Abstract
Background
Prevention and control of thalassemia requires simple, rapid, and accurate screening tests for carrier couples who are at risk of conceiving fetuses with severe thalassemia.
Methods
Single-tube multiplex real-time PCR with SYBR Green1 and high-resolution melting (HRM) analysis were used for the identification of α-thalassemia-1 Southeast Asian (SEA) and Thai type deletions and β-thalassemia 3.5-kb gene deletion. The results were compared with those obtained using conventional gap-PCR. DNA samples were derived from 28 normal individuals, 11 individuals with α-thalassemia-1 SEA type deletion, 2 with α-thalassemia-1 Thai type deletion, and 2 with heterozygous β-thalassemia 3.5-kb gene deletion.
Results
HRM analysis indicated that the amplified fragments from α-thalassemia-1 SEA type deletion, α-thalassemia-1 Thai type deletion, β-thalassemia 3.5-kb gene deletion, and the wild-type β-globin gene had specific peak heights at mean melting temperature (Tm) values of 86.89℃, 85.66℃, 77.24℃, and 74.92℃, respectively. The results obtained using single-tube multiplex real-time PCR with SYBR Green1 and HRM analysis showed 100% consistency with those obtained using conventional gap-PCR.
Thalassemia, a group of inherited blood disorders, can be classified as α- and β-thalassemias on the basis of its clinical manifestations and genetic background. More than 95% of documented cases of α-thalassemia reported the deletion of one or both α-globin genes from chromosome 16p13.3 [1, 2]. These gene deletions cause mild α-thalassemia-2 and severe α-thalassemia-1. The most common mutations of α-thalassemia-1 in the Thai population are the Southeast Asian (SEA) and Thai type deletions; the SEA deletion is approximately 20.5 kb in length, involving both functional α-globin genes but leaving the ζ2-globin gene intact [3, 4], and the Thai type deletion, is approximately 33.45 kb in length, involving both α-globin genes as well as the ζ2 gene [5]. In Southeast Asia, the carrier frequencies of α-thalassemia-1 SEA and Thai type deletions were 4-14% and 0.2%, respectively, depending on the population [4, 6, 7].
Unlike α-thalassemia, in which large deletion mutations predominate, β-thalassemia usually occurs because of mutations other than deletion mutations. However, some cases of β-thalassemia are due to large deletions, which vary from 0.1 to 10 kb [8, 9]. The most frequent β-globin gene deletion found in the Thai population is a 3.5-kb deletion located at the 5' breakpoint in the region between nucleotides -810 and -128 of the β-globin locus and the 3' breakpoint between the Ava II and Xmn I sites located downstream of the β-globin gene [10]; this 3.5-kb deletion has a prevalence of 2% [11-13]. In addition, double heterozygosity for α- and β-thalassemias can also be found in the Thai population with a frequency of approximately 2% [14].
Even though carriers of α-thalassemia-1 do not show any clinical symptoms, couples in which both individuals are carriers have a 25% chance of conceiving a fetus that is homozygous for the deletion, which manifests as Bart's hydrops fetalis, the most severe thalassemic syndrome. All these fetuses either die in utero or soon after birth [15-17]. In addition, the homozygosity or compound heterozygosity for β-thalassemia mutations produce severe clinical phenotypes, and the child is mostly blood transfusion-dependent. Moreover, the compound β-thalassemia/Hb-E causes anemia, which varies from almost asymptomatic states to severe anemia and transfusion dependency in patients [18-20]. An examination for α-thalassemia-1 and β-thalassemia is, therefore, essential for carrier couples who are at risk of conceiving a fetus with severe thalassemias (Bart's hydrops fetalis, β-thalassemia, and β-thalassemia/Hb-E).
Real-time PCR with SYBR Green1 and high resolution melting (HRM) analysis are currently used to diagnose α-thalassemia-1 SEA type deletion and β-thalassemia 3.5-kb gene deletion [12, 21]. Furthermore, HRM analysis and multiplex PCR with SYTO-9 is used for prenatal diagnosis of β-thalassemia/Hb-E [22], and multiplex PCR with SYBR Green1 followed by melting curve analysis is used for the identification of α-thalassemia-1 SEA and Thai type deletions [23]. HRM analysis is simple, high-throughput, and is faster than the conventional PCR since it does not require the post-PCR processing steps [12, 21, 22]. This approach would be more cost-effective if it could be processed as a multiplex PCR for diagnosis of several types of thalassemias. Therefore, the aim of this study was to develop a system of single-tube multiplex real-time PCR using SYBR Green1 and HRM analysis for rapid diagnosis of α-thalassemia-1 SEA and Thai type deletions and β-thalassemia 3.5-kb gene deletion, the most frequent thalassemia large gene deletions found in Thai and Southeast Asian populations.
DNA was extracted from EDTA-anticoagulated blood samples of 28 normal individuals, 11 individuals with α-thalassemia-1 SEA type deletion, 2 with α-thalassemia-1 Thai type deletion, and 2 with β-thalassemia 3.5-kb gene deletion by using the NucleoSpin® kit (Macherey-Nagel; KG., Duren, Germany), according to the manufacturer's instructions. The thalassemic genotypes of the DNA samples were determined by conventional gap-PCR [24, 25] following the thalassemia screening protocol before using single-tube multiplex real-time PCR. This study was approved by the Ethics Committee of the Faculty of Associated Medical Sciences, Chiang-Mai University.
DNA amplification was carried out in a 25 µL reaction mixture containing 12.5 µL 2× Fast SYBR® Green Master Mix (QIAGEN; GmbH, Hilden, Germany); primer sets specific for α-thalassemia-1 SEA and Thai type deletions, β-thalassemia 3.5-kb deletion and wild-type β-globin gene, which was used as the internal control; and 7 µL DNA sample. Nucleotide sequences and final concentrations of the primers used in this study are shown in Table 1. The primer sequences used in this study for α-thalassemia-1 SEA type deletion, β-thalassemia 3.5-kb deletion, and wild-type β-globin gene were the same as those used in previous studies [12, 21]. PCR with SYBR Green1 was performed using Rotor-Gene 6000™ (Corbett Research; Mortlake, New South Wales, Australia). The mixture was preheated at 95℃ for 5 min, followed by 40 cycles at 95℃ for 10 sec and 60℃ for 30 sec. The amplification cycles were followed by a high-resolution melting cycle from 70℃ to 95℃ at the rate of 0.1℃/2 sec. When the melting temperature (Tm) is reached, double-stranded DNA is denatured and SYBR Green1 is released, which causes a dramatic decrease in fluorescence intensity. The rate of this change was determined by plotting the derivative of the fluorescence relative to that of the temperature (dF/dT) by using the data analysis software of the real-time PCR instrument. The temperature at which a peak occurs on the plot corresponds to the Tm of the DNA duplex. The Tm values of α-thalassemia-1 SEA type deletion, β-thalassemia 3.5-kb deletion, and wild-type β-globin gene that were obtained in this study were similar to those determined by singleplex PCR in previous studies [12, 21]. The Tm of α-thalassemia-1 Thai type deletion was determined using singleplex PCR prior to the multiplex analysis performed using newly designed primers.
Representative results of the dissociation curve analysis of each type of thalassemia assessed are shown in Fig. 1. The amplified fragments from the α-thalassemia-1 SEA and Thai type deletions had specific peak heights at mean Tm values of 86.89±0.17℃ and 85.66±0.08℃, respectively. The amplified fragment from β-thalassemia 3.5-kb gene deletion had specific peak height at a mean Tm of 77.24±0.14℃. Moreover, the specific peak height of the internal control fragment from the wild-type β-globin gene was obtained at a mean Tm of 74.92±0.26℃. The results interpreted using single-tube multiplex real-time PCR with SYBR Green1 and HRM analysis were completely consistent with those derived using conventional gap-PCR.
Development of strategies for the prevention and control of thalassemia requires the identification of individuals at a risk of having offspring with severe thalassemias, including Bart's hydrops fetalis, homozygous β-thalassemia, and β-thalassemia/Hb-E [26, 27]. Real-time PCR with SYBR Green1 and HRM analysis are currently used for the identification of thalassemia mutations [12, 21-23, 28]. This technique does not require fluorescently labeled probes or separation steps. Therefore, it offers additional advantages, including minimal labor, rapid turnaround time, and decreased risk of carryover contamination. Usually, SYBR Green1 is incorporated in the commercial master mix, and this is not cost effective, especially for single gene analysis.
To reduce the cost and complexity for screening thalassemia large gene deletions, which are the causes of severe anemia, we have developed a system of single-tube multiplex real-time PCR with SYBR Green1 and HRM analysis for identification of α-thalassemia-1 SEA and Thai type deletions and β-thalassemia 3.5-kb gene deletion. By this approach, a specific peak height was observed for each type of thalassemia assessed. To increase the sensitivity of the analysis, primers that specifically detected α-thalassemia-1 deletions were used at a higher concentration than primers for detection of the wild-type β-globin gene, which was used as an internal control. As a consequence, the specific peak heights for α-thalassemia-1 SEA and Thai type deletions were more noticeable than those for the internal control gene. Further, when the analyzed α- and β-thalassemic genes were not present in the samples, the peak height of the internal control was still clearly observed. Even though this technique cannot be used for identifying homozygous α- and β-thalassemia, it can facilitate rapid screening of their heterozygosity. Considering the high prevalence of homozygosity of α-thalassemia-1 (Hb Bart's hydrops fetalis) and β-thalassemia diseases in fetuses produced by carrier couples (8% and 1%, respectively [29]), the homozygosity of the positive samples analyzed by single-tube multiplex real-time PCR with SYBR Green1 and HRM analysis should be further analyzed by conventional gap-PCR or real-time gap-PCR with HRM analysis [12, 21, 24, 30].
It has been previously suggested that SYBR Green1 is not suitable for real-time PCR with HRM analysis since high concentrations of SYBR Green1 (10 µM) could inhibit PCR amplification [31]; it can only be used at a non-saturated concentration. Moreover, SYBR Green1 that dissociates from DNA during melting can re-intercalate into regions of unmelted double-stranded DNA [32]. This phenomenon of fluorescence dye redistribution can mask the small differences in the melting analysis stage [33, 34]. In the current study, SYBR Green1 is incorporated in the commercial master mix at an optimal concentration for multiplex PCR. Furthermore, the re-intercalation of SYBR Green1 did not occur and hence did not affect the melting curve analysis in our study. Additionally, the primer sets in this study were designed to amplify short lengths of PCR products (134 bp for α-thalassemia-1 SEA type deletions, 180 bp for Thai type deletions, 224 bp for β-thalassemia 3.5-kb gene deletions and 124 bp for wild-type β-globin genes). As a result, the re-intercalation of SYBR Green1 was scarce, providing sufficient discrimination between the Tm value peaks of each amplicon.
Although, the deletions of globin genes assessed in this study are most commonly found in only Thai and Southeast Asian populations, single-tube multiplex real-time PCR with SYBR Green1 and HRM analysis developed in this study may be used for diagnosis of other types of thalassemia gene deletions.
In conclusion, our results indicated that single-tube multiplex real-time PCR with SYBR Green1 and HRM analysis for screening α-thalassemia-1 SEA and Thai type deletions and β-thalassemia 3.5-kb gene deletion is as effective as using a conventional method. This method would significantly reduce the cost and complexity of screening α-thalassemia-1 SEA and Thai type deletions and β-thalassemia 3.5-kb gene deletions. Therefore, single-tube multiplex real-time PCR with SYBR Green1 and HRM analysis may be used as an alternative for routine clinical diagnosis of thalassemias and may be especially useful for genetic counseling and prevention and control programs of severe thalassemia in regions with a high prevalence of α- and β-thalassemia.
Acknowledgments
We thank the technicians in the Associated Medical Sciences Clinical Service Center, Associated Medical Sciences, Chiang-Mai University, Chiang-Mai, Thailand for their help and assistance.
This study was supported in part by grants from the National Research Council of Thailand.
References
1. Higgs DR, Vickers MA, Wilkie AO, Pretorius IM, Jarman AP, Weatherall DJ. A review of the molecular genetics of the human alpha-globin gene cluster. Blood. 1989; 73:1081–1104. PMID: 2649166.


2. Kazazian HH Jr. The thalassemia syndromes: molecular basis and prenatal diagnosis in 1990. Semin Hematol. 1990; 27:209–228. PMID: 2197725.
3. Nicholls RD, Fischel-Ghodsian N, Higgs DR. Recombination at the human α-globin gene cluster: sequence features and topological constraints. Cell. 1987; 49:369–378. PMID: 3032452.


4. Winichagoon P, Higgs DR, Goodbourn SE, Clegg JB, Weatherall DJ, Wasi P. The molecular basis of α-thalassaemia in Thailand. Embo J. 1984; 3:1813–1818. PMID: 6548185.
5. Eng B, Patterson M, Borys S, Chui DH, Waye JS. PCR-based diagnosis of the Filipino (--[FIL]) and Thai (--[THAI]) α-thalassemia-1 deletions. Am J Hematol. 2000; 63:54–56. PMID: 10602170.


6. Fischel-Ghodsian N, Vickers MA, Seip M, Winichagoon P, Higgs DR. Characterization of two deletions that remove the entire human zeta-alpha globin gene complex (- -THAI and - -FIL). Br J Haematol. 1988; 70:233–238. PMID: 3191033.
7. Fucharoen G, Fucharoen S, Wanhakit C, Srithong W. Molecular basis of alpha (0)-thalassemia in northeast of Thailand. Southeast Asian J Trop Med Public Health. 1995; 26(S1):S249–S251.
8. Craig JE, Kelly SJ, Barnetson R, Thein SL. Molecular characterization of a novel 10.3 kb deletion causing beta-thalassaemia with unusually high Hb A2. Br J Haematol. 1992; 82:735–744. PMID: 1482661.
9. Nopparatana C, Saechan V, Nopparatana C, Pornpatkul M, Panich V, Fukumaki Y. A novel 105 basepair deletion causing β0-thalas-semia in members of a Thai family. Am J Hematol. 1999; 61:1–4. PMID: 10331503.
10. Sanguansermsri T, Pape M, Laig M, Hundrieser J, Flatz G. Beta zero-thalassemia in a Thai family is caused by a 3.4 kb deletion including the entire beta-globin gene. Hemoglobin. 1990; 14:157–168. PMID: 2272839.
11. Nopparatana C, Panich V, Saechan V, Sriroongrueng V, Nopparatana C, Rungjeadpha J, et al. The spectrum of beta-thalassemia mutations in southern Thailand. Southeast Asian J Trop Med Public Health. 1995; 26:229–234. PMID: 8629112.
12. Prathomtanapong P, Pornprasert S, Phusua A, Suanta S, Saetung R, Sanguansermsri T. Detection and identification of β-thalassemia 3.5 kb deletion by SYBR Green1 and high resolution melting analysis. Eur J Haematol. 2009; 82:159–160. PMID: 19067740.
13. Winichagoon P, Fucharoen S, Wilairat P, Fukumaki Y. Molecular mechanisms of thalassemia in southeast Asia. Southeast Asian J Trop Med Public Health. 1995; 26(S1):235–240. PMID: 8629113.
14. Wanapirak C, Muninthorn W, Sanguansermsri T, Dhananjayanonda P, Tongsong T. Prevalence of thalassemia in pregnant wo-men at Maharaj Nakorn Chiang Mai Hospital. J Med Assoc Thai. 2004; 87:1415–1418. PMID: 15822533.
15. Ko TM, Hsieh FJ, Hsu PM, Lee TY. Molecular characterization of severe alpha-thalassemias causing hydrops fetalis in Taiwan. Am J Med Genet. 1991; 39:317–320. PMID: 1867284.
16. Liang ST, Wong VC, So WW, Ma HK, Chan V, Todd D. Homozygous alpha-thalassaemia: clinical presentation, diagnosis and ma-nagement. A review of 46 cases. Br J Obstet Gynaecol. 1985; 92:680–684. PMID: 4016025.


17. Thumasathit B, Nondasuta A, Silpisornkosol S, Lousuebsakul B, Unchalipongse P, Mangkornkanok M. Hydrops fetalis associated with Bart's hemoglobin in northern Thailand. J Pediatr. 1968; 73:132–138. PMID: 5658622.


18. Fucharoen S, Ketvichit P, Pootrakul P, Siritanaratkul N, Piankijagum A, Wasi P. Clinical manifestation of beta-thalassemia/hemoglobin E disease. J Pediatr Hematol Oncol. 2000; 22:552–557. PMID: 11132229.
19. Fucharoen S, Winichagoon P, Pootrakul P, Piankijagum A, Wasi P. Variable severity of Southeast Asian beta 0-thalassemia/Hb E disease. Birth Defects Orig Artic Ser. 1987; 23:241–248. PMID: 3689905.
20. Winichagoon P, Fucharoen S, Chen P, Wasi P. Genetic factors affecting clinical severity in beta-thalassemia syndromes. J Pediatr Hematol Oncol. 2000; 22:573–580. PMID: 11132233.
21. Pornprasert S, Phusua A, Suanta S, Saetung R, Sanguansermsri T. Detection of alpha-thalassemia-1 Southeast Asian type using real-time gap-PCR with SYBR Green1 and high resolution melting analysis. Eur J Haematol. 2008; 80:510–514. PMID: 18284625.


22. Pornprasert S, Sukunthamala K. SYTO9 and SYBR GREEN1 with a high resolution melting analysis for prenatal diagnosis of β0-thalassemia/hemoglobin-E. Eur J Haematol. 2010; 85:424–429. PMID: 20722700.
23. Munkongdee T, Vattanaviboon P, Thummarati P, Sewamart P, Winichagoon P, Fucharoen S, et al. Rapid diagnosis of alpha-thalassemia by melting curve analysis. J Mol Diagn. 2010; 12:354–358. PMID: 20190015.
24. Chanprapaph P, Tongsong T, Wanapirak C, Sirichotiyakul S, Sanguansermsri T. Prenatal diagnosis of alpha-thalassemia-1 (SEA type) by chorionic villus sampling. J Med Assoc Thai. 2002; 85:1049–1053. PMID: 12501894.
25. Tan AS, Quah TC, Low PS, Chong SS. A rapid and reliable 7-deletion multiplex polymerase chain reaction assay for α-thalassemia. Blood. 2001; 98:250–251. PMID: 11439976.


26. Fucharoen S, Winichagoon P. Thalassemia in SouthEast Asia: problems and strategy for prevention and control. Southeast Asian J Trop Med Public Health. 1992; 23:647–655. PMID: 1298071.
27. Fucharoen S, Winichagoon P, Thonglairoam V, Siriboon W, Siritanaratkul N, Kanokpongsakdi S, et al. Prenatal diagnosis of thalassemia and hemoglobinopathies in Thailand: experience from 100 pregnancies. Southeast Asian J Trop Med Public Health. 1991; 22:16–29. PMID: 1948258.
28. Chamras U, Sukunthamala K, Pornprasert S. SYTO9 and SYBR GREEN1 with high resolution melting analysis for molecular confirmatory testing of the common Southeast Asian β0-thalassemia mutations. Hemoglobin. 2009; 33:539–545. PMID: 19958204.
29. Yamsri S, Sanchaisuriya K, Fucharoen G, Sae-Ung N, Ratanasiri T, Fucharoen S. Prevention of severe thalassemia in northeast Thailand: 16 years of experience at a single university center. Prenat Diagn. 2010; 30:540–546. PMID: 20509153.


30. Winichagoon P, Fucharoen S, Kanokpongsakdi S, Fukumaki Y. Detection of alpha-thalassemia-1 (Southeast Asian type) and its application for prenatal diagnosis. Clin Genet. 1995; 47:318–320. PMID: 7554366.
31. Monis PT, Giglio S, Saint CP. Comparison of SYTO9 and SYBR Green I for real-time polymerase chain reaction and investigation of the effect of dye concentration on amplification and DNA melting curve analysis. Anal Biochem. 2005; 340:24–34. PMID: 15802126.


32. Giglio S, Monis PT, Saint CP. Demonstration of preferential binding of SYBR Green I to specific DNA fragments in real-time multiplex PCR. Nucleic Acids Res. 2003; 31:e136. PMID: 14602929.


33. Herrmann MG, Durtschi JD, Bromley LK, Wittwer CT, Voelkerding KV. Amplicon DNA melting analysis for mutation scanning and genotyping: cross-platform comparison of instruments and dyes. Clin Chem. 2006; 52:494–503. PMID: 16423901.


34. Krypuy M, Newnham GM, Thomas DM, Conron M, Dobrovic A. High resolution melting analysis for the rapid and sensitive detection of mutations in clinical samples: KRAS codon 12 and 13 mutations in non-small cell lung cancer. BMC Cancer. 2006; 6:295. PMID: 17184525.


Fig. 1
Representative results of dissociation curve analysis for α-thalassemia-1 SEA and Thai type deletions and β-thalassemia 3.5-kb deletion. DNA samples were obtained from 5 normal individuals, 5 individuals with α-thalassemia-1 SEA type deletion, 2 with α-thalassemia-1 Thai type deletion, and 2 with heterozygous β-thalassemia 3.5-kb gene deletions.
Abbreviations: dF/dT, derivative of the fluorescence/derivative of the temperature; SEA, Southeast Asian.
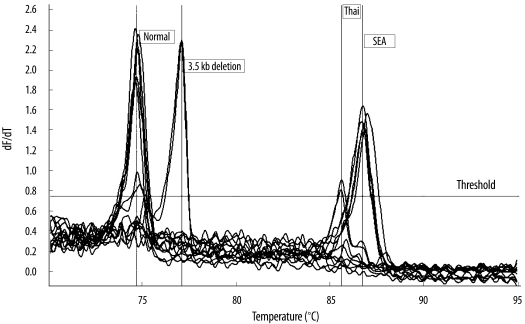