Abstract
Purpose
To elucidate the relation between fracture healing and angiogenesis, we checked expression of Hypoxia-inducible factor (HIF) and Vascular endothelial growth factor (VEGF) in hypoxic cell cultures and the callus from a rat femur fracture model.
Materials and Methods
Human osteoblasts, chondrocytes, and rat ST2 cells were cultured in DME/F12 media with 10% FBS. Hypoxic DME/F12 media (PO2<60 mmHg) was generated by bubbling with 95% N2 and 5% CO2 and added to cells. After 2, 6, and 24 hours, RNA and proteins were collected for reverse transcription - polymerase chain reaction (RT-PCR) and Western blot. In addition, immunocytochemistry and siRNA treatment for HIF-1α were performed. Next, femurs from 9-week SD rats were fractured after fixation with needles. The rats were sacrificed at post-fracture day (PFD) 3, 5, 7, 10, 14, 21 and calluses were collected for RT-PCR and Western blot.
Results
HIF-1α and HIF-2α expression were not increased in RT-PCR but protein levels were increased. VEGF expression in RT-PCR was increased. Treatment with siRNA directed towards HIF inhibited VEGF expression. In the rat fracture callus, HIF-1α and VEGF expression peaked between PFD 5 and 7 and decreased after PFD 10. In contrast to cell culture, mRNA expression of HIF-1α was increased at PFD 7.
Figures and Tables
Fig. 1
Hypoxia does not induce HIF-1α mRNA expression (A, B) but increases protein levels (C, D). N, normoxia; H, hypoxia.
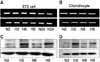
Fig. 4
Hypoxia does not induce HIF-1α and HIF-2α mRNA expression but increases protein levels. HIF-1α, HIF-2α and VEGF expression in human osteoblasts shows similar expression patterns to ST2 cells and chondrocytes.
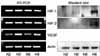
Fig. 6
VEGF expression was decreased by treatment with siRNA for HIF-1α in human osteoblasts. Mock, transfection control.
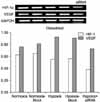
Fig. 7
VEGF expression was decreased by treatment with siRNA for HIF-1α in human chondrocytes. Mock, transfection control.
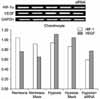
Fig. 8
Western blot for HIF-1α in callus shows elevation at early stages of fracture healing (peak at PFD 5) and decreases after PFD 10.
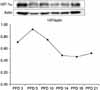
References
1. Ashton N, Ward B, Serpell G. Effect of oxygen on developing retinal vessels with particular reference to the problem of retrolental fibroplasia. Br J Ophthalmol. 1954. 38:397–432.


2. Brighton CT, Hunt RM. Early histological and ultrastructural changes in medullary fracture callus. J Bone Joint Surg Am. 1991. 73:832–847.


3. Choi P, Ogilvie C, Thompson Z, Miclau T, Helms JA. Cellular and molecular characterization of murine non-union model. J Orthop Res. 2004. 22:1100–1101.
4. Folkman J, Merler E, Abernathy C, Williams G. Isolation of a tumor factor responsible for angiogenesis. J Exp Med. 1971. 133:275–288.


5. Forsythe JA, Jiang BH, Iyer NV, et al. Activation of vascular endothelial growth factor gene transcripthion by hypoxia-inducible factor 1. Mol Cell Biol. 1996. 16:4604–4613.
6. Gerstenfeld LC, Cullinane DM, Barnes GL, Graves DT, Einhorn TA. Fracture healing as a post-natal developmental process: molecular, spatial, and temporal aspects of its regulation. J Cell Biochem. 2003. 88:873–884.


7. Gleadle JM, Ebert BL, Firth J, Ratcliffe PJ. Regulation of angiogenic growth factor expression by hypoxia, transition metals, and chelating agents. Am J Physiol. 1995. 268:C1362–C1368.


8. Goldberg MA, Schneider TJ. Similarities between the oxygen-sensing mechanisms regulating the expression of vascular endothelial growth factor and erythropoietin . J Biol Chem. 1994. 269:4355–4359.


9. Grundnes A, Reikerås O. The importance of the hematoma for fracture healing in rats. Acta Orthop Scand. 1993. 64:340–342.


10. Jaakkola P, Mole DR, Tian YM, et al. Targeting of HIF-alpha to the von Hippel-Lindau ubiquitylation complex by O2-regulated prolyl hydroxylation. Science. 2001. 292:468–472.
11. Jelkmann W. Erythropoitin: structure, control of production, and function. Physiol Rev. 1992. 72:449–489.
12. Komatsu DE, Hadjiargyrou M. Activation of the transcription factor HIF-1 and its target genes, VEGF, HO-1, iNOS, during fracture repair. Bone. 2004. 34:680–688.


13. Kourembanas S, Hannan RL, Faller DV. Oxygen tension regulates the expression of the platelet-derived growth factor-β chain gene in human endothelial cells. J Clin Invest. 1990. 86:670–674.
14. Krishnamachary B, Berg-Dixon S, Kelly B, et al. Regulation of colon carcinoma cell invasion by hypoxia-inducible factor 1. Cancer Res. 2003. 63:1138–1143.
15. Lando D, Peet DJ, Gorman JJ, Whelan DA, Whitelaw ML, Bruick RK. FIH-1 is an asparaginyl hydroxylase enzyme that regulates the transcriptional activity of hypoxia-inducuble factor. Genes Dev. 2002. 16:1466–1471.
16. Lee JW, Bae SH, Jeong JW, Kim SH, Lim KW. Hypoxia-inducible factor (HIF-1) alpha: its protein stability and biological functions. Exp Mol Med. 2004. 36:1–12.
17. Marsh D. Concepts of fracure union, delayd union, and nonunion. Clin Orthop Relat Res. 1998. 355:Suppl 355. S22–S30.
18. Mizuno K, Mieno K, Tachibana T, Sumi M, Hirohata K. The osteogenetic potential of fracture haematoma. Subperiosteal and intramuscular transplantation of the haematoma. J Bone Joing Surg Br. 1990. 72:822–829.


19. Pufe T, Wildemann B, Petersen W, Mentlein R, Raschke M, Schmidmaier G. Quantitative measurement of the spilce variants 120 and 164 of the angiogenic peptide vascular endothelial growth factor in the time flow of fracture healing: a study in the rat. Cell Tissue Res. 2002. 309:382–392.
20. Pugh CW, Ratcliffe PJ. Regulation of angiogenesis by hypoxia: role of the HIF system. Nat Med. 2003. 9:677–684.


21. Shweiki D, Itin A, Soffer D, Keshet E. Vascular endothelial growth factor induced by hypoxia may mediate hypoxia-initiated angiogenesis. Nature. 1992. 359:843–845.


22. Street J, Bao M, deGuzman L, et al. Vascular endothelial growth fator stimulates bone repair by promoting angiogenesis and bone turnover. Proc natl Acad Sci USA. 2002. 99:9656–9661.
23. Thomlinson RH, Gray LH. The histological structure of some human lung cancers and the possible implications for radiotherapy. Br J Cancer. 1955. 9:539–549.

