Abstract
Purpose
To investigate the effect of cysteamine on mixed peripheral blood mononuclear cells (PBMCs)-chemically injured kera-tocytes reaction (mixed lymphocyte-keratocyte reaction; MLKR).
Methods
PBMC stimulation assay was performed after keratocytes were chemically injured with 0.05 N NaOH for 60 seconds. MLKR was treated with various concentrations of cysteamine (0-10 mM). Intracellular reactive oxygen species (ROS) formation was measured using the oxidation-sensitive fluorescent probe, 2′7′-dichlorofluorescein diacetate (DCF-DA). Proliferation rate of PBMCs stimulated by NaOH-treated keratocytes and secretion profiles of matrix metalloprotease-9 (MMP-9), transforming growth factor-beta1 (TGF-β1), interleukin-6 (IL-6), and macrophage migration inhibitory factor (MIF) were determined using the bromodeoxyuridine proliferation assay and enzyme-linked immunosorbent assay, respectively.
Results
Proliferation rate of PMBCs was suppressed by cysteamine in a dose-dependent manner ( p = 0.019). Fluorescence of DCF-DA decreased depending on cysteamine concentration ( p < 0.001). MMP-9, IL-6 and TGF-β1 levels were suppressed by cyste-amine in a dose-dependent manner ( p < 0.05), whereas MIF levels increased with cysteamine concentration of 0.5-10 mM ( p = 0.008).
References
1. Wagoner MD. Chemical injuries of the eye: current concepts in pathophysiology and therapy. Surv Ophthalmol. 1997; 41:275–313.


2. Oh SY, Choi JS, Kim EJ. . The role of macrophage migration inhibitory factor in ocular surface disease pathogenesis after chem-ical burn in the murine eye. Mol Vis. 2010; 16:2402–11.
3. Shi W, Liu J, Li M. . Expression of MMP, HPSE, and FAP in stroma promoted corneal neovascularization induced by different etiological factors. Curr Eye Res. 2010; 35:967–77.


4. Planck SR, Rich LF, Ansel JC. . Trauma and alkali burns in-duce distinct patterns of cytokine gene expression in the rat cornea. Ocul Immunol Inflamm. 1997; 5:95–100.


6. Chung JH, Kim HJ, Fagerholmb P, Cho BC. Effect of topically ap-plied Na-hyaluronan on experimental corneal alkali wound healing. Korean J Ophthalmol. 1996; 10:68–75.


7. Pattamatta U, Willcox M, Stapleton F, Garrett Q. Bovine lacto-ferrin promotes corneal wound healing and suppresses IL-1 ex-pression in alkali wounded mouse cornea. Curr Eye Res. 2013; 38:1110–7.


8. Dohlman CH, Cade F, Pfister R. Chemical burns to the eye: para-digm shifts in treatment. Cornea. 2011; 30:613–4.


9. Kubota M, Shimmura S, Kubota S. . Hydrogen and N-ace-tyl-L-cysteine rescue oxidative stress-induced angiogenesis in a mouse corneal alkali-burn model. Invest Ophthalmol Vis Sci. 2011; 52:427–33.
10. Kessler A, Biasibetti M, da Silva Melo DA. . Antioxidant ef-fect of cysteamine in brain cortex of young rats. Neurochem Res. 2008; 33:737–44.


11. Wilmer MJ, Kluijtmans LA, van der Velden TJ. . Cysteamine restores glutathione redox status in cultured cystinotic proximal tubular epithelial cells. Biochim Biophys Acta. 2011; 1812:643–51.


12. Choi YM, Park CG, Lee HS, Hur WJ. The effects of cysteamine on the radiation-induced apoptosis. J Korean Soc Ther Radiol Oncol. 2000; 18:214–19.
13. Chakravarti S, Wu F, Vij N. . Microarray studies reveal macro-phage-like function of stromal keratocytes in the cornea. Invest Ophthalmol Vis Sci. 2004; 45:3475–84.


14. Funderburgh JL, Funderburgh ML, Mann MM. . Proteoglycan expression during transforming growth factor beta-induced kerato-cyte-myofibroblast transdifferentiation. J Biol Chem. 2001; 276:44173–8.
15. Bourcier T, Borderie V, Forgez P. . In vitro effects of dex-amethasone on human corneal keratocytes. Invest Ophthalmol Vis Sci. 1999; 40:1061–70.
16. Borderie VM, Lopez M, Lombet A. . Cryopreservation and culture of human corneal keratocytes. Invest Ophthalmol Vis Sci. 1998; 39:1511–19.
17. Musselmann K, Kane BP, Hassell JR. Isolation of a putative kera-tocyte activating factor from the corneal stroma. Exp Eye Res. 2003; 77:273–9.


18. Mosmann T. Rapid colorimetric assay for cellular growth and sur-vival: application to proliferation and cytotoxicity assays. J Immunol Methods. 1983; 65:55–63.


19. Pathak S, Goldofsky E, Vivas EX. . IL-1β is overexpressed and aberrantly regulated in corticosteroid nonresponders with auto-immune inner ear disease. J Immunol. 2011; 186:1870–9.


20. Yang L, Hou Y. Different characters of spleen OX-62 positive den-dritic cells between Fischer and Lewis rats. Cell Mol Immunol. 2006; 3:145–50.
21. Shin YJ, Kim JH, Seo JM. . Protective effect of clusterin on ox-idative stress-induced cell death of human corneal endothelial cells. Mol Vis. 2009; 15:2789–95.
22. Wagoner MD. Chemical injuries of the eye: current concepts in pathophysiology and therapy. Surv Ophthalmol. 1997; 41:275–313.


23. Rahman I, Adcock IM. Oxidative stress and redox regulation of lung inflammation in COPD. Eur Respir J. 2006; 28:219–42.


24. Saika S. TGF-beta signal transduction in corneal wound healing as a therapeutic target. Cornea. 2004; 23:(8 Suppl). S25–30.
25. Jester JV, Barry PA, Lind GJ. . Corneal keratocytes: in situ and in vitro organization of cytoskeletal contractile proteins. Invest Ophthalmol Vis Sci. 1994; 35:730–43.
26. Katakami C, Fujisawa K, Sahori A. . Localization of collagen (I) and collagenase mRNA by in situ hybridization during corneal wound healing after epikeratophakia or alkali-burn. Jpn J Ophthalmol. 1992; 36:10–22.
27. Chakravarti S, Wu F, Vij N. . Microarray studies reveal macro-phage-like function of stromal keratocytes in the cornea. Invest Ophthalmol Vis Sci. 2004; 45:3475–84.


28. Bryan N, Ahswin H, Smart N. . Reactive oxygen species (ROS)-a family of fate deciding molecules pivotal in constructive inflammation and wound healing. Eur Cell Mater. 2012; 24:249–65.
29. Kovac S, Anqelova PR, Holmström KM. . Nrf2 regulates ROS production by mitochondria and NADPH oxidase. Biochim Biophys Acta. 2015; 1850:794–801.


30. Misík V, Miyoshi N, Riesz P. Effects of cysteamine and cystamine on the sonochemical accumulation of hydrogen peroxide- im-plications for their mechanisms of action in ultrasound-exposed cells. Free Radic Biol Med. 1999; 26:961–7.
31. Chen M, Matsuda H, Wang L. . Pretranscriptional regulation of Tgf-beta1 by PI polyamide prevents scarring and accelerates wound healing of the cornea after exposure to alkali. Mol Ther. 2010; 18:519–27.
32. Saika S, Ikeda K, Yamanaka O. . Expression of Smad7 in mouse eyes accelerates healing of corneal tissue after exposure to alkali. Am J Pathol. 2005; 166:1405–18.


33. Zieske JD, Hutcheon AE, Guo X. . TGF-beta receptor types I and II are differentially expressed during corneal epithelial wound repair. Invest Ophthalmol Vis Sci. 2001; 42:1465–71.
34. Sambursky R, O'Brien TP. MMP-9 and the perioperative manage-ment of LASIK surgery. Curr Opin Ophthalmol. 2011; 22:294–303.


35. Chang JH, Han KY, Azar DT. Wound healing fibroblasts modulate corneal angiogenic privilege: interplay of basic fibroblast growth factor and matrix metalloproteinases in corneal angiogenesis. Jpn J Ophthalmol. 2010; 54:199–205.


36. Calandra T, Roger T. Macrophage migration inhibitory factor: a regulator of innate immunity. Nat Rev Immunol. 2003; 3:791–800.


37. Daun JM, Cannon JG. Macrophage migration inhibitory factor an-tagonizes hydrocortisone-induced increases in cytosolic IkappaBalpha. Am J Physiol Regul Integr Comp Physiol. 2000; 279:R1043–49.
Figure 1.
Inverted phase-contrast image and immunofluorescent vimentin staining of human keratocytes. (A) Keratocytes were ob-served growing parallel to the etched lines. Magnification, ×200. (B) Immunofluorescence with anti-vimentin antibody, a general marker of keratocyte, revealed the morphology. The nuclei were stained using Hoechst 33342 (blue). Magnification, ×400.

Figure 2.
Peripheral blood monocular cell (PBMC) stimulation test. NaOH-treated human keratocytes served as the stimulators. Cysteamine suppressed the PBMC proliferation in a dose-de-pendent manner ( p = 0.019, Kruskal-Wallis test). PBMC pro-liferation was suppressed with ≥5 mM of cysteamine ( p = 0.034 and 0.021, respectively, Mann-Whitney U-test). PBMC pro-liferation with 0-5 mM of cysteamine was higher than that of neg-ative control ( p = 0.008 for all, Mann- Whitney U-test), whereas it was lower than that of negative control in 10 mM of cysteamine * Statistically sig-( p = 0.008). BrdU = bromodeoxyuridine. nificant by Mann-Whitney U-test.
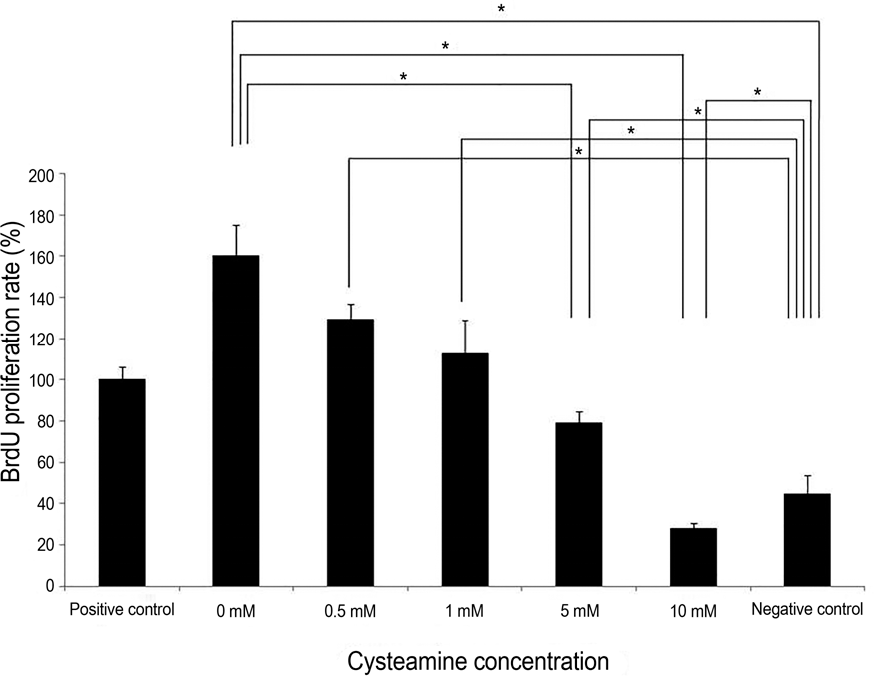
Figure 3.
Relative 2’,7’-dichlorofluorescein diacetate (DCF- DA) fluorescence. Intracellular ROS levels measured by rela-tive DCF fluorescence decreased by 0-10 mM cysteamine in a dose-dependent manner ( p < 0.001; Kruskal-Wallis test). Cyst-eamine concentration of ≥1 mM significantly lowered the intra-cellular ROS levels ( p = 0.014, 0.014 and 0.014, respectively; Mann-Whitney U-test). Intracellular ROS levels with 0-1 mM of cysteamine was higher than that of negative control ( p = 0.016, 0.008, and 0.008, respectively, Mann-Whitney U-test). However, there was no significant difference of intracelluar ROS level between 5-10 mM of cysteamine and negative control. ROS = reactive oxygen species; DCF = 2‘7’-dichl-oroflurescein. * Statistically significant by Mann-Whitney U-test.
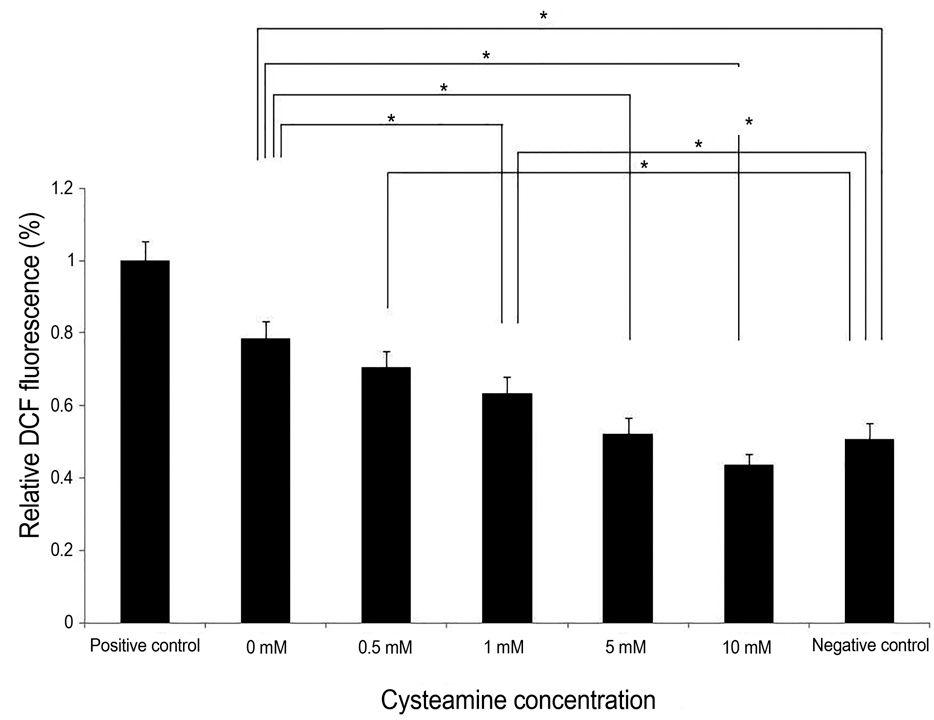
Figure 4.
MMP-9 levels measured by ELISA. MMP-9 levels decreased in a dose-dependent manner by 0-10 mM cysteamine ( p = 0.001; Kruskal-Wallis test). Cysteamine concentration of ≥5 mM significantly lowered the MMP-9 levels ( p = 0.016, and 0.009, respectively; Mann-Whitney U-test). MMP-9 lev-els with 0-5 mM of cysteamine was higher compared with neg-ative control ( p = 0.016, 0.016, 0.008, and 0.008, respectively, Mann-Whitney U-test). However, there was no significant dif-ference of intracelluar ROS level between 10 mM of cysteamine and negative control. MMP-9 = matrix metalloprotease-9; ELISA = enzyme-linked immunosorbent assay; ROS = reactive oxygen species. * Statistically significant by Mann-Whitney U-test.
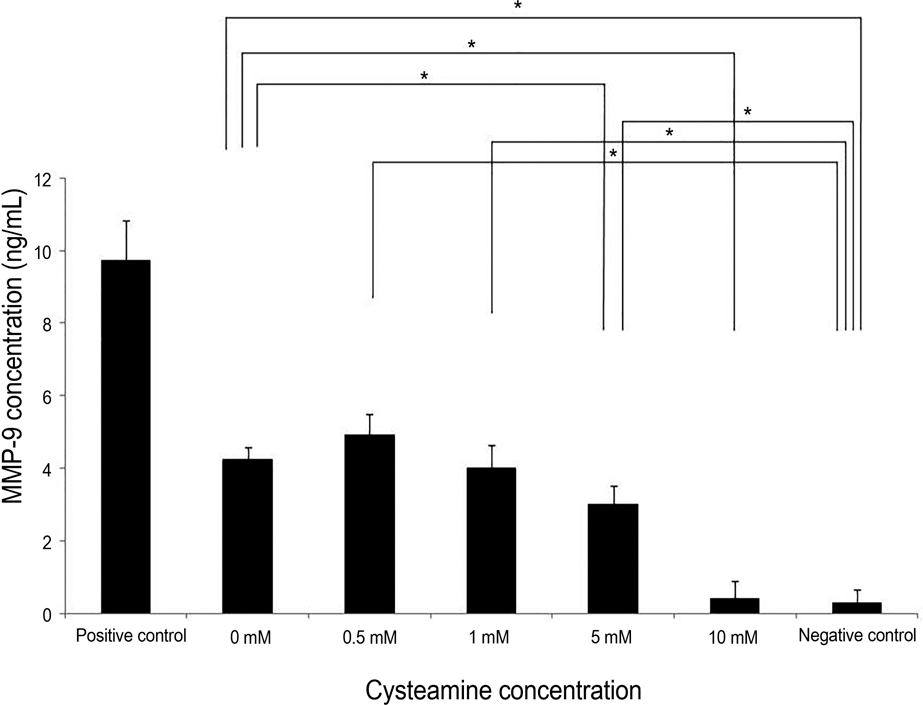
Figure 5.
IL-6 levels measured by ELISA. Cysteamine decreased IL-6 levels in a dose-dependent manner ( p = 0.003; Kruskal- Wallis test). Cysteamine concentration of ≥1 mM significantly lowered the IL-6 levels ( p = 0.043, 0.021, and 0.021, respectively; Mann-Whitney U-test). IL-6 levels with 0-0.5 mM of cysteamine was higher than that of negative control ( p = 0.016, and 0.032, respectively, Mann-Whitney U-test), whereas IL-6 levels with cysteamine concentration of ≥5 mM were lower than that of neg-ative control ( p = 0.032, and 0.016, respectively; Mann-Whitney U-test). IL-6 = interleukin-6; ELISA = enzyme-linked immunosorbent assay. * Statistically significant by Mann- Whitney U-test.
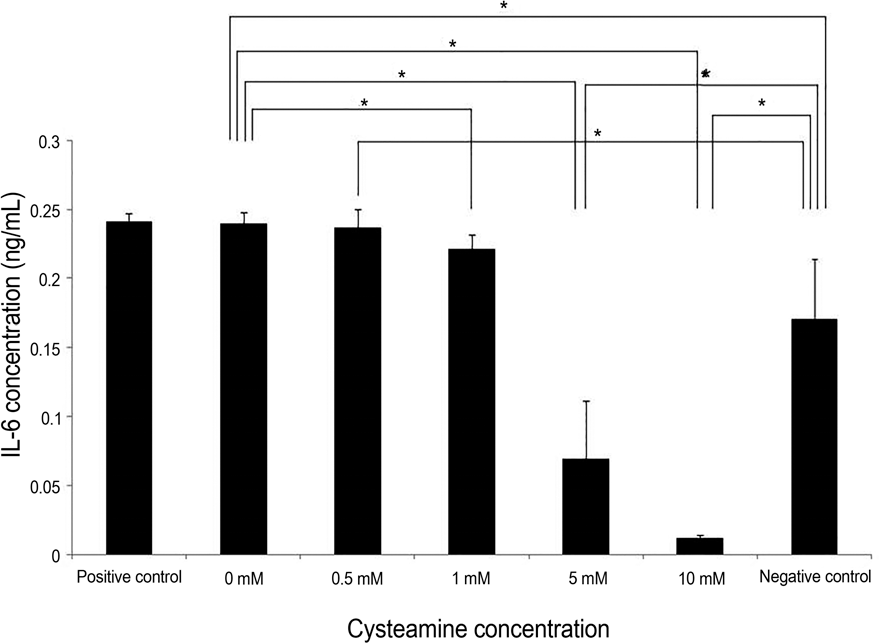
Figure 6.
TGF-β1 levels measured by ELISA. TGF-β1 level was slightly elevated in a low (0.05 mM) cysteamine concen-tration ( p = 0.016; Mann-Whitney U-test). However, it de-creased at high concentrations (5 mM and 10 mM) ( p = 0.009 and 0.009; Mann-Whitney U-test). TGF-β1 levels with 0.5 mM of cysteamine was higher than that of negative control ( p = 0.016, Mann-Whitney U-test), whereas TGF-β1 levels with cysteamine concentration of ≥5 mM were lower than that of negative control ( p = 0.016 for both). TGF-β1 = trans-forming growth factor-beta 1; ELISA = enzyme-linked im-munosorbent assay. * Statistically significant by Mann-Whitney U-test.
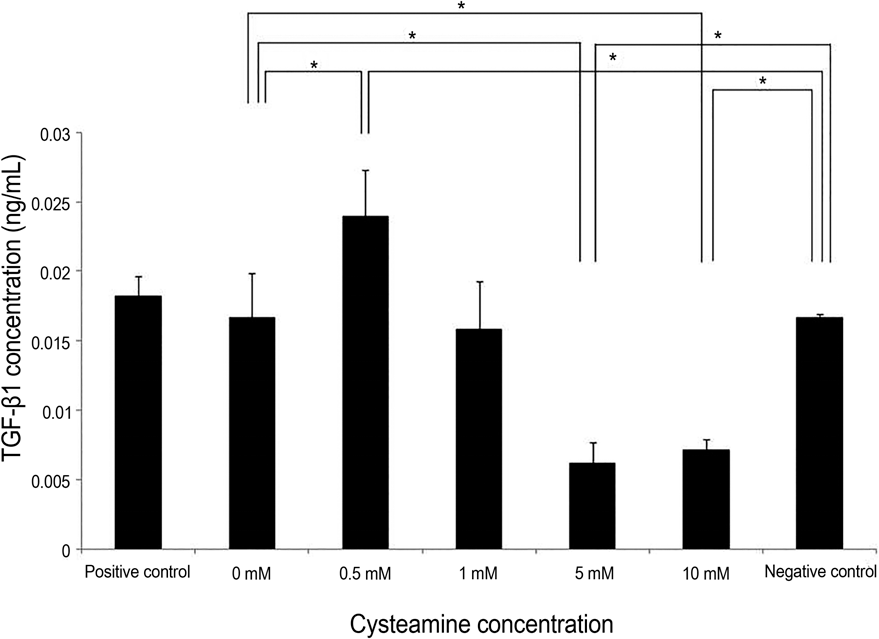
Figure 7.
MIF levels measured by ELISA. Cysteamine in-creased MIF levels at high concentrations (≥ 1 mM) ( p = 0.014, 0.014, and 0.027, respectively; Mann-Whitney U-test). MIF levels with 0-10 mM of cysteamine was higher than that of negative control ( p = 0.016, 0.008, 0.008, 0.008, and 0.008, respectively). MIF = macrophage migration inhibitory factor; * Statistically ELISA = enzyme-linked immunosorbent assay. significant by Mann-Whitney U-test.
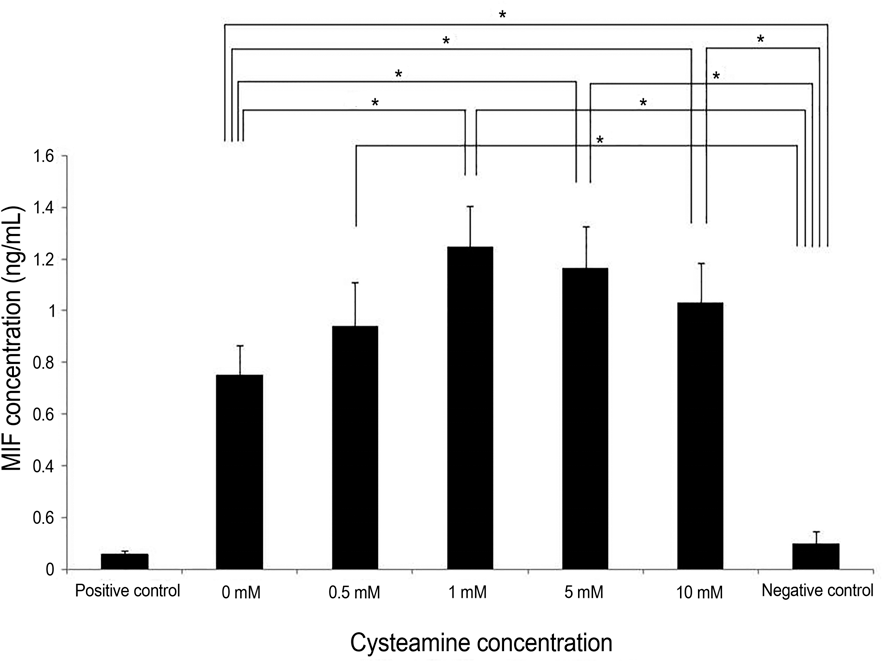