Abstract
Background and Objectives
Resveratrol (trans-3, 4', 5-trihydroxy-stilbene), a naturally occurring polyphenolic phytoalexin found abundantly in grape skins and red wines, has been reported to protect heart cells from ischemia/reperfusion (I/R) injury through its significant antioxidant properties. Apoptosis of cardiac myocytes is also involved in several cardiovascular diseases, but it remains unknown whether the protective effects of resveratrol in hypoxic myocardial cell injury are mediated via suppression of apoptosis. In this study, we investigated whether resveratrol confers cardioprotection against hypoxia via anti-apoptosis in a hypoxic model of cultured H9c2 cardiomyoblasts.
Materials and Methods
H9c2 cardiomyoblasts were obtained from the Korean Cell Line Bank. The cultured cells were divided into four groups: a normal control group, a hypoxia group, a group treated with resveratrol (10 µg/mL) before hypoxic insult, and a group treated with resveratrol (10 µg/mL) after hypoxic insult. The control group was placed in 5% CO2 incubators, and the hypoxia and resveratrol-treated groups were placed in 1% O2 incubators. Apoptosis was assayed by cytological analysis with Western blotting and real-time PCR for Bcl-2, Bax, and caspase-3.
Results
The expression of Bcl-2 was significantly decreased in the hypoxia group compared with the control group, and resveratrol treatment inhibited the hypoxia-induced decline of Bcl-2 in hypoxic myocardial cells. Conversely, the expressions of Bax and caspase-3 were significantly increased in the hypoxia group, while resveratrol inhibited the hypoxia-induced increase of Bax and caspase. In addition, hypoxia significantly increased the ratio of Bax/Bcl-2 expression, but it was significantly decreased in the resveratrol-treated group.
Cardiovascular disease remains a leading cause of morbidity and mortality, even in developed countries. In France, however, where red wine is commonly taken with meals, the mortality rate from coronary heart disease is approximately half that of other Western countries, despite the presence of similar cardiovascular risk factors.1) This cardioprotective role of red wine is well-known worldwide as the "French paradox". It has been suggested that resveratrol(trans-3, 4', 5-trihydroxystilbene), a polyphenolic phytoalexin that is found in abundance in grape skins and red wines, may be the beneficial agent responsible for this protection.2) Resveratrol is present in the cis and trans isoforms, the latter is the biologically active form.
Some in vivo and in vitro studies have suggested that resveratrol is able to protect against coronary heart disease via significant antioxidant properties.3) Resveratrol may also exert cardioprotective action through carious other mechanisms, including inhibition of platelet aggregation,4) inhibition of endothelin-1 synthesis,5) vasorelaxation,6) and anti-inflammatory function.7) Recently, resveratrol has been found to protect kidney, brain, and heart cells from ischemia/reperfusion(I/R) injury.8-11)
I/R injury is a major complication of anginal syndromes, myocardial infarction, cardiopulmonary bypass surgery, and heart transplantation.12) Previously, myocardial cell death after I/R injury was considered to be mediated mainly by necrosis.13)14) However, it has recently been proposed that apoptosis can also contribute significantly to myocardial cell death after I/R injury.15) As cardiomyocytes have very limited ability to regenerate, death of these cells due to apoptosis, as well as necrosis, may play an important role in myocardial ischemia.12)16)
A recent report suggested that resveratrol inhibits the mitochondrial steps of the apoptotic process in the rat brain after hypoxia-reoxygenation.17) However, it remains unknown whether the protective effects of resveratrol in hypoxic myocardial cells are mediated through suppression of apoptosis. In this study, we investigated whether resveratrol confers cardioprotection against hypoxia via anti-apoptosis in an in vitro hypoxic model of cultured H9c2 cardiomyoblasts.
H9c2 cardiomyoblasts were obtained from the Korean Cell Line Bank(KCLB), and were maintained in Dulbecco's modified Eagle's medium(DMEM, GibcoBRL, NY, USA) supplemented with 10% fetal bovine serum (FBS, GibcoBRL, NY, USA) and antibiotic-antimycotic solution(GibcoBRL, NY, USA) in a 5% CO2 incubator at 37℃. Subcultured cells were grown for about 24 hours, to approximately 80% confluence prior to treatment.
The cultured cells were divided into four groups: a normal control group, a hypoxia group, a group treated with resveratrol(10 µg/mL) before hypoxic insult, and a group treated with resveratrol(10 µg/mL) after hypoxic insult. We increased the resveratrol concentration in the hypoxic medium to 100 µg/mL for the evaluation of an adequate resveratrol dosage, but it did not affect our data(data not shown). The control cells were placed in 5% CO2 incubators, and the hypoxia and resveratrol-treated groups were placed in 1% O2 incubators(94% N2, 5% CO2) for 24 hours, at which time the cells were collected and homogenized. The cell homogenates were stored at -70℃ before further processing.
H9c2 cells were lysed, and total protein was extracted using protein lysis buffer(50 mM, pH 8.0 Tris, 150 mM NaCl, 5 mM ethylenediaminetetraacetic acid(EDTA), 0.5% Nonidet P-40, 100 mM phenylmethylsulfonly fluoride(PMSF), 1 mg/mL leupeptin, 1 mg/mL aprotinin, 1 M 1, 4-dithio-DL-threitol(DTT)). Equal aliquots of proteins were boiled in loading buffer(100 mM Tris-HCl [pH 6.8], 200 mM DTT, 20% glycerol, 4% sodium dodecyl sulfate(SDS), and 0.2% bromopnenol blue) for 5 minutes. Samples containing equal amounts of protein(10 µg) were subjected to 12% SDS-polyacrylamide gel electrophoresis(SDS-PAGE), and proteins were then electro-transferred to polyvinylidine difluoride(PVDF) membranes. The membranes were blocked in TBS-T buffer(20 mM Tris-HCl [pH 7.5], 150 mM NaCl, 0.1% Tween-20) containing 5% nonfat milk for 1 hour at room temperature. Proteins were visualized by specific primary antibodies against Bcl-2(Santa Cruz Biotechnology, Santa Cruz, CA, USA), Bax(Cell Signaling Technology, Beverly, MA, USA), and caspase-3(Cell Signaling Technology, Beverly, MA, USA) at 4℃ overnight. After four washes in TBS-T buffer, the membranes were incubated with horseradish peroxidase-conjugated secondary antibodies(Santa Cruz Biotechnology, Santa Cruz, CA, USA) for 1 hour at room temperature. Immunoreactivity was detected using the Enhanced Chemiluminescence(ECL) Plus Western Blotting Detection System(Amersham Biosciences, Piscataway, NJ, USA).
The intensities of the Western blot bands were measured using a densitometer(Multi Gauge Software, Fuji Photofilm), and relative protein concentrations were expressed as the ratio of the signal intensity in the hypoxic group to that of the control group.
Total RNA was extracted with TRIzol reagent(Invitrogen Corporation, Carlsbad, CA, USA). In brief, total cultured cells were homogenized in 1 mL of TRIzol reagent, and total RNA was separated from DNA and proteins by extraction with chloroform and precipitation with isopropanol. The precipitate was washed twice in 75% ethanol, air-dried, and re-diluted in diethylpyrocarbonate(DEPC)-treated distilled water. The amount and purity of extracted RNA was quantified with a spectrophotometer(Beckman Coulter, Fullerton, CA, USA). The RNA was then stored at -70℃ pending further processing.
For reverse transcription, 1 µg total RNA was reverse transcribed for 1 hour at 37℃ in a reaction mixture containing 20 U RNase inhibitor(Promega, Madison, WI, USA), 1mM dNTP,(TaKaRa, Shiga, Japan), 0.5 ng Oligo(dT) 15 primer(Promega, Madison, WI, USA), 1×RT buffer, and 200 U MMLV reverse transcriptase (Promega, Madison, WI, USA). The reaction mixture was then heated at 95℃ for 5 minutes to stop the reaction. The newly transcribed cDNA was then stored at -20℃ pending further processing.
Real-time PCR was performed in 48-well PCR plates(Mini OpticonTM Real-Time PCR System, Bio-Rad, Hercules, CA, USA) using the Finnzymes DyNAmo SYBR green qPCR kit(Finnzymes, Beverly, MA, USA). Amplification conditions are shown in Table 1. The thermal profile was 95℃ for 15 minutes, followed by 40 cycles of denaturation, annealing, and extension as indicated in Table 1. Real-time PCR data were analyzed with LightCycler software(Bio-Rad, Hercules, CA, USA). Each sample was run in triplicate.
The H9c2 cells were observed by microscopy under high magnification(×400). The myocardial cells showed morphologically characterized changes of both apoptotic and necrotic cell death by hypoxia and resveratrol(10 µg/mL), which inhibited hypoxia-induced myocardial cell death.
The cells in the control group (A) were well preserved. Nuclear membranes were distinct, and the shape of the cytoplasm was normal. The cells in the hypoxia group (B) showed damage. Damaged cells showed cellular swelling, cell membrane disruption, and necrotic cellular debris. The nuclear shape was indistinct, and the cytoplasm showed numerous irregular vacuoles. Cells treated with resveratrol before a hypoxic insult (C) were preserved almost identically to those in the control group. The cellular shape was generally regular. The degree of cellular swelling and number of damaged cells were decreased compared to the hypoxia group, whereas cells treated with resveratrol after a hypoxic insult(D) were less well preserved than those treated with resveratrol prior to a hypoxic insult. The findings of cellular swelling and damaged cells are similar to the findings for the hypoxia group(Fig. 1).
The expression of Bcl-2, an anti-apoptotic marker, was significantly decreased in the hypoxia group(94.79) compared with the control group(100). Resveratrol treatment before hypoxic insult inhibited the hypoxia-induced decline in Bcl-2 immunoreactivity(96.74), but there was no significant inhibition of the hypoxia-induced decline in Bcl-2 in the resveratrol-treated group after hypoxic insult(91.64)(Fig. 2A). Conversely, the immunoreactivity of the pro-apoptotic marker, Bax, in the hypoxia group(107.93) was significantly increased compared to that of the control group(100). The hypoxia-induced incline in Bax was significantly inhibited in both resveratrol groups(97.68, 93.59)(Fig. 2B). The immunoreactivity of the other pro-apoptotic marker, caspase-3, was greater in the hypoxia group(105.73) than in the control group(100). The hypoxia-induced incline in caspase-3 was significantly inhibited in both resveratrol groups(93.82, 92.89)(Fig. 2C). The ratio of Bax/Bcl-2 expression was greater in the hypoxia group(1.14) than in the control group(1), and was significantly decreased in both resveratrol-treated groups(1.00, 1.02) compared with the hypoxia group(Fig. 2D).
The mRNA expression of the apoptotic marker, Bcl-2, in the hypoxia group(42.34) was significantly decreased as compared with the control group(100). Treatment with resveratrol prior to hypoxic insult significantly inhibited the hypoxia-induced decline in Bcl-2 mRNA expression(114.08), but there no significant change was observed in the resveratrol-treated group after hypoxic insult(25.17)(Fig. 3A). In contrast, the mRNA expression of the pro-apoptotic marker, Bax, in the hypoxia group (179.98) was significantly increased compared to that in the control group(100). The hypoxia-induced incline in Bax was significantly inhibited in both resveratrol groups, and the inhibition was greater in the resveratrol group treated before hypoxic insult(70.43) than in the group treated after hypoxic insult(114.65)(Fig. 3B). The mRNA expression of the other pro-apoptotic marker, caspase-3, was greater in the hypoxia group (121.42) than in the control group(100). The hypoxia-induced incline in caspase-3 was significantly inhibited in both of the resveratrol groups(105.70, 91.38)(Fig. 3C). The ratio of Bax/Bcl-2 expression was greater in the hypoxia group(4.25) than in the control group (1), and was significantly decreased in the resveratrol group treated before hypoxic insult(0.62). However, there was no significant change in the group treated after hypoxic insult(4.55) compared with the hypoxia group(Fig. 3D).
At the cellular/molecular level, resveratrol acts by modulating numerous intracellular target proteins,18) but little is known about the exact cellular and molecular mechanisms by which resveratrol protects against coronary heart diseases, including myocardial dysfunction. Recently, resveratrol has been shown to exert cardiovascular benefits in the rat heart after I/R injury.10)11)
Apoptosis plays a central role in homeostasis of an organ, and in disease processes. In apoptosis, the cell undergoes an ordered disassembly, which is followed by death and phagocytosis; the process of disassembly is characterized by cell shrinkage, chromatin condensation, membrane blebbing, and preservation of organelle ultrastructural integrity.13)19) Two major signaling mechanisms of apoptosis are well-described: the receptor-dependent death pathway and the mitochondrial-dependent death pathway.12)20) In the receptor-dependent death pathway, cell surface "death receptors" are activated.21) Upon activation, intracellular adapter proteins are recruited by these receptors, which subsequently stimulate the activation of caspase-8 and initiate a well-defined caspase cascade that leads to apoptotic cell death. Alternatively, in the mitochondrial-dependent death pathway, increases in mitochondrial permeability lead to the release of cytochrome c and the activation of caspase-9, which initiates the downstream caspase pathway and results in activation of the effector caspase 3, also leading to apoptosis.12)20)
It has been demonstrated that hypoxia increases the expression of Bax, a pro-apoptotic protein, in myocardial cells. It has also been shown that translocation of cytosolic Bax into mitochondria is an important trigger for the release of mitochondrial cytochrome c into cytosol.22) In contrast, Bcl-2, a representative anti-apoptotic protein, is demonstrated to prevent the release of cytochrome c and to mediate anti-apoptotic effects.23) Previous observations have also indicated that Bcl-2 and Bax play important pathophysiological roles in the protection and acceleration of apoptosis in human myocytes after ischemia and/or reperfusion.20)24)
The family of caspases is also a key regulator of the apoptotic signaling pathway.25) In the apoptotic process, inactive procaspases are cleaved into their active forms by other activated caspases. Activated executioner caspase- 3 can cleave additional downstream substrates that are involved in apoptotic changes.26) The cleavage of procaspases has been used as an indicator of apoptosis.
We studied the cardioprotective effect of resveratrol using an in vitro culture model of hypoxia. Apoptosis was assayed by cytological analysis with Western blotting and real-time PCR for Bcl-2, Bax, and caspase-3. The present study revealed that, in cultured H9c2 cells, cells treated with resveratrol showed better morphological preservation than those in the hypoxia group, whether treated before or after a hypoxic injury.
Apoptosis as a result of hypoxic ischemia(HI) is morphologically different from developmental apoptosis, and many hybrid necrotic-apoptotic phenotypes are normally seen.27) In contrast, from a biochemical perspective, apoptotic processes are involved in HI. Indeed, key factors in apoptosis such as caspase-3,28) Bcl-2,29) and Bax30) are regulated, and have been postulated to play prominent roles in pathological situations. From the results of many studies, including those described above, it is evident that Bcl-2 is anti-apoptotic in nature, whereas Bax and caspase-3 are pro-apoptotic. Caspase-3, a widely studied caspase, plays a role as an effector in myocardial cell death during HI insult.
In the present study, the expressions of Bcl-2, Bax, and caspase-3 were assayed by Western blot analysis and real-time PCR in cultured H9c2 cells. It has been shown that hypoxia significantly increased the ratio of Bax/Bcl-2 expression and caspase-3, which were involved in the apoptotic death pathway in the myocardial cells. Our study also demonstrates that resveratrol inhibits the hypoxia-induced decline of Bcl-2 in hypoxic myocardial cells, and also inhibits the hypoxia-induced increase of Bax and caspase. Therefore, these data suggest that the cardioprotective effect of resveratrol works in the mitochondrial death signaling pathway, via an anti-apoptotic mechanism.
The present study has shown that hypoxic injury of myocardial cells resulted in morphological changes to both apoptotic and necrotic cell death, and that resveratrol(10 µg/mL) treatment prior to hypoxic insult inhibited hypoxia-induced myocardial cell death, whereas resveratrol treatment after a hypoxic insult showed less inhibition of hypoxia-induced cell death. It seems that the cardioprotective effect of resveratrol in hypoxic injury is greater when it is administered before a hypoxic insult than when it is administered after a hypoxic insult.
We found that hypoxia significantly decreased the expression of the anti-apoptotic factor(Bcl-2), mRNA, and protein, and that treatment with resveratrol before hypoxic insult significantly inhibited the hypoxia-induced decline in Bcl-2 expression. However, there was no significant inhibition of the hypoxia-induced decline in Bcl-2 in the resveratrol-treated group after hypoxic insult. This may partially explain why the effect of resveratrol is greater in the resveratrol group treated before hypoxic insult than in the group treated after hypoxic insult in the morphologic study. Conversely, hypoxia significantly increased Bax and caspase-3 mRNA and protein expression, and the hypoxia-induced increases of Bax and caspase-3 were significantly inhibited in both resveratrol groups. The ratio of Bax/Bcl-2 expression was increased in the hypoxia group as compared with the control group. The ratio was increased 4.25-fold using the real-time PCR method, but was increased only 1.14-fold using the Western blot method. In addition, the ratio was decreased by the Western blot method in both of the resveratrol-treated groups, but was decreased only in the resveratrol-treated group before hypoxic insult using the real-time PCR method. This could have happened due to differences between the methods of study and their sensitivities.
As stated above, there was a discrepancy in the results for the resveratrol-treated groups before and after hypoxic insult. It is likely that the effect of resveratrol on Bcl-2 is greater in the viable myocardial cells before a hypoxic insult than in damaged cells after hypoxic insult. The effect of resveratrol on Bax and caspase-3 may be less affected by the cell viability, and it is possible that the effects of resveratrol on Bcl-2, Bax, and caspase-3 may function independently. Further investigation will be needed to fully clarify other molecular mechanisms by which resveratrol exerts its cardioprotective effects against hypoxic injury.
In conclusion, the present study demonstrates that resveratrol can regulate the expressions of Bcl-2, Bax, and caspase-3 proteins in mitochondria, and suppress the mitochondrial death pathway in a model of hypoxic injury in myocardial cells. These effects are likely to play a role in resveratrol-mediated cardioprotection, and may have significant implications in the development of future therapies for hypoxic myocardial injury. However, more experiments are needed in order to test the usefulness of resveratrol as a preventive measure and as a treatment for hypoxic myocardial injury.
Figures and Tables
Fig. 1
High magnification (×400) photomicrographs of cultured H9c2 cells. A: control group; nuclear membranes are distinct and the shape of the cytoplasm is regular. B: hypoxia group; the picture shows cellular swelling. Damaged cells show cell membrane disruption and necrotic cellular debris. Nuclear shape is indistinct, the cytoplasm shows numerous irregular vacuoles. C: resveratrol group treated before hypoxic exposure; the cellular shape is generally regular. The degree of cellular swelling and number of damaged cells are decreased compared to those of (B, D). Resveratrol group treated after hypoxic exposure: findings of cellular swelling and damaged cells are similar to those of (B).
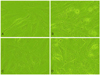
Fig. 2
Western blotting of Bcl-2 (A), Bax (B), and caspase-3 (C) from cultured H9c2 cells. The ratio of Bax/Bcl-2 (D) expression is also shown. Resveratrol was administered at 10 µg/mL. Immunoblots from three independent experiments are shown. Densitometric analysis is also shown. Data are presented as the ratios of band intensities compared with the control group. Before: resveratrol group treated before hypoxic exposure, After: resveratrol group treated after hypoxic exposure. *: p<0.05 compared with control.
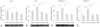
Fig. 3
Real-time PCR was performed for Bcl-2 (A), Bax (B), and caspase-3 (C) on DNA from cultured H9c2 cells. The ratio of Bax/Bcl-2 (D) expression is also shown. Resveratrol was administered at 10 µg/mL. Data are presented as the ratios of mRNA expression compared with those of the control group. Before: resveratrol group treated before hypoxic exposure, After: resveratrol group treated after hypoxic exposure. *: p<0.05 compared with control. PCR: polymerase chain reaction, DNA: deoxyribonucleic acid, mRNA: messenger ribonucleic acid.
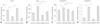
References
1. Renaud S, de Lorgeril M. Wine, alcohol, platelets, and the French paradox for coronary heart disease. Lancet. 1992. 339:1523–1526.
2. Kopp P. Resveratrol, a phytoestrogen found in red wine: a possible explanation for the conundrum of the 'French paradox'? Eur J Endocrinol. 1998. 138:619–620.
3. Cao Z, Li Y. Potent induction of cellular antioxidants and phase 2 enzymes by resveratrol in cardiomyocytes: protection against oxidative and electrophilic injury. Eur J Pharmacol. 2004. 489:39–48.
4. Pace-Asciak CR, Hahn S, Diamandis EP, Soleas G, Goldberg DM. The red wine phenolics trans-resveratrol and quercetin block human platelet aggregation and eicosanoid synthesis: implications for protection against coronary heart disease. Clin Chim Acta. 1995. 235:207–219.
5. Khan NQ, Lees DM, Douthwaite JA, Carrier MJ, Corder R. Comparison of red wine extract and polyphenol constituents on endothelin-1 synthesis by cultured endothelial cells. Clin Sci. 2002. 103:Suppl 48. 72S–75S.
6. Naderali EK, Doyle PJ, Williams G. Resveratrol induces vasorelaxation of mesenteric and uterine arteries from female guineapigs. Clin Sci. 2000. 98:537–543.
7. Das S, Cordis GA, Maulik N, Das DK. Pharmacological preconditioning with resveratrol: role of CREB-dependent Bcl-2 signaling via adenosine A3 receptor activation. Am J Physiol Heart Circ Physiol. 2005. 288:H328–H335.
8. Giovannini L, Migliori M, Longoni BM, et al. Resveratrol, a polyphenol found in wine, reduces ischemia reperfusion injury in rat kidneys. J Cardiovasc Pharmacol. 2001. 37:262–270.
9. Huang SS, Tsai MC, Chih CL, Hung LM, Tsai SK. Resveratrol reduction of infarct size in Long-Evans rats subjected to focal cerebral ischemia. Life Sci. 2001. 69:1057–1065.
10. Ray PS, Maulik G, Cordis GA, Bertelli AA, Bertelli A, Das DK. The red wine antioxidant resveratrol protects isolated rat hearts from ischemia reperfusion injury. Free Radic Biol Med. 1999. 27:160–169.
11. Bradamante S, Barenghi L, Piccinini F, et al. Resveratrol provides late-phase cardioprotection by means of a nitric oxide-and adenosine-mediated mechanism. Eur J Pharmacol. 2003. 465:115–123.
12. Lee SH, Kim YD. Death and survival of cardiomyocytes in acute ischemia. Korean Circ J. 2006. 36:165–177.
13. Kerr JF, Wyllie AH, Currie AR. Apoptosis: a basic biological phenomenon with wide-ranging implications in tissue kinetics. Br J Cancer. 1972. 26:239–257.
14. Kim YK, Han DS, Lee MY, et al. Apoptosis in ischemia-reperfused myocardiaum of rabbit. Korean Circ J. 1997. 27:1017–1026.
15. Yaoita H, Ogawa K, Maehara K, Maruyama Y. Apoptosis in relevant clinical situations: contribution of apoptosis in myocardial infarction. Cardiovasc Res. 2000. 45:630–641.
16. Nadal-Ginard B, Kajstura J, Leri A, Anversa P. Myocyte death, growth, and regeneration in cardiac hypertrophy and failure. Circ Res. 2003. 92:139–150.
17. Morin C, Zini R, Albengres E, Bertelli AA, Bertelli A, Tillement JP. Evidence for resveratrol-induced preservation of brain mitochondria functions after hypoxia-reoxygenation. Drugs Exp Clin Res. 2003. 29:227–233.
18. Dore S. Unique properties of polyphenol stilbenes in the brain: more than direct antioxidant actions; gene/protein regulatory activity. Neurosignals. 2005. 14:61–70.
19. Seo HS. Apoptosis in cardiovascular system. Korean Circ J. 1997. 27:793–799.
20. Joza N, Kroemer G, Penninger JM. Genetic analysis of the mammalian cell death machinery. Trends Genet. 2002. 18:142–149.
21. Ashkenazi A, Dixit VM. Death receptors: signaling and modulation. Science. 1998. 281:1305–1308.
22. Tatsumi T, Shiraishi J, Keira N, et al. Intracellular ATP is required for mitochondrial apoptotic pathways in isolated hypoxic rat cardiac myocytes. Cardiovasc Res. 2003. 59:428–440.
23. Yang J, Liu X, Bhalla K, et al. Prevention of apoptosis by Bcl-2: release of cytochrome c from mitochondria blocked. Science. 1997. 275:1129–1132.
24. Misao J, Hayakawa Y, Ohno M, Kato S, Fujiwara T, Fujiwara H. Expression of bcl-2 protein, an inhibitor of apoptosis, and Bax, an accelerator of apoptosis, in ventricular myocytes of human hearts with myocardial infarction. Circulation. 1996. 94:1506–1512.
25. Alnemri ES, Livingston DJ, Nicholson DW, et al. Human ICE / CED-3 protease nomenclature. Cell. 1996. 87:171.
26. Kaufmann SH, Desnoyers S, Ottaviano Y, Davidson NE, Poirier GG. Specific proteolytic cleavage of poly (ADP-ribose) polymerase: an early marker of chemotherapy-induced apoptosis. Cancer Res. 1993. 53:3976–3985.
27. Leist M, Jaattela M. Four deaths and a funeral: from caspases to alternative mechanisms. Nat Rev Mol Cell Biol. 2001. 2:589–598.
28. de Moissac D, Gurevich RM, Zheng H, Singal PK, Kirshenbaum LA. Caspase activation and mitochondrial cytochrome C release during hypoxia-mediated apoptosis of adult ventricular myocytes. J Mol Cell Cardiol. 2000. 32:53–63.
29. Jung F, Weiland U, Johns RA, Ihling C, Dimmeler S. Chronic hypoxia induces apoptosis in cardiac myocytes: a possible role for Bcl-2-like proteins. Biochem Biophys Res Commun. 2001. 286:419–425.
30. Dong JW, Zhu HF, Zhu WZ, Ding HL, Ma TM, Zhou ZN. Intermittent hypoxia attenuates ischemia/reperfusion induced apoptosis in cardiac myocytes via regulating Bcl-2/Bax expression. Cell Res. 2003. 13:385–391.