Abstract
Background
Single-nucleotide polymorphism (SNP) analysis is a powerful strategy for large-scale molecular population studies examining phylogenetic relationships among bacterial strains. Mycobacterial interspersed repetitive units-variable number of tandem repeats (MIRU-VNTR) can be easily digitized to share data among laboratories. This study applied SNP and MIRU-VNTR analyses for molecular strain typing of Mycobacterium tuberculosis isolates collected throughout Korea.
Methods
We studied 102 clinical M. tuberculosis isolates, including 6 paired strains, collected from 11 university hospitals in Korea in 2008 and 2009. SNPs were detected using hairpin primer assays, and then, MIRU-VNTR analysis was performed.
Results
Thirty-five SNPs contained polymorphisms that helped differentiate the 96 tested isolates. The isolates were classified into 15 clusters. The Beijing family strains were distributed within closely related clusters in the SNP dendrogram. For MIRU-VNTR analysis, the 96 isolates were divided into 12 groups. The discriminatory index in 8 of these groups (MIRU-10, -23, -26, and -31; ETR-A, -B, -C, and -F) was high (Hunter-Gaston diversity index > 0.6). Unlike the SNP method, MIRU-VNTR analysis did not identify any notable localizations of Beijing or non-Beijing family isolates in specific clusters.
Molecular typing methods have been extensively applied to understand the genetic variations in and phylogenetic relationships among various Mycobacterium tuberculosis strains, particularly the strains responsible for large-scale infection outbreaks and the drug-resistant strains, and to differentiate re-infection from relapse [1-3].
Detection of the IS6110-restriction fragment length polymorphism (RFLP) is considered the gold standard for genotyping M. tuberculosis, because this RFLP has high discriminatory power [4]. However, RFLP has some drawbacks; a long culture time is required to obtain enough DNA, and it is time- and labor-intensive [5]. Unlike the IS6110 genotyping method, spoligotyping, which is based on polymorphisms of direct repeat loci, is simple and rapid because it is PCR-based [6, 7]. One limitation of this method is its inability to discriminate Beijing family strains. This renders the method unsuitable for strain typing as an epidemiologic tool in Korea where the Beijing family strains are predominant [8, 9].
Recently, genome sequence data of 4 M. tuberculosis complex strains have become available, and this has enabled the development of a molecular method using single-nucleotide polymorphisms (SNPs) to identify M. tuberculosis strains [10, 11]. These SNPs can be used as a precise tool in phylogenetic studies [12-14]. SNP analysis also provides a powerful strategy for large-scale molecular population studies examining phylogenetic relationships among bacterial strains [15]. However, there is no standard SNP analysis method for estimating genetic relationships between strains isolated during large-scale outbreaks of infection or drug-resistant strains.
Mycobacterial interspersed repetitive units-variable number of tandem repeats (MIRU-VNTR), another strain-typing method, has been developed in the past decade [2]. This method is considerably faster, requires only small amounts of DNA, and can be easily digitized to share data among laboratories [5, 16]. Thus, SNP and MIRU-VNTR analyses are good alternative methods for M. tuberculosis molecular strain typing.
This study applied SNP and MIRU-VNTR analyses to M. tuberculosis isolates collected throughout Korea.
We studied 102 clinical M. tuberculosis isolates collected from 11 university hospitals in Korea in 2008 and 2009. Among the isolated strains, 96 were collected from single cultures performed in 96 patients, and 6 were collected from different cultures performed in the same patients. Epidemiologic distribution of the 96 isolates had already been characterized by IS6110-RFLP, and all isolates were collected from epidemiologically unrelated patients [17]. The 6 paired strains were used to evaluate the reproducibility of the strain-typing methods used in this study.
We selected 45 SNPs previously demonstrated to provide high resolution between clinical isolates [18]. Positions of each of the 45 SNPs and its nucleotides in M. tuberculosis H37Rv are presented in Table 1. SNPs were detected using hairpin primer (HP) assays as described previously [19]. DNA including a target nucleotide in each primer was amplified using the HP assay. A wild-type HP primer and different mutant HP primer were used in a complementary fashion. Primer sequences are published in a previous paper and are not repeated here [19]. In brief, the amplification protocol was as follows: stage 1, 95℃ for 10 min, 70℃ for 30 sec; stage 2, 72℃ for 30 sec, 95℃ for 20 sec, 69℃ for 30 sec, lowering 1° in the last step for every cycle during 10 cycles; and stage 3, 72℃ for 30 sec, 95℃ for 20 sec, and 60℃ for 30 sec; this was repeated 40 times. PCR products were analyzed on a 2% agarose gel.
MIRU-VNTR was performed as described previously [20, 21]. Briefly, isolates were genotyped by PCR amplification of the 12 MIRU-VNTR loci (MIRU-02, -04, -10, -16, -20,-23, -24, -26, -27, -31, -39, and -40) and 4 exact tandem repeat (ETR) loci (ETR-A, -B, -C, -F). The amplification protocol consisted of 30 cycles of 30 sec at 95℃, 30 sec at 61℃, and 1 min at 72℃. PCR products were analyzed on a 2% agarose gel, and the number of tandem repeats was calculated.
Phylogenetic analysis was performed using the complete data of 96 isolates for SNP analysis at 45 loci and for MIRU-VNTR analysis at 16 loci. A dendrogram of SNP and MIRU-VNTR analyses was constructed from the UPGMA cluster based on the overall similarity of each nucleotide polymorphism (http://genomes.urv.cat/UPGMA/). For discrimination analysis of MIRU-VNTR, the Hunter-Gaston diversity index (HGDI) was calculated as described elsewhere [22] and used for comparison of the discriminatory power of each VNTR locus.
Among the 45 SNPs, 35 had polymorphisms that could be used to differentiate the 96 tested isolates. The other 10 SNPs did not contain polymorphisms. Based on SNP results, isolates were classified into 15 clusters (Fig. 1). Cluster 1, the most populated cluster, contained 19 isolates, followed by cluster 4 with 17 isolates. Three clusters consisted of only 1 isolate each (Table 2). As shown in Fig. 1, nearly all Beijing family strains, which are defined and identified by IS6110 RFLP data [17], were distributed within closely related clusters in the SNP dendrogram. Seventy-four of the 76 Beijing family strains were allocated to SNP clusters 1 through 12. The remaining 2 isolates were allocated to clusters 13 and 14. Nearly all 14 isolates of the K family, a subfamily of the Beijing family identified in a previous study [17], were allocated to clusters 4 and 6.
The 96 isolates showed amplification products from 16 VNTR loci and contained at least 1 copy of each locus. Results of allelic diversity and HGDI testing are summarized in Table 3. Among the 16 loci, the discriminatory index in 8 (MIRU-10, -23, -26 and -31; ETR-A, -B, -C, and -F) was high (HGDI > 0.6) according to the definition of Sola et al. [23]. Six loci (MIRU-4, -16, -24, -27, -39 and -40) were dispersed and discriminated moderately well (0.3 ≤ HGDI ≤ 0.6). Two loci (MIRU-2 and -20) were poorly discriminated (HGDI < 0.3). The complete data were divided into 12 groups. The largest group was group 2, which included 15 isolates. Group 11 contained the only unique pattern (Fig. 2). Unlike the SNP method, MIRU-VNTR did not show notable localizations of Beijing or non-Beijing family isolates in specific clusters.
SNP analysis was developed after whole-genome sequence data became available and has been for genome-scale analysis to estimate overall chromosomal relationships among isolates [24, 25]. SNP analysis is a powerful strategy for examining phylogenetic relationships among bacterial strains and is easy to perform [26, 27]. Among various methodologies described previously, we chose the HP assay for this study to improve PCR specificity by decreasing nucleotide mismatching [19, 28]. Additionally, the material costs are lower for this method than for a sequencing-based method [19]. Previous studies have demonstrated that all tested M. tuberculosis strains were distinguishable by SNP analysis, and therefore, the authors proposed that SNP analysis was an ideal tool to investigate biological variations among clinical M. tuberculosis isolates [15, 18]. Filliol et al. [18] identified a minimal number of 45 highly informative SNPs by analyzing 212 SNPs against 323 clinical M. tuberculosis and Mycobacterium bovis isolates. Our SNP analysis was based on this set of 45 SNPs. In this study, however, only 35 SNP loci could be used to categorize our 96 M. tuberculosis isolates. Interestingly, nearly all Beijing family strains, including those of the K family, were allocated to closely related clusters. Our results indicate that SNP analysis using 35 loci is useful for phylogenetic analysis of clinical M. tuberculosis isolates from Korea. This method is effective, easy, and economical. In the 96 M. tuberculosis isolates examined, 10 of 45 SNPs were identical. We think that this difference was due to the difference in strain distribution between the current and previous studies [18]. Our study included a larger proportion of Beijing family isolates, whereas only 15 Beijing family isolates, among the 246 tested isolates, were included in the previous study [18]; moreover, those 15 isolates were classified as a single SNP group. Therefore, some SNPs proven to be polymorphic between strains isolated in 1 region may not be suitable to discriminate strains originating in other regions.
The MIRU-VNTR typing method is technically more flexible and faster than IS6110-RFLP typing, and the results are expressed as numerical codes, which are easy to compare and exchange [29, 30]. A previous report demonstrated that MIRU-VNTR analysis using well-selected loci is useful for analyzing clinical M. tuberculosis isolates from Korea, where the Beijing family is predominant [31]. In the current MIRU-VNTR study using 96 isolates, we identified 2 interesting findings. First, in contrast to SNP results, MIRU-VNTR patterns were not related to IS6110-RFLP patterns. As shown in Fig. 2, isolates of Beijing and non-Beijing families were distributed in almost all clusters. This means that changes in MIRU-VNTR loci developed independently of changes in IS6110 insertion. Hanekom et al. [32] described discordant results between MIRU-VNTR and IS6110 RFLP and suggested that the degree of discordance was dependent on the genetic distance between isolates. However, SNP analysis resulted in clusters closely related to IS6110-RFLP patterns. Filliol et al. [18] described similar close clustering of Beijing family strains by both the SNP and spoligotyping methods. We could not locate M. tuberculosis molecular strain-typing data that had been obtained by simultaneously using IS6110-RFLP, SNPs, and MIRU-VNTR. Here, we describe a close relationship between SNP and IS6110-RFLP and independent results for MIRU-VNTR and IS6110-RFLP. This means that, unlike IS6110-RFLP, spoligotyping, or SNP, MIRU-VNTR shows high discriminatory power for typing M. tuberculosis strains in areas where the Beijing family is highly prevalent. Second, the discriminatory power of MIRU-VNTR in some loci varied from that described in a previous study conducted with 81 isolates from Korea [31]. In that study, Yun et al. [31] reported that MIRU-23 and ETR-A, -B, and -C had low discriminatory power (HGDI < 0.3). In our study, however, these 4 loci were highly discriminatory (HGDI > 0.6), with MIRU-23 having the second highest discriminatory power (HGDI = 0.75). The highest discriminatory power was shown by MIRU-26 in both studies (HGDI > 0.80). The discriminatory power of a specific locus is inevitably affected by the selection of isolates, and the selection criteria were different between the 2 studies. In the previous study, all isolates were collected from Pusan National University Hospital or provided by the Korean Institute of Tuberculosis, so the distribution of patients' place of residences might have been restricted [31]. In this study, all 96 isolates were collected from 11 university hospitals located in various areas, and the number of isolates from each hospital was roughly proportional to the recorded patient numbers in the area served by that institution. Therefore, our study represents MIRU-VNTR performance more accurately. Combining the results of both the studies, we found that MIRU-10, -26, -31, and ETR-F show a high discriminatory power for molecular strain typing of M. tuberculosis isolates from Korea. Our assessment of the clinical utility of other loci, such as ETR-A, -B, and -C, that showed good discriminatory power in only 1 study is reserved until further data are obtained.
We confirmed the reproducibility of SNP and MIRU-VNTR analyses using 6 pairs of strains from different cultures of the same patients. Both SNP and MIRU-VNTR can discriminate clinical M. tuberculosis isolates in Korea, where Beijing family strains are highly prevalent. Both methods are PCR-based and early application to clinical specimens is possible. Strain typing is often requested to detect outbreaks or contamination, and rapid results are required to control these phenomena. Although IS6110-RFLP has high discriminatory power, it is not useful for these purposes. Therefore, SNP and MIRU-VNTR can be used as surrogate molecular strain-typing methods for M. tuberculosis in Korea.
Acknowledgement
This work was supported by the Korea Research Foundation Grant funded by the Korean Government (Ministry of Education and Human Resources Development, Basic Research Promotion Fund) (KRF-2008-1-E00318).
References
1. Gopaul KK, Brown TJ, Gibson AL, Yates MD, Drobniewski FA. Progression toward an improved DNA amplification-based typing technique in the study of Mycobacterium tuberculosis epidemiology. J Clin Microbiol. 2006; 44:2492–2498. PMID: 16825370.
2. Van Soolingen D. Molecular epidemiology of tuberculosis and other mycobacterial infections: main methodologies and achievements. J Intern Med. 2001; 249:1–26. PMID: 11168781.


3. Seidler A, Nienhaus A, Diel R. The transmission of tuberculosis in the light of new molecular biological approaches. Occup Environ Med. 2004; 61:96–102. PMID: 14739374.


4. van Embden JD, Cave MD, Crawford JT, Dale JW, Eisenach KD, Gicquel B, et al. Strain identification of Mycobacterium tuberculosis by DNA fingerprinting: recommendations for a standardized methodology. J Clin Microbiol. 1993; 31:406–409. PMID: 8381814.
5. Barlow RE, Gascoyne-Binzi DM, Gillespie SH, Dickens A, Qamer S, Hawkey PM. Comparison of variable number tandem repeat and IS6110-restriction fragment length polymorphism analyses for discrimination of high- and low-copy-number IS6110 Mycobacterium tuberculosis isolates. J Clin Microbiol. 2001; 39:2453–2457. PMID: 11427553.
6. Gori A, Bandera A, Marchetti G, Degli Esposti A, Catozzi L, Nardi GP, et al. Spoligotyping and Mycobacterium tuberculosis. Emerg Infect Dis. 2005; 11:1242–1248. PMID: 16102314.
7. Gori A, Esposti AD, Bandera A, Mezzetti M, Sola C, Marchetti G, et al. Comparison between spoligotyping and IS6110 restriction fragment length polymorphisms in molecular genotyping analysis of Mycobacterium tuberculosis strains. Mol Cell Probes. 2005; 19:236–244. PMID: 16038791.
8. Kamerbeek J, Schouls L, Kolk A, van Agterveld M, van Soolingen D, Kuijper S, et al. Simultaneous detection and strain differentiation of Mycobacterium tuberculosis for diagnosis and epidemiology. J Clin Microbiol. 1997; 35:907–914. PMID: 9157152.
9. Kremer K, van Soolingen D, Frothingham R, Haas WH, Hermans PW, Martin C, et al. Comparison of methods based on different molecular epidemiological markers for typing of Mycobacterium tuberculosis complex strains: interlaboratory study of discriminatory power and reproducibility. J Clin Microbiol. 1999; 37:2607–2618. PMID: 10405410.
10. Cole ST, Brosch R, Parkhill J, Garnier T, Churcher C, Harris D, et al. Deciphering the biology of Mycobacterium tuberculosis from the complete genome sequence. Nature. 1998; 393:537–544. PMID: 9634230.
11. Fleischmann RD, Alland D, Eisen JA, Carpenter L, White O, Peterson J, et al. Whole-genome comparison of Mycobacterium tuberculosis clinical and laboratory strains. J Bacteriol. 2002; 184:5479–5490. PMID: 12218036.
12. Kersulyte D, Mukhopadhyay AK, Velapatino B, Su W, Pan Z, Garcia C, et al. Differences in genotypes of Helicobacter pylori from different human populations. J Bacteriol. 2000; 182:3210–3218. PMID: 10809702.
13. Schork NJ, Fallin D, Lanchbury JS. Single nucleotide polymorphisms and the future of genetic epidemiology. Clin Genet. 2000; 58:250–264. PMID: 11076050.


14. Gutacker MM, Smoot JC, Migliaccio CA, Ricklefs SM, Hua S, Cousins DV, et al. Genome-wide analysis of synonymous single nucleotide polymorphisms in Mycobacterium tuberculosis complex organisms: resolution of genetic relationships among closely related microbial strains. Genetics. 2002; 162:1533–1543. PMID: 12524330.
15. Gutacker MM, Mathema B, Soini H, Shashkina E, Kreiswirth BN, Graviss EA, et al. Single-nucleotide polymorphism-based population genetic analysis of Mycobacterium tuberculosis strains from 4 geographic sites. J Infect Dis. 2006; 193:121–128. PMID: 16323140.
16. Frothingham R, Meeker-O'Connell WA. Genetic diversity in the Mycobacterium tuberculosis complex based on variable numbers of tandem DNA repeats. Microbiology. 1998; 144(Pt 5):1189–1196. PMID: 9611793.
17. Choi GE, Jang MH, Song EJ, Jeong SH, Kim JS, Lee WG, et al. IS6110-restriction fragment length polymorphism and spoligotyping analysis of Mycobacterium tuberculosis clinical isolates for investigating epidemiologic distribution in Korea. J Korean Med Sci. 2010; 25:1716–1721. PMID: 21165284.
18. Filliol I, Motiwala AS, Cavatore M, Qi W, Hazbón MH, Bobadilla del Valle M, et al. Global phylogeny of Mycobacterium tuberculosis based on single nucleotide polymorphism (SNP) analysis: insights into tuberculosis evolution, phylogenetic accuracy of other DNA fingerprinting systems, and recommendations for a minimal standard SNP set. J Bacteriol. 2006; 188:759–772. PMID: 16385065.
19. Hazbón MH, Alland D. Hairpin primers for simplified single-nucleotide polymorphism analysis of Mycobacterium tuberculosis and other organisms. J Clin Microbiol. 2004; 42:1236–1242. PMID: 15004082.
20. Supply P, Allix C, Lesjean S, Cardoso-Oelemann M, Rüsch-Gerdes S, Willery E, et al. Proposal for standardization of optimized mycobacterial interspersed repetitive unit-variable-number tandem repeat typing of Mycobacterium tuberculosis. J Clin Microbiol. 2006; 44:4498–4510. PMID: 17005759.
21. Supply P, Mazars E, Lesjean S, Vincent V, Gicquel B, Locht C. Variable human minisatellite-like regions in the Mycobacterium tuberculosis genome. Mol Microbiol. 2000; 36:762–771. PMID: 10844663.
22. Hunter PR, Gaston MA. Numerical index of the discriminatory ability of typing systems: an application of Simpson's index of diversity. J Clin Microbiol. 1988; 26:2465–2466. PMID: 3069867.


23. Sola C, Filliol I, Legrand E, Lesjean S, Locht C, Supply P, et al. Genotyping of the Mycobacterium tuberculosis complex using MIRUs: association with VNTR and spoligotyping for molecular epidemiology and evolutionary genetics. Infect Genet Evol. 2003; 3:125–133. PMID: 12809807.
24. Hirsh AE, Tsolaki AG, DeRiemer K, Feldman MW, Small PM. Stable association between strains of Mycobacterium tuberculosis and their human host populations. Proc Natl Acad Sci U S A. 2004; 101:4871–4876. PMID: 15041743.
25. Brosch R, Gordon SV, Marmiesse M, Brodin P, Buchrieser C, Eiglmeier K, et al. A new evolutionary scenario for the Mycobacterium tuberculosis complex. Proc Natl Acad Sci U S A. 2002; 99:3684–3689. PMID: 11891304.
26. Kaboev OK, Luchkina LA, Tret'iakov AN, Bahrmand AR. PCR hot start using primers with the structure of molecular beacons (hairpin-like structure). Nucleic Acids Res. 2000; 28:E94. PMID: 11058144.


27. Nazarenko I, Lowe B, Darfler M, Ikonomi P, Schuster D, Rashtchian A. Multiplex quantitative PCR using self-quenched primers labeled with a single fluorophore. Nucleic Acids Res. 2002; 30:e37. PMID: 11972352.


28. Bouakaze C, Keyser C, de Martino SJ, Sougakoff W, Veziris N, Dabernat H, et al. Identification and genotyping of Mycobacterium tuberculosis complex species by use of a SNaPshot Minisequencing-based assay. J Clin Microbiol. 2010; 48:1758–1766. PMID: 20220173.
29. Supply P, Lesjean S, Savine E, Kremer K, van Soolingen D, Locht C. Automated high-throughput genotyping for study of global epidemiology of Mycobacterium tuberculosis based on mycobacterial interspersed repetitive units. J Clin Microbiol. 2001; 39:3563–3571. PMID: 11574573.
30. Kwara A, Schiro R, Cowan LS, Hyslop NE, Wiser MF, Roahen Harrison S, et al. Evaluation of the epidemiologic utility of secondary typing methods for differentiation of Mycobacterium tuberculosis isolates. J Clin Microbiol. 2003; 41:2683–2685. PMID: 12791904.
31. Yun KW, Song EJ, Choi GE, Hwang IK, Lee EY, Chang CL. Strain typing of Mycobacterium tuberculosis isolates from Korea by mycobacterial interspersed repetitive units-variable number of tandem repeats. Korean J Lab Med. 2009; 29:314–319. PMID: 19726893.
32. Hanekom M, van der Spuy GD, Gey van Pittius NC, McEvoy CR, Hoek KG, Ndabambi SL, et al. Discordance between mycobacterial interspersed repetitive-unit-variable-number tandem-repeat typing and IS6110 restriction fragment length polymorphism genotyping for analysis of Mycobacterium tuberculosis Beijing strains in a setting of high incidence of tuberculosis. J Clin Microbiol. 2008; 46:3338–3345. PMID: 18716230.
Fig. 1
Distance-based neighbor joining phylogenetic tree of 96 Mycobacterium tuberculosis isolates based on 35 single-nucleotide polymorphisms reveals 15 clusters (indicated by dotted circles). Each IS6110-restriction fragment length polymorphism type is indicated by a closed circle, quadrangle, or arrow.
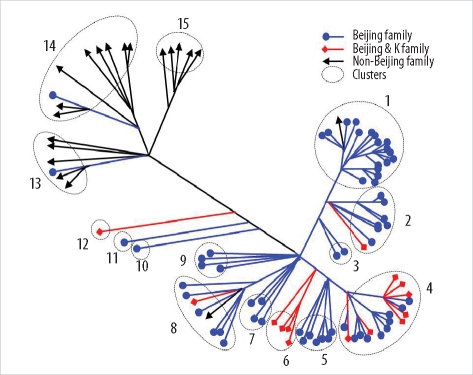
Fig. 2
Distance-based neighbor joining phylogenetic tree of 96 Mycobacterium tuberculosis isolates based on based on mycobacterial interspersed repetitive units-variable number of tandem repeats of 16 loci reveals 12 clusters (dotted circles). Each IS6110-restriction fragment length polymorphism type is indicated by a closed circle, quadrangle, or arrow.
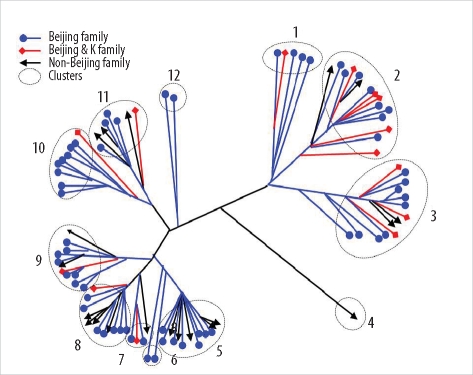
Table 1
Position of 45 single-nucleotide polymorphisms and identification of their nucleotides in Mycobacterium tuberculosis H37Rv
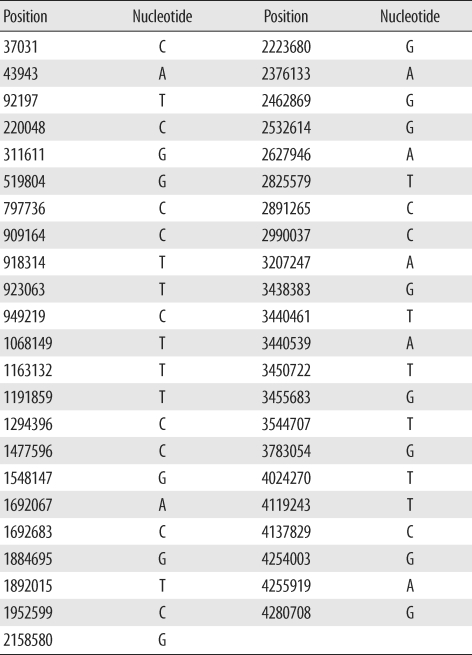
Table 2
SNP clusters of 102 clinical isolates of Mycobacterium tuberculosis collected throughout Korea
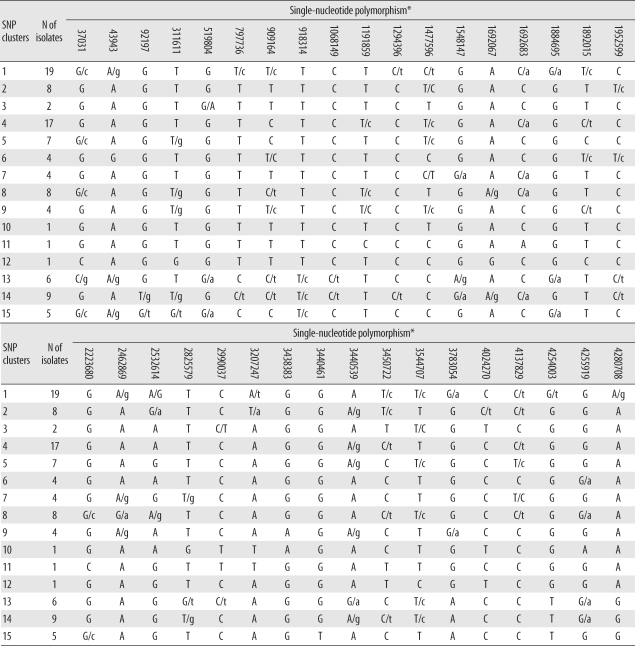
Table 3
Variable repeat numbers in each VNTR locus and allelic diversity among 96 clinical isolates of Mycobacterium tuberculosis collected throughout Korea
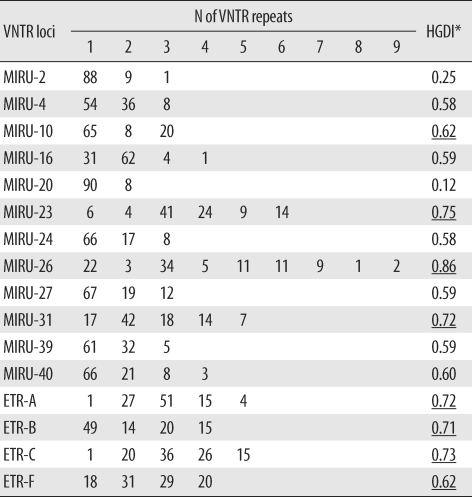
*Underlining indicates loci showing high discriminatory power, as defined by Sola et al. [23].
Abbreviations: VNTR, variable number of tandem repeats; HGDI, Hunter-Gaston diversity index; MIRU, mycobacterial interspersed repetitive units; ETR, exact tandem repeat.