes and this image plate was scanned with an image reader. Autoradiography was performed using a Fuji BAS1000 analyzer.
The total-cell extracts were prepared by standard techniques. The tissues were washed twice in cold phosphate buffered saline (PBS) (pH 7.4) and then they were lysed for 30 minutes at 4℃ with a buffer that contained 20 mM Tris-HCl (pH 7.4), 2 mM ethylenediaminetetraacetic acid (EDTA), 1 mM sodium orthovanadate, 0.1 mM phenylmethanesulphonyl fluoride (PMSF) and a cocktail of protease inhibitors. The lysates were centrifuged at 15,000 g for 10 minutes at 4℃ and the supernatants were separated into aliquots and stored at -80℃. Aliquots of the membrane fraction (approx. 30 µg) were run on a 10% sodium dodecyl sulfate-polyacrylamide gel electrophoresis (SDS PAGE) under denaturing and reducing conditions, and the proteins were trans-blotted onto nitrocellulose (NC) membranes. Non-specific binding of the antibody to the membrane was blocked by a 1 hours treatment with 5% non-fat dried milk.
The membrane was subsequently incubated overnight with the primary rabbit polyclonal immunoglobulin G (IgG) antibody (CD71) and then it was incubated for 1 hours with goat anti-rabbit IgG horseradish peroxidase (HRP) conjugated secondary antibody. The immunoreactivity was detected with using an enhanced chemiluminescence detection kit (Pierce, Rockford, IL). The experiments for each time point after the sham operation or reperfusion were duplicated.
Neutrophil accumulation in the rat myocardium was investigated by measuring the myeloperoxidase, as was described previously.9) Briefly, the heart was washed twice in ice-cold potassium phosphate buffer (PPB) solution (20 mM, pH 7.4) and then it was lysed with 2 mL ice-cold PPB and by using a homogenizer. The lysates were centrifuged at 12,000 rpm for 20 minutes at 4℃ and the supernatants were discarded. This process was repeated twice. The pellet was suspended in membrane lysis buffer (0.5% hexacyltrimethylamonium bromide, 10 mM EDTA in 50 mM, pH 6.0, PPB). Then the thawing and freezing steps were repeated 3 or 4 times. The lysates were next centrifuged at 12,000 rpm for 20 minutes at 4℃ and then the supernatants were separated into a new micro-centrifuge tube. This fraction was added to reaction buffer (1 mL PPB solution, 16.7 µL o-Dianisidine dihydrochloride, 17 µL 0.3% H2O2). After the reaction, the optical density was detected at 450 nm with using a UV spectrometer.
The Tfc was synthesized by carbohydrate conjugation. The polysaccharides on the transferrin were oxidated with sodium periodate. The oxidation reaction creates highly reactive aldehyde functionalities. The amines on the chitosan can react with aldehyde groups under reductive amination conditions with using sodium cyanoborohydride. The conjugates were characterized by Matrix-Assisted Laser Desorption Ionization Time of Flight (MALDI-TOF) mass spectrometry.8) The spectrum results showed the increased molecular weight after conjugation, which demonstrated that human apotransferrin was successfully conjugated as Tfc. We have already reported that this conjugate targets the TfR on cell membranes.8)
On the instant thin-layer chromatography (ITLC-SG) radio-chromatography, the in vitro labeling efficiency of the 99mTc-Tfc was higher than 95% for up to 6 hours. The 99mTc-Tfc was incubated at 37℃ with 1 mL of human serum. The human serum stability was above 98% and 94% in acetone and saline, respectively, during 6 hours. The prepared conjugate was stable for at least 6 hours when stored at room temperature, and it was also stable for 6 hours in serum at 37℃.
The histopathology showed myocardial damage and the neutrophils infiltrated from the epicardia to the myocardia with the increased reperfusion time. The degree of neutrophil infiltration showed a sparse, focal pattern until 3 hours after reperfusion, and thereafter this changed to a transmural, diffuse pattern from 6 hours to 24 hours (Fig. 1).
To demonstrate that the uptake of 99mTc-Tfc in the heart corresponded to I/R injury, the rat hearts were subjected to digital autoradiography following the I/R experiment. The uptake of 99mTc-Tfc in the I/R injured myocardium until 90 minutes after reperfusion was gradually intense. The models reperfused during 3 hours showed little uptake of 99mTc-Tfc in the sectioned heart. Thereafter, a diffuse uptake of 99mTc-Tfc appeared in all the myocardial layers (Fig. 2).
We determined the amount of TfR in the IR myocardial models. The results of western blotting showed that TfR proteins were increased at 0 to 1.5 hours compared with that of the sham operation; the TfR proteins disappeared at 3 hours, and they showed up again at 6 to 24 hours for a second time.
The MPO activity only at 24 hours was significantly higher than that of the sham operation and those MPO activities at 0 to 6 hours (p=0.001).
The present study demonstrated the potential of employing 99mTc-Tfc for imaging the increased expression of TfR receptor after I/R injury in the myocardium. We recently reported on the synthesis and in vivo evaluation of the same imaging agent for detecting infection/inflammation lesions in Balb/C mice.8) In the case of the inflammatory process, the site where TfRs are expressed is predominantly inflamed lesion that is infiltrated by inflammatory cells such as macrophages and leukocytes. In this present study, macrophages or leukocytes did not predominantly infiltrate into the myocardium until the 3 hours after the myocardium was damaged by reperfusion injury. Nevertheless, the results of western blotting and autoradiographic imaging using 99mTc-Tfc showed the overexpression of TfR in the injured myocardia and also intensive binding of 99mTc-Tfc to its receptors. It means that the over-expression of TfR was induced in the damaged area at the early period of I/R injury.
Previous studies have demonstrated that I/R injury is related to iron intake through receptor-mediated endocytosis in the various organs of animal models. The results of this study support that for the 30 minutes occlusion model, the over-expression of TfR on the myocardium during approximately the first 3 hours is related with the free iron influx into the myocardial cells by endocytosis, and I/R injury develops thereafter. Additionally, there were some reports on apoptosis being related with myocardial reperfusion injury.10)11) Further study is needed to investigate the relationship between apoptosis and iron uptake into the damaged myocardial cells. Although the most effective therapeutic strategy is restoration of blood flow to the ischemic myocardia, knowing that reperfusion itself is accompanied with injury by the above mentioned mechanisms is important to establish an effective method to minimize the damage. The time course, distribution and severity of I/R injury may be different among the patients with myocardial infarction. Imaging studies with using targeting molecules, like TfR or apoptotic-related cellular proteins, are powerful tools for determining such factors as iron intake, neutrophils infiltration and apoptosis in patients suffering with myocardial ischemia or infarction.10)
Molecular imaging techniques such as autoradiography are useful tools for investigating the pathophysiologic mechanisms of various diseases. In this study, we used a synthetic transferrin conjugate that was capable of being labeled with radioisotope (99mTc) and it was capable of binding with its receptors. TfRs are known to be on many kinds of tumor cells, and activated macrophages and reperfused cells induce their overexpression according to different stimuli, as was seen in this study. Therefore, this conjugate can provide information on the degree of expression of its receptors when an adequate imaging modality is selected, like autoradiography, which was used in our study. For clinical or preclinical situations, nuclear imaging with 99mTc and using gamma camera has been widely used. In vivo nuclear imaging for 99mTc-Tfc, and not ex vivo autoradiographic imaging, could show the over-expression of TfR in the I/R injured myocardium if we used an animal imaging system, like micro single photon emission computerized tomography (SPECT). The results of myeloperoxidase assay distinguished between the cells that expressed TfR with time, that is, the myocardial cells and leukocytes. Both the free iron influx by the TfR and the infiltration of inflammatory cells would have an effect on the pathogenesis of reperfused myocardial injury according to the time course.12) In this study, the MPO activity at 24 hours after reperfusion was significantly increased. This finding is well correlated with that of the H & E staining. After performing reperfusion, neutrophils infiltrates after 6 hours, and particularly on the first day, much more than before. These neutrophils aggravate the I/R injury. Understanding the mechanism of I/R injury and the in vivo status according to the time course will provide important information for selecting the appropriate therapeutic regimens and modalities.13)14)
In conclusion, when we studied the status of TfR in the 30 minutes occlusion rat model with using the novel synthetic 99mTc-Tfc, an increased expression of its receptor in the myocardium was shown, and inflammatory cell infiltrates did not predominantly participate in the myocardial damage till 3 hours after reperfusion. TfR-mediated entry of iron into the cardiomyocytes may represent that iron plays a role in I/R injury during the early reperfusion period. A greater understanding of the TfR-mediated pathophysiologic process in the I/R model will provide a key for developing new therapeutic strategies that can reduce I/R injury in those patients who suffer with coronary artery disease.
Figures and Tables
Fig. 1
H & E staining according to the duration of reperfusion (×100). The neutrophil infiltration in the model of myocardial ischemia/reperfusion (I/R) became severe and then it became diffuse after the reperfused time went on for 24 hours. H & E: hematoxylin and eosin.
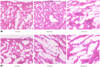
Fig. 2
Representative autoradiographic findings after injecting 37 MBq 99mTc transferrin-chitosan-HYNIC (99mTc-Tfc) via tail vein. The Tfc images demonstrate the area and intensity of binding to TfR. The 99mTc-Tfc uptake was increased with time after reperfusion until 3 hours and then it decreased at 3 hours. Thereafter, the uptake showed a diffuse pattern in the injured myocardial layer. Upper row: the auto-radiographic images, lower row: the sliced myocardial tissue for autoradiography. RT: reperfusion time, HYNIC: hydrazino nicotinate hydrochloride.
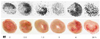
Fig. 3
Representative results of western blotting for transferrin receptor (TfR) and the myeloperoxidase (MPO) assay according to the duration of reperfusion time after occlusion. The amount of TfR proteins was increased at 0, 0.5 and 1.5 hours compared with that of the sham operation. The expression of TfR was changed from very low at 3 hours and it showed up again at 6 and 24 hours for a second time. The MPO activity only at 24 hours was significantly increased. *p<0.05. RT: reperfusion time.
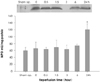
References
1. Jaeschke H. Reactive oxygen and ischemia/reperfusion injury of the liver. Chem Biol Interact. 1991. 79:115–136.
2. Kirschner RE, Fantini GA. Role of iron and oxygen-derived free radicals in ischemia-reperfusion injury. J Am Coll Surg. 1994. 179:103–117.
3. Lentsch AB, Kato A, Yoshidome H, McMasters KM, Edwards MJ. Inflammatory mechanisms and therapeutic strategies for warm hepatic ischemia/reperfusion injury. Hepatology. 2000. 32:169–173.
4. McCord JM. Effect of positive iron status at a cellular level. Nutr Rev. 1996. 54:85–88.
5. Tacchini L, Fusar Poli D, Bernelli-Zazzera A, Cairo G. Transferrin receptor gene expression and transferrin-bound iron uptake are increased during postischemic rat liver reperfusion. Hepatology. 2002. 36:103–111.
6. Omori N, Maruyama K, Jin G, et al. Targeting of post-ischemic cerebral endothelium in rat by liposomes bearing polyethylene glycol-coupled transferrin. Neurol Res. 2003. 25:275–279.
7. Kim EM, Jeong HJ, Heo YJ, Moon HB, Bom HS, Kim CG. Intratumoral injection of 188Re labeled cationic polyethyleneimine conjugate: a preliminary report. J Korean Med Sci. 2004. 19:647–651.
8. Kim EM, Jeong HJ, Kim SL, et al. Synthesis and in vivo evaluation of 99mTc-transferrin conjugate for detection of inflamed site. J Drug Target. 2007. 15:595–602.
9. Lysiak JJ, Turner SD, Nguyen QA, Singbartl K, Ley K, Turner TT. Essential role of neutrophils in germ cell-specific apoptosis following ischemia/reperfusion injury of the mouse testis. Biol Reprod. 2001. 65:718–725.
10. Taki J, Higuchi T, Kawashima A, et al. Detection of cardiomyocyte death in a rat model of ischemia and reperfusion using 99mTc-labeled annexin V. J Nucl Med. 2004. 45:1536–1541.
11. Kim YK. Apoptosis in ischemia-reperfused myocardium of rabbit. Korean Circ J. 1997. 27:1017–1026.
12. Vinten-Johansen J. Involvement of neutrophils in the pathogenesis of lethal myocardial reperfusion injury. Cardiovasc Res. 2004. 61:481–497.
13. de Vries B, Walter SJ, von Bonsdorff L, et al. Reduction of circulating redox-active iron by apotransferrin protects against renal ischemia-reperfusion injury. Transplantation. 2004. 77:669–675.
14. Becker LB. New concepts in reactive oxygen species and cardiovascular reperfusion physiology. Cardiovasc Res. 2004. 61:461–470.