Abstract
Background
Osteoporosis of transplanted/grafted bone is well known in the immediate post bone transplantation/bone grafting period. In limb transplantation, the growth plates in the transplanted limbs retain their longitudinal growth properties. However, there is a paucity of reports on what happens to the bone and the growth potential of the growth plate when limb transplantation between a juvenile donor and an adult recipient is performed.
Materials and Methods
Ten juvenile to juvenile hind limb transplants and five juvenile to adult hind limb transplants were performed in male syngeneic Lewis rats. Osteoporosis in the isochronograft as well as the heterochronograft limbs was measured by 3D micro-CT. In addition, the increase in tibial length, after transplantation was measured and compared with the increase in the tibial length of the opposite non-operated limbs.
Results
The 3D CT parameters indicate a significantly inferior bone quality in the heterochronografts compared with the isochronografts. After transplantation, the increase in the tibial length of the isochronografts was similar the increase in length of the opposite juvenile non operated tibiae and the heterochronograft tibias.
Conclusion
Age is a significant factor that affects the bone quality, resulting in post transplant osteoporosis in heterochronografts compared with isochronografts. However, the growth plate after transplantation remains unaffected by the difference in age and continues to grow at its own inherent rate in adult recipients as it does in the juvenile recipients.
It is known that osteoporosis develops in the grafted or transplanted bone after bone transplantation or bone grafting. This phenomenon occurs due to the revascularization and creeping substitution during the repair process.
Each growth plate has an inherent mechanism for determining the growth rate and ultimate limb morphology3), but the process is also influenced by a variety of hormones that have a permissive effect on enabling the growth plate to achieve its maximum growth potential. Growth hormone, thyroxine, somatomedin C, insulin like growth factor I, insulin like growth factor II, cortisol, insulin, and sex hormones all play important roles in regulating the development of the growth plate as well as the rate of limb growth5-9).
Limb transplantation between male syngeneic Lewis rats of different ages provides a unique experimental model that is made possible by the advances in micro vascular surgery that allow an examination of post bone transplantation osteoporosis and the growth potential of the donor growth plates. The aim of this study was to determine the correlation between the bone quality and growth potential with age as a recipient dependent factor after limb transplantation by comparing the degree of osteoporosis and growth of the limb that develop after limb transplantation between animals of different ages.
The main focus was on what occurs when a growth plate with significant growth potential is placed into an adult environment i.e. do they still retain their property of promoting longitudinal growth in the changed milieu? If the answer is yes, then is this increase in length different from the increase in length occurring in the juvenile environment? This study also examined whether or not the difference in age has an effect on the density of the transplanted bone. Several studies have examined the behavior of a juvenile growth plate in an adult body by limb transplantation between animals of different ages. However, the reported results are conflicting. Some studies reporting that the juvenile growth plate attains its full growth potential in an adult body3), while other studies claim that the juvenile growth plate attains only a part of its growth potential in an adult hormonal environment10,11). One of the aims of this study was to provide valuable information that could be applied clinically to vascularized growth plate transplantations. Vascularized growth plate transplantation is becoming a feasible alternative for the management of growth arrest due to growth plate damage by tumors, infections, trauma or congenital anomalies.
There are several reports where microvascular epiphyseal transplantation has been used to reconstruct the extremities in children whose epiphyseal plates have been damaged or surgically removed as a result of disease or trauma12-16). This serves the dual purpose of reconstruction of a bony defect as well as the restoration of longitudinal growth.
Male syngeneic Lewis rats were selected for this study. Two groups of rats differing only in age were used in this study. The first group consisted of juvenile rats (mean 23.8 days, range 21-28 days). The second group consisted of adult rats (mean 72.4 days, range 71-77 days). The hind limbs of these rats were divided into the following four experimental groups:
Group 1: Ten juvenile to juvenile right hind limb isochronografts.
Group 2: The non-operated contra lateral left hind limbs of the 10 juvenile rats in Group 1.
Group 3: Five juvenile to adult right hind limb heterochronografts.
Group 4: The non-operated contra lateral left hind limbs of the adult rats in Group 3.
The surgical procedure was performed under general anesthesia, which was induced and maintained by a single intraperitoneal dose of ketamine. Two surgical teams were involved. One team was dedicated to harvesting the donor limb from the 15 juvenile rats while the other team performed the microvascular anastomosis of the donor limb to the recipient stumps of 10 juvenile and five adult rats. The donor juvenile limb was harvested using an above the knee amputation at the mid-femur diaphysis level. The femoral vessels of the donor limbs were the last structures divided in an attempt to keep the ischemia time to less than one hour. This was followed by the microvascular anastomosis of the donor limb to the recipient above the Knee amputation stumps of 10 juvenile and five adult rats. Bony stabilization was first achieved by inserting an intramedullary 18G needle into the femur, which was followed by microvascular anastomosis of the femoral vessels using 10-0 Nylon interrupted sutures. Femoral and sciatic nerve anastomoses were then performed with 10-0 Nylon, and finally the corresponding muscle groups were sutured. All the rats received prophylactic antibiotics in the form of an intramuscular injection of Penicillin 1 postoperatively. After the transplant, the rats were housed separately and were allowed to bear weight on the transplanted limbs when the pain permitted. The increase in the length of the transplanted limbs was then monitored by calculating the tibial lengths on the serial postoperative radiographs. The first set of radiograms was taken three weeks after transplantation. These radiograms were taken after sedating the rats in order to allow correct positioning of the limbs for the radiograms. All the animals were sacrificed at 10 weeks after the transplant by administering an overdose of intraperitoneal Thiopentone. A second set of radiograms was taken at this time. The increase in length of the tibia of the transplanted limbs was then measured by calculating the difference in the lengths of the tibia at the three and 10 weeks post-transplantation radiograms. Similarly, the increase in length of the tibia of the contra lateral non-operated hind limbs during the same time interval was measured in all animals.
In order to ensure accuracy, all tibial lengths were measured using the software program Rapidia Version 2.7 (INFINITT, Seoul, Korea), which also corrected for the errors due to X-ray magnification. In order to measure the degree of osteoporosis in the transplanted limbs, the tibias of both, the isochronografts and heterochronografts, were harvested from the sacrificed animals. After stripping the harvested tibias of all soft tissues the bones were prepared by precision sawing and subjected to a high resolution three-dimensional micro-CT analysis (µ-CT-40, Scanco Medical AG, Zurich, Switzerland). The scanned images had a three-dimensional reconstruction of the cubic voxel sizes 31×31×31 µm3. Each three dimensional image dataset consisted of approximately 200 micro-CT slide images (1024×1024 pixels) with 16 bit gray levels. The micro CT images were segmented from the accurate three dimensional data sets using a previously described method (Ding et al, 1999). The following parameters were examined: bone volume fraction (VF), Trabecular Number (Tb.N), Trabecular Thickness (Tb.Th), Trabecular Separation (Tb.Sp), Bone Surface to Total Volume ratio (BS/TV) and Bone Surface to Bone Volume ratio (BS/BV) were calculated based on unbiased, assumption free three dimensional methods. The micro CT parameter values from the isochronograft group were then compared with the heterograft measures using an unpaired Student's t test. A p-value<0.05 was considered significant. Comparisons were then made between the increase in the tibial lengths of the transplanted isochronograft (juvenile to juvenile) limbs and the increase in the tibial lengths of the corresponding contra lateral non-operated hind limbs in the same animals (Group 1 v/s Group 2).
Similarly comparisons were made between the increase in the tibial lengths of the transplanted isochronograft limbs and the transplanted heterochronograft (juvenile to adult) limbs (Group 1 v/s Group 3). Finally, comparisons were made between the increase in the tibial lengths of the heterochronograft limbs and the corresponding contra lateral non-operated hind limbs in the adult animals (Group 3 v/s Group 4).
Statistical analysis of the obtained data was then performed. The limbs from completely separate animals (e.g., juvenile to juvenile isochronografts versus juvenile to adult heterochronografts) were compared using a student's t test for independent groups, while the limbs from the same animal (e.g., juvenile to juvenile isochronografts versus contralateral nonoperated limbs of the juvenile animals) were compared using a student's t test for the correlated groups. A p-value<0.05 was considered significant. University Animal Care and Use Committee (ACUC) approval was obtained before commencing the study.
The parameter values for bone mass indicated a significantly inferior bone quality in the transplanted bone in the heterochronograft group compared with the bone quality of the isochronograft group. The 3D fractional bone volume (VF) from the heterochronograft group was significantly lower than the bone volume fraction of the isochronograft group (p=0.0096). In the heterochrongraft group, the values for the trabecular number (Tb.N) were significantly smaller (p=0.001) than those from the isochronograft group. The values for the trabecular separation (Tb.Sp) were significantly higher in the heterochronograft group than in the isochronograft group (p<0.001) indicating that the trabecular density of the heterochronograft group was significantly lower than that of the isochronograft group. The trabecular thickness (Tb.Th) was significantly lower in the heterochronograft group than in the isochronograft group (p=0.028) indicating that the trabeculae in the heterochronograft group was significantly thinner and weaker than in the isochronograft group. The bone surface to total volume ratio (BS/TV) derived from the heterochronograft group was significantly smaller than that from the isochronograft group (p<0.001). The bone surface to bone volume ratio was significantly higher (p=0.0080) in the heterochronograft group than in the isochronograft group.
During the experimental period, the tibia in the juvenile isochronograft limbs grew in length by 1.23±0.13 cm (range 1.0 to 1.4 cm). This was not significantly different (p=0.66) from the increase in tibial length from the non-operated contra lateral hind limb of these juvenile animals, which grew by a total of 1.28±0.19 cm (range 1.0 to 1.7 cm) during the same period.
The tibia from the juvenile isochronograft hind limbs grew in length by 1.23±0.13 cm (range 1.0 to 1.4 cm) during the experimental period, which was not significantly different (p=0.61) from the increase in tibial length from the juvenile to adult heterochronograft hind limbs, which grew by 1.22±0.18 cm (range 1.0 to 1.4 cm) during the same time interval.
During the experimental period, the tibia of the juvenile to adult heterochronograft hind limbs increased in length by 1.22±0.18 cm (range 1.0 to 1.4 cm), which was different (p<0.001) from the increase in tibial length from the adult non-operated contra lateral hind limbs, which increased in length by 0.50±0.30 cm (range 0 to 0.8 cm) during the same time interval (Tables 1, 2, 3, Fig. 1, 2)
Osteoporosis of transplanted/grafted bone in the immediate post bone transplantation/bone grafting period is well known. Steinberg et al4) showed by radiographic and histological studies that cortical grafts had decreased density during the first six months but gradually regained their mass to the normal cortical bone at two years. They concluded that a creeping substitution remodeling process might take up to two to three years.
Buchardt1,2) reported that there are three differences between cancellous and cortical bone incorporation into the host bone after a bone graft or bone transplantation: in the revascularization phase, in the initiation of the creeping substitution phase and in the repair phase. The mechanical strength and bone mass of cancellous and cortical grafts are related to their respective repair process. There is an increase in bone apposition in the cancellous bone and a reverse process in the cortical bone during the revascularization and creeping substitution phases. The cancellous graft/transplant tends to be strengthened first whereas cortical transplants are weakened. An allograft incorporates at a slower rate than an autograft. Both vascular penetration and bone formation are slower and less extensive.
Kline et al3), reported the occurrence of osteoporosis after limb transplantation in animals of different ages. However, they did not establish a correlation between the degree of osteoporosis and the age of the recipient animal because that study primarily evaluated the growth characteristics of the growth plate after limb transplantation. Kline attributed the post transplantation osteoporosis to non weight bearing and a lack of external stress after limb transplantation. Non weight bearing and a lack of external stress may play some role in post transplantation osteoporosis but the significant difference in bone quality between isochronografts and heterochronografts suggests that age also plays a role in the etiology and pathogenesis of post transplantation osteoporosis. These experimental findings suggest that the bone quality after a bone graft/transplant also depends on the age of the recipient
The results of the above experiment suggest that even after the transplantation of limbs from juvenile animals to adult animals (heterochronografts), the growth plates in the transplanted limbs retain their property of longitudinal growth and they continue to grow at the same rate in the new adult environment, as they would have in the juvenile environment. The increase in length of the heterochronograft limbs was similar to the increase in length of the isochronograft limbs. In addition, the increase in length of the isochronograft limbs was similar to the increase in the length of the non operated contra lateral hind limbs. However, the increase in length of the heterochronograft limbs was significantly less than the increase in length of the non-operated contra lateral hind limbs of the adult rats. Therefore, after three weeks of age, the local environment of the host ceases to have a decisive role in determining the growth characteristics of the growth plate, and the increase in length of the growth plate is determined primarily by local transplantable factors expressed before transplantation through an interaction between the inducing factors and the inherited genomes.
The increase in the length of the adult non-operated limbs can be explained by the fact that the growth pattern in rats differs from that in humans, in that the growth plates in rats remain open until well into adulthood. This is despite the fact that the growth rate observed at 10 weeks of age was a fraction of that observed at three weeks of age17,18). For this reason, caution should be taken when extrapolating these results to humans, and that these findings will require confirmation in a clinical setting for human patients.
Kline et al also examined the growth plate morphology in transplanted limbs and observed that all transplanted limbs maintained the growth plate morphology and columnar organization. These results are consistent with those reported by Kline et al3), who showed that the adult environment does not inhibit the longitudinal growth of an immature growth plate.
Glickman et al20) examined epiphyseal growth after microvascular transplantation to the sites of a different growth potential and reaffirmed that the growth potential of an epiphyseal plate transplant is a function of the donor regardless of the recipient site to which it is transplanted. They hoped their study would provide useful information for the future transplantation of epiphyseal plates or extremity allografts, which might provide the ideal biological solution for clinical problems involving damage to the epiphyseal plate damage by an infection or trauma, or an absence resulting from tumor resection or congenital malformations.
Chiu et al10) reported a similar study involving limb transplantation between animals of different ages, and observed that the transplanted bone could achieve only 70% of its normal growth in length. This finding was corroborated by Drzewiecki et al11) who also found that after limb transplantation, the transplanted bone could not achieve its normal growth potential but noted that the maximum growth (91% of normal) occurred in heterochronografts. However, in both studies, the nerves of the transplanted limbs were not sutured and the animals were kept in non weight bearing environments. Hence, the failure to achieve full growth could be attributed to the effects of denervation and a lack of external stress. Stevens et al19) examined the growth of epiphyseal plate allografts after micro vascular transplantation in rabbits of different ages and found that the growth rate depended on the age of the donor epiphyseal plate and was independent of the age of the recipient.
There are reports in the literature where microvascular epiphyseal transplant has been used to reconstruct extremities in children whose epiphyseal plates have been damaged or surgically removed as a result of disease or trauma12-16). Innocenti et al12-15) described the treatment for a loss of the distal part of the radius, including the physis and epiphysis in skeletally immature patients by performing a vascularized proximal fibula transfer based on the anterior tibial artery, including the physis and a variable length of the diaphysis, and reported a consistent and predictable longitudinal growth of the transferred fibula. Based their findings, Innocenti et al proposed that in cases of loss or damage of the epiphyseal plates, vascularized epiphyseal transfer is the only possible procedure that can solve the dual problem of replacement of an osseous defect and the restoration of longitudinal growth. Vilkki16) performed microvascular transplantation of a metatarsophalangeal joint with the whole metatarsal bone for the treatment of a radial club hand. To date, there are no clinical reports of an allogenic transfer of growth plates. However, the results of this study might provide useful information that may make this procedure feasible in the near future. Further studies will be needed to assess the various biochemical, hormonal and other factors responsible for the differences in the bone densities of the transplanted bones in different age groups.
In conclusion, age is one of the significant factors that affect the bone quality after bone transplantation. However, after transplantation, the growth plate remains unaffected by the difference in age and continues to grow at its own inherent rate.
Figures and Tables
Fig. 1
Radiogram of the rat hindlimbs taken 3 weeks after transplantation-tibial lengths of both the transplanted and opposite non operated limb were measured.
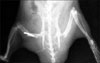
Fig. 2
Radiograph of rat hindlimbs 10 weeks after transplantation showing an increase in the length of the tibias of the transplanted limbs as well as the opposite non operated limbs.
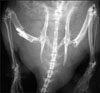
Table 1
The Micro-CT 3D Parameters of the Transplanted Bone in the Isochronograft and Heterochronograft Limbs
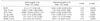
References
2. Burchardt H, Enneking WF. Transplantion of bone. Surg Clin North Am. 1978. 58:403–427.
3. Kline SC, Hotchkiss RN, Randolph MA, Weiland AJ. Study of growth kinetics and morphology in limbs transplanted between animals of different ages. Plast Reconstr Surg. 1990. 85:273–280.


4. Steinberg EL, Luger E, Zwas T, Katznelson A. Very long-term radiographic and bone scan results of frozen autograft and allograft bone grafting in 17 patients (20 grafts) a 30- to 35-year follow-up. Cell Tissue Bank. 2004. 5:97–104.


5. Green H, Morikawa M, Nixon T. A dual effector theory of growth-hormone action. Differentiation. 1985. 29:195–198.


6. Kan KW, Cruess RL, Posner BI, Guyda HJ, Solomon S. Hormone receptors in the epiphysial cartilage. J Endocrinol. 1984. 103:125–131.


7. Nilsson A, Isgaard J, Lindahl A, Dahlstrom A, Skttner A, Isaksson OG. Regulation by growth hormone of number of chondrocytes containing IGF-I in rat growth plate. Science. 1986. 233:571–574.


8. Ogden JA, Southwick WO. Endocrine dysfunction and slipped capital femoral epiphysis. Yale J Biol Med. 1977. 50:1–16.
9. Trippel SB, Van Wyk JJ, Mankin HJ. Localization of somatomedin-C binding to bovine growth-plate chondrocytes in situ. J Bone Joint Surg Am. 1986. 68:897–903.


10. Chiu HY, Harii K. Morphologic and growth alterations of epiphyseal plate after isohistogeneic transfer of young rat limb to adult rat. J Reconstr Microsurg. 1988. 4:103–111.


11. Drzewiecki AE, Randolph MA, Hotchkiss RH, Weiland AJ. Vascularized growth-plate transplantation: a comparative study in the rat. J Reconstr Microsurg. 1992. 8:93–100.


12. Innocenti M, Delcroix L, Manfrini M, Ceruso M, Capanna R. Vascularized proximal fibular transfer for distal radial reconstruction. J Bone Joint Surg Am. 2005. 87:Suppl 1. (Pt 2):237–246.
13. Innocenti M, Delcroix L, Romano GF. Epiphyseal transplant: harvesting technique of the proximal fibula based on the anterior tibial artery. Microsurgery. 2005. 25:284–292.


14. Innocenti M, Delcroix L, Manfrini M, Ceruso M, Capanna R. Vascularized proximal fibular epiphyseal transfer for distal radial reconstruction. J Bone Joint Surg Am. 2004. 86:1504–1511.


15. Innocenti M, Ceruso M, Manfrini M, et al. Free vascularized growth-plate transfer after bone tumor resection in children. J Reconstr Microsurg. 1998. 14:137–143.


16. Vilkki SK. Distraction and micro vascular epiphysis transfer for radial club hand. J Hand Surg. 1995. 31:1.
17. Donaldson HH. The rat: data and reference tables. 1992. 2nd ed. Philadelphia: