Abstract
Background and Objectives
Pulmonary hypertension is characterized by abnormal proliferation of vascular endothelial cells and smooth muscle cells, and progressive pulmonary microvascular leakage that leads to pulmonary edema. This study was designed to investigate the protective effect of simvastatin on monocrotaline (MCT)- induced pulmonary hypertension and the role of the aquaporin (AQP) water channels.
Materials and Methods
Twenty one 8-week-old rats were randomized to the control, MCT (60 mg/kg, sc) and the MCT plus simvastatin (5 mg/kg/day, po) groups. Four weeks later, the systolic right ventricular pressure, the right ventricular hypertrophy, the medial wall thickness of the peribronchiolar artery and pulmonary arterioles and the renal function were measured to examine the effects of MCT and simvastatin in the rats. Western blotting for lung aquaporin1 (AQP1) and renal aquaporin2 (AQP2) was performed to analyze the effects of MCT and simvastatin on the AQP water channels.
Results
Treatment with simvastatin reduced the MCT-induced enhanced right ventricular pressure (32.3±2.1 vs. 52.4±3.9 mmHg, respectively; p<0.05), the right ventricular hypertrophy (0.32±0.03 vs. 0.48±0.07, respectively; p<0.05) and the increased medial wall thickness of the peribronchiolar artery (0.14±0.02 vs. 0.28±0.02, respectively; p<0.05) and pulmonary arterioles (0.15±0.04 vs. 0.29±0.11, respectively; p<0.05). The decreased expression of lung AQP1 and renal AQP2 protein after MCT treatment was normalized by simvastatin treatment (p<0.05). Additionally, simvastatin treatment significantly reduced the perivascular and interstitial edema in the rats' lungs without major alterations of renal function.
Pulmonary arterial hypertension (PAH) is a fatal disease that's caused by progressive narrowing of the pulmonary arterioles and increased pulmonary vascular resistance, and this can result in right ventricular failure and death.1)2) There are several factors that play roles in the pathogenesis of PAH such as genetic mutation of bone morphogenetic protein receptor II (BMPR II) and activin receptor-like kinase 1 (ALK-1), exposure to drugs, infection and inflammation. These factors eventually lead to multiple abnormalities, including endothelial cell proliferation, matrix production, thrombosis, abnormal vascular vasoconstriction and vascular remodeling.2)
The currently available treatments for PAH include adjunctive therapy like diuretics, anticoagulant, digoxin and oxygen for right heart failure, and pulmonary vasodilating agents such as calcium-channel blockers, prostanoids and endothelin receptor antagonists.3) However, these currently available therapies can not reverse the disease process or the considerable morbidity and mortality, although they have a beneficial clinical effect.4) Therefore, new therapies are warranted that are based on a better understanding of the pathophysiology of the disease, and especially those therapies that are directed at suppressing inappropriate cellular proliferation in the pulmonary arteries.2)5)
Simvastatin is a well known drug with cardiovascular benefits that exceed their effects on lowering serum cholesterol. In addition, simvastatin also exert potent antiproliferative and proapoptotic effects on vascular smooth muscle cells through the inhibiting the activities of ras and rho guanine triphosphatase (GTPase), which are important for cell proliferation.6) It has recently been demonstrated that simvastatin could reverse established, severe pulmonary hypertension after toxic injury in rats and improve their survival. Other studies also showed that simvastatin treatment potently attenuates chronic hypoxic pulmonary hypertension in rats and it inhibits vascular remodeling.2)7-10)
The aquaporins (AQPs) are a family of small membrane-spanning proteins that are expressed on the plasma membranes of many cell types and they facilitate water transport. In the lung, AQP1 is expressed on the apical and basolateral membranes of the microvascular endothelial cells. The abundance of AQP1 in the peribronchiolar vessels of perinatal rats may suggest their role in clearance of lung water from the alveolar space.11) Renal AQP2 is present in the vesicles of the collecting duct principal cells that translocate to the apical membrane in response to vasopressin.12) AQP2 overexpression has been described in several conditions associated with fluid retention, including congestive heart failure.13) These results suggest that AQP may play a role in the edema formation in PAH. Several animal models of PAH revealed pulmonary microvascular leakage and interstitial inflammation, which resulted in pulmonary edema.14) The aims of this study were examine the protective effect of simvastatin on monocrotaline (MCT)-induced PAH and the role of the AQP water channels.
Twenty one male Sprague Dawley rats (180-200 g) were randomly divided into three groups: group 1 was injected with distillated water (the control group, n=7), group 2 was treated with MCT (the MCT group: MCT was injected at a single dose 60 mg/kg, sc, n=7) and group 3 was MCT rats that were treated with simvastatin (Hanmi Pharmacy, Seoul, Korea) (the MCT+S group, 5 mg/kg/day, po, daily for 28 days from the onset of MCT treatment, n=7). The rats in all three groups were kept in the same room and all of them were subjected to the same light-dark cycle. After 28 days, their tissue samples were obtained for morphometric analysis and western blotting. The experimental procedures we used were reviewed and approved by the Animal Care and Use Committee of Dongguk University. The animal care and use were in accordance with the guidelines of the National Institute of Health.
After 28 days, the animals were anaesthetized by an intraperitonial injection of ketamine (100 mg/kg). For measuring the right ventricular pressure, a PE 50 catheter (Becton Dickinson, Franklin Lakes, NJ, USA) was inserted into the right ventricle via the internal jugular vein, and a fluid-filled PE-50 tube was connected to a pressure transducer (Grass polygraph, Grass instrument CO, Quincy, MA, USA).
After 4 weeks, the rats were euthanized by an anesthetic overdose and then the right ventricle (RV) free wall was dissected from the left ventricle (LV) and septum (S), and they were weighed separately on the analytic scale. The RV remodeling was assessed by the RV-to-LV plus S weight ratio. For analyzing the vascular remodeling, the left lung was fixed with a transcardiac infusion of 4% paraformaldehyde. The perfused lung was removed and then paraffin-embedded. Serial coronal sections 5 µm thick were obtained at the lower zone of the lung. Following deparaffinization, the sections were stained with the hematoxylin-eosin (H&E). The medial wall thickness (MWT) of the pulmonary arterioles was measured at the pulmonary arterioles that were 50-100 µm in size and at the peribronchiolar muscular arteries. The MWT ratio, which is an index of medial wall hypertrophy, was determined as the average data of 10 to 15 fields per slice and the MWT ratio was calculated as: [MWT=(external diameter-internal diameter)/external diameter].
The MCT and/or simvastatin treated lung and kidney tissues were removed and then snap-frozen at -70℃ for Western Blot analysis. The tissue samples were homogenized in ten volumes of homogenizing buffer (0.32 M sucrose, 25 mM imidazole and 1 mM ethylenediaminetetraacetic acid (EDTA) (pH 7.2) containing 8.5 mM leupeptin and 1 mM phenylmethylsulfonyl fluoride), for 10 s with using a polytron. The aliquots were stored at -70℃. Samples of the homogenate were run on 7.5% polyacrylamide mini gels (Bio-rad Mini Protean). For each gel, an identical gel was run in parallel and the two gels subjected to Coomassie staining to assure identical loading. After electrophoresis, the protein was transferred to nitrocellulose paper for 2 hours at 400 mA and 120 V in a BioRad transblot system. After transfer, the protein bands were identified by Ponceaus S and they were destained with distilled water. The nitrocelluse sheets were washed in tween phosphate buffered saline (PBST) and then incubated with rabbit anti-AQP1 (Alomone, Jerusalem, Israel) and rabbit anti-AQP2 (Alomone, Jerusalem, Israel) for overnight at 4℃. The labeling was visualized with horseradish peroxidase-conjugated secondary antibodies (Santa Cruz Biotechnology, Santa Cruz, CA, USA) and with using an enhanced chemiluminescence (ECL) system (Amersham Pharmacia Biotech, Little Chalfont, UK). The immunoblot signal was developed by an ECL system and it was quantified using Scion Image software (version 1.59).
On day 26 after treatments, the animals were individually housed in metabolic cages. The daily dietary intake and urine volume were measured for 2 days. The rats' blood and urine samples were stored for electrolyte and renal function testing.
All the data is presented as means±SDs. The data was analyzed by one-way analysis of variance (ANOVA) followed by Tukey's multiple-comparisons test. Multiple-comparisons tests were applied only when a significant difference was determined by ANOVA (p<0.05). P<0.05 were considered statistically significant.
The right ventricular pressure was significantly increased in the MCT group as compared with that of the controls (52.4±3.9 vs. 24.1±0.26 mmHg, respectively, p<0.05), and the right ventricular pressure was markedly suppressed in the simvastatin treatment group (32.3±2.1 mmHg, p<0.05). However, simvastatin treatment did not suppress the right ventricular pressure to normal values (p<0.05) (Fig. 1).
In the MCT group, right ventricular hypertrophy developed and there were significant increases in the RV/LV+S ratio compared with that of the controls (0.48±0.07 vs. 0.26±0.02, respectively, p<0.05), and the right ventricular pressure was markedly suppressed in the simvastatin treatment group (0.32±0.03, p<0.05). However, simvastatin treatment did not completely suppress the RV hypertrophy to normal values (p<0.05) (Fig. 2).
MCT treatment increased the medial wall thickness (MWT ratio) of the peribronchiolar artery compared with that of the controls (0.28±0.02 vs. 0.10±0.03, respectively, p<0.05), and the medial wall thickness was significantly suppressed in the simvastatin treated group (0.14±0.02, p<0.05) (Fig. 3A-C). The medial wall thickness of the small pulmonary arterioles (50-100 µm) was also significantly reduced in the simvastatin treated group as compared with the MCT group (0.15±0.04 vs. 0.29±0.11, respectively, p<0.05) (Fig. 3D-F). This treatment, however, did not completely reverse the pulmonary arterial wall thickness to a normal value (p<0.05). Perivascular and interstitial edema was clearly seen in the MCT group. However, simvastatin treatment significantly reduced the pulmonary edema (Fig. 3G-I).
To quantitatively evaluate the effect of simvastatin on MCT-induced pulmonary hypertension, the AQP1 and AQP2 expressions were measured in the lung and kidney from the controls and the MCT and/or simvastatin treated rats. Western blot analysis demonstrated that the expression of lung AQP1 and renal AQP2 in the MCT group was significantly decreased compared with that of the control group (p<0.05). However, the expression of lung AQP1 and kidney AQP2 was normalized after treatment with simvastatin (p<0.05) (Figs. 4 and 5).
The serum osmolarity and sodium levels were slightly increased in the MCT group (p<0.05), but they were normalized after simvastatin treatment (p<0.05). Other parameters of renal functions such as the urine output and the Na, K, blood urea nitrogen, creatinine and creatinine clearance were not altered (Table 1).
PAH is a disease that's characterized by elevated pulmonary artery pressure, and this can lead to right ventricular failure and death.1)2) The median survival of PAH patients was reported to be just a few years with an estimated 5-year survival of 34%.15-17) Yet the newer medical therapies, besides the adjunctive therapies, have been shown to improve a variety of clinically relevant end-points, including survival, exercise tolerance, the haemodynamics and the quality of life measures.2)5) The introduction of continuous intravenous prostacyclin, a dual endothelin receptor antagonist, for the treatment of PAH has been promising.3) Unfortunately, there is no curative therapy. Therefore, the current therapy for PAH is based on a better understanding of its pathogenesis and an effort to reverse the vascular remodeling with using phosphodiesterase type 5 inhibitors, elastase inhibitor, platelet derived growth factor (PDGF) receptor antagonist and simvastatin.12)18)19)
Simvastatin improves the cardiovascular outcomes, and this is independent of its effects on cholesterol reduction. In addition to its potent antipoliferative and antiapoptotic effects on the vascular smooth muscle cells,20) simvastatin enhances the production of endothelial nitric oxide synthase (NOS)21) and it also has anti-inflammatory effects.22) Simvastatin treatment causes activation of caspase-3 and pulmonary microvascular endothelial cell apoptosis in cases of severe pulmonary hypertension.10) It has recently been demonstrated that simvastatin could reverse or attenuate pulmonary hypertension.9)23) A small study reported improvements of the 6 min walk tests and the hemodynamics in patients who received simvastatin daily.6) Clinically, we showed that simvastatin decreased the right ventricular pressure, the right ventricular hypertrophy and the medial wall thickness of the pulmonary artery in the rats with MCT-induced PAH. These results suggest that simvastatin attenuates the progression of MCT-induced pulmonary hypertension through the reduction of vascular resistance. This study was not intended to explore the mechanisms of simvastatin's effects, but the increased apoptosis of vascular smooth muscle cells and/or the endothelial cells and production of endothelial NOS are might be candidate mechanisms that prevent the increase of vascular resistance.
The existence of water-specific membrane channel proteins in selected tissues has been postulated for several decades, and eleven mammalian AQPs have now been identified.12) The abundance of AQP1 in the peribronchiolar vessels may suggest a role for this protein in clearance of water from the interstitial space.11) In ventilator-induced lung injury, AQP water channels have protective effects on pulmonary edema formation.24) However, in a murine model of lipopolysaccharide-induced acute lung injury, depletion of AQP1 did not affect the formation of lung edema, the lung vascular permeability or the lung histology.25) These results suggest that any correlation between the decreased expression of AQP1 and lung edema formation is controversial. In this study, we revealed that the APQ1 expression was decreased in the MCT-induced PAH rat tissues and this was normalized with simvastatin treatment. The APQ1 expression was also correlated with the resolution of lung edema. Recent papers have reported that AQP depletion would have subclinical effects on water homeostasis, and these effects would become apparent under stressful conditions such as congestive heart failure and pulmonary edema.12)26) Therefore, we suggest that simvastatin might attenuate the formation of pulmonary edema through the decreased expression of AQP1 water channels.
In a previous rat model of congestive heart failure caused by acute myocardial infarction, the renal AQP2 expression was activated in the apical plasma membrane of the renal collecting ducts, which accounted for fluid overload.13) Further, the up-regulation of APQ2 was normalized after fosinopril, valsartan and losartan treatment.27)28) So, we hypothesized that the fluid retention with pulmonary edema in PAH would be related with alterations of the renal AQP2 expression. Our study revealed that the decreased expression of renal APQ2 in MCT-induced PAH rat tissues was not due to functional renal changes such as increased urine output or lowering the sodium concentration in the serum. Therefore, we speculate that the altered expression of renal AQP2 after MCT and/or simvastatin was not associated with the formation and resolution of pulmonary edema. The altered expression of AQP2 may be due to the compromised true glomerular filtration rate and it may be somewhat due to the dehydration via MCT-induced renal damage.
In summary, our study demonstrated that simvastatin attenuates the progression of MCT-induced pulmonary hypertension and the pulmonary edema by the increased expression of lung AQP1. Further investigations will be needed to reveal the mechanisms of simvastatin effects and the exact role of APQs in PAH.
Figures and Tables
Fig. 1
The systolic right ventricle pressure. Simvaststin (S) prevented the development of pulmonary arterial hypertension in the monocrotaline (MCT) treated rats. However, simvastatin treatment did not suppress the right ventricular pressure to normal values. The results are expressed as means±SDs. *p<0.05 vs. control, †p<0.05 vs. MCT.
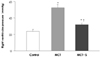
Fig. 2
The ratio of the right ventricle (RV) to left ventricle (LV) plus septum weight. The monocrotaline (MCT)-induced RV hypertrophy was attenuated after simvastatin (S) treatment. However, simvastatin treatment did not completely suppress the RV hypertrophy to normal values. Values are expressed as means±SDs. *p<0.05 vs. control, †p<0.05 vs. MCT.
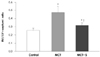
Fig. 3
Light microscopic findings of lung. Representative photographs of the peribronchiolar muscular pulmonary artery (A-C, ×200), the pulmonary arteriole (D-F, ×400), and the histological change of the lung (G-I, ×200). The increased medial wall thickness in the monocrotaline (MCT) treatment group was reduced with simvastatin (S) treatment. MCT induced severe perivascular and interstitial edema, but simvastatin treatment markedly reduced the pulmonary edema. Hematoxylin and eosin staining. Bar=50 µm. Br: bronchiole.
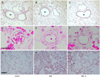
Fig. 4
AQP1 in lung. The expression of lung AQP1 was significantly diminished in the monocrotaline (MCT)-induced pulmonary hypertension rats. But the lung AQP1 protein expression was normalized by simvastatin (S) treatment. Values are expressed as means±SDs. *p<0.05 vs. control, †p<0.05 vs. MCT. AQP: aquaporin.
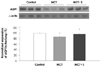
Fig. 5
AQP2 in kidney. The expression of renal AQP2 was significantly diminished in the monocrotaline (MCT)-induced pulmonary hypertension rats. But the renal AQP2 protein expression was normalized by simvastatin (S) treatment. Values are expressed as means±SDs. *p<0.05 vs. control, †p<0.05 vs. MCT. AQP: aquaporin.
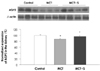
Acknowledgments
This work was supported in part by grants from the Dongguk University Research Fund.
References
1. Humbert M, Morrell NW, Archer SL, et al. Cellular and molecular pathobiology of pulmonary arterial hypertension. J Am Coll Cardiol. 2004. 43(Suppl):13S–24S.
2. Martin KB, Klinger JR, Rounds SI. Pulmonary arterial hypertension: new insights and new hope. Respirology. 2006. 11:6–17.
3. Lee SH, Rubin LJ. Current treatment strategies for pulmonary arterial hypertension. J Intern Med. 2005. 258:199–215.
4. Kunieda T, Nakanishi N, Satoh T, Kyotani S, Okano Y, Nagaya N. Prognoses of primary pulmonary hypertension and chronic major vessel thromboembolic pulmonary hypertension determined from cumulative survival curves. Intern Med. 1999. 38:543–546.
5. Puri A, McGoon MD, Kushwaha SS. Pulmonary arterial hypertension: current therapeutic strategies. Nat Clin Pract Cardiovasc Med. 2007. 4:319–329.
6. Kao PN. Simvastatin treatment of pulmonary hypertension: an observational case series. Chest. 2005. 127:1446–1452.
7. Hu H, Sung A, Zhao G, et al. Simvastatin enhances bone morphogenetic protein receptor type II expression. Biochem Biophys Res Commun. 2006. 339:59–64.
8. Girgis RE, Li D, Zhan X, et al. Attenuation of chronic hypoxic pulmonary hypertension by simvastatin. Am J Physiol Heart Circ Physiol. 2003. 285:H938–H945.
9. Nishimura T, Vaszar LT, Faul JL, et al. Simvastatin rescues rats from fatal pulmonary hypertension by inducing apoptosis of neointimal smooth muscle cells. Circulation. 2003. 108:1640–1645.
10. Taraseviciene-Stewart L, Scerbavicius R, Choe KH, et al. Simvastatin causes endothelial cell apoptosis and attenuates severe pulmonary hypertension. Am J Physiol Lung Cell Mol Physiol. 2006. 291:L668–L676.
11. King LS, Nielsen S, Agre P. Aquaporin-1 water channel protein in lung: ontogeny, steroid-induced expression, and distribution in rat. J Clin Invest. 1996. 97:2183–2191.
12. King LS, Yasui M. Aquaporins and disease: lessons from mice to humans. Trends Endocrinol Metab. 2002. 13:355–360.
13. Nielsen S, Terris J, Andersen D, et al. Congestive heart failure in rats is associated with increased expression and targeting of aquaporin-2 water channel in collecting duct. Proc Natl Acad Sci U S A. 1997. 94:5450–5455.
14. Reindel JF, Ganey PE, Wagner JG, Slocombe RF, Roth RA. Development of morphologic, hemodynamic, and biochemical changes in lungs of rats given monocrotaline pyrrole. Toxicol Appl Pharmacol. 1990. 106:179–200.
15. D'Alonzo GE, Barst RJ, Ayres SM, et al. Survival in patients with primary pulmonary hypertension: results from a national prospective registry. Ann Intern Med. 1991. 115:343–349.
16. Lee WD, Kim DS, Lee JH, et al. A clinical review of primary pulmonary hypertension. Korean Circ J. 2003. 33:507–512.
17. Lee WS, Kim KH, Jeong DH, et al. Clinical characteristics and prognostic factors of patients with severe pulmonary hypertension. Korean Circ J. 2007. 37:265–270.
18. Schermuly RT, Kreisselmeier KP, Ghofrani HA, et al. Chronic sildenafil treatment inhibits monocrotaline-induced pulmonary hypertension in rats. Am J Respir Crit Care Med. 2004. 169:39–45.
19. Newman JH, Fanburg BL, Archer SL, et al. Pulmonary arterial hypertension: future directions: report of a National Heart, Lung and Blood Institute/Office of Rare Diseases workshop. Circulation. 2004. 109:2947–2952.
20. Takemoto M, Liao JK. Pleiotropic effects of 3-hydroxy-3-methylglutaryl coenzyme a reductase inhibitors. Arterioscler Thromb Vasc Biol. 2001. 21:1712–1719.
21. Laufs U, Fata VL, Liao JK. Inhibition of 3-hydroxy-3-methylglutaryl (HMG)-CoA reductase blocks hypoxia-mediated down-regulation of endothelial nitric oxide synthase. J Biol Chem. 1997. 272:31725–31729.
22. Son JW, Koh KK, You SM, et al. Effects of simvastatin alone or combined with ramipril on nitric oxide bioactivity and inflammation markers in hypercholesterolemic patients. Korean Circ J. 2003. 33:1053–1059.
23. Girgis RE, Mozammel S, Champion HC, et al. Regression of chronic hypoxic pulmonary hypertension by simvastatin. Am J Physiol Lung Cell Mol Physiol. 2007. 292:L1105–L1110.
24. Hales CA, Du HK, Volokhov A, Mourfarrej R, Quinn DA. Aquaporin channels may modulate ventilator-induced lung injury. Respir Physiol. 2001. 124:159–166.
25. Su X, Song Y, Jiang J, Bai C. The role of aquaporin-1 (AQP1) expression in a murine model of lipopolysaccharide-induced acute lung injury. Respir Physiol Neurobiol. 2004. 142:1–11.
26. Agre P, King LS, Yasui M, et al. Aquaporin water channels-from atomic structure to clinical medicine. J Physiol. 2002. 542:3–16.
27. Staahltoft D, Nielsen S, Janjua NR, et al. Losartan treatment normalizes renal sodium and water handling in rats with mild congestive heart failure. Am J Physiol Renal Physiol. 2002. 282:F307–F315.
28. Yu CM, Wing-Hon Lai K, Li PS, Lam KY, Leung JC, Lai KN. Normalization of renal aquaporin-2 water channel expression by fosinopril, valsartan, and combination therapy in congestive heart failure: a new mechanism of action. J Mol Cell Cardiol. 2004. 36:445–453.