Abstract
Purpose
To measure the lamina cribrosa thickness (LCT) in vivo of healthy people and to determine any association be-tween the LCT and age or sex.
Methods
We evaluated 100 eyes of 100 healthy volunteers. Forty eyes were selected and divided into 2 specific age groups (38-49 and 78-89 years) and the differences were assessed with respect to age and sex. Each participant under-went a complete eye examination and the LCT was evaluated with spectral domain optical coherence tomography (OCT) using enhanced depth imaging (EDI) mode.
Results
Eighty-seven eyes were considered for the measurement and analysis of the LCT and 13 eyes were excluded be-cause of an unclear image for identifying the LCT. The mean age was 55.0 ± 19.5 years. The mean LCT in healthy volun-teers was 231.3 ± 41.6 μ m (range, 152.5 - 327.5 μ m) and a negative relationship was found between LCT and age (LCT = -1.614 × age + 320.191 μ m, 95% CI for slope -1.91 to -1.32, r2 = 0.572, p < 0.001). Regarding differences related to sex, males had relatively thicker LCT than females, irrespective of age, although not statistically significant (p = 0.623).
References
1. Crawford Downs J, Roberts MD, Sigal IA. Glaucomatous cupping of the lamina cribrosa: a review of the evidence for active pro-gressive remodeling as a mechanism. Exp Eye Res. 2011; 93:133–40.
2. Kourkoutas D, Karanasiou IS, Tsekouras GJ. . Glaucoma risk assessment using a non-linear multivariable regression method. Comput Methods Programs Biomed. 2012; 108:1149–59.


3. Kotecha A, Izadi S, Jeffery G. Age-related changes in the thickness of the human lamina cribrosa. Br J Ophthalmol. 2006; 90:1531–4.


5. Anderson DR. Ultrastructure of human and monkey lamina cri-brosa and optic nerve head. Arch Ophthalmol. 1969; 82:800–14.


6. Emery JM, Landis D, Paton D. . The lamina cribrosa in normal and glaucomatous human eyes. Trans Am Acad Ophthalmol Otolaryngol. 1974; 78:OP290–7.
7. Park HY, Jeon SH, Park CK. Enhanced depth imaging detects lami-na cribrosa thickness differences in normal tension glaucoma and primary open-angle glaucoma. Ophthalmology. 2012; 119:10–20.


8. Gaasterland D, Tanishima T, Kuwabara T. Axoplasmic flow during chronic experimental glaucoma. 1. Light and electron microscopic studies of the monkey optic nervehead during development of glaucomatous cupping. Invest Ophthalmol Vis Sci. 1978; 17:838–46.
9. Minckler DS, Bunt AH, Johanson GW. Orthograde and retrograde axoplasmic transport during acute ocular hypertension in the monkey. Invest Ophthalmol Vis Sci. 1977; 16:426–41.
10. Quigley HA, Addicks EM, Green WR, Maumenee AE. Optic nerve damage in human glaucoma. II. The site of injury and suscepti-bility to damage. Arch Ophthalmol. 1981; 99:635–49.


11. Quigley HA, Green WR. The histology of human glaucoma cup-ping and optic nerve damage: clinicopathologic correlation in 21 eyes. Ophthalmology. 1979; 86:1803–30.


12. Bellezza AJ, Rintalan CJ, Thompson HW. . Deformation of the lamina cribrosa and anterior scleral canal wall in early experimental glaucoma. Invest Ophthalmol Vis Sci. 2003; 44:623–37.


13. Burgoyne CF, Downs JC. Premise and prediction-how optic nerve head biomechanics underlies the susceptibility and clinical behav-ior of the aged optic nerve head. J Glaucoma. 2008; 17:318–28.


14. Albon J, Purslow PP, Karwatowski WS, Easty DL. Age related compliance of the lamina cribrosa in human eyes. Br J Ophthalmol. 2000; 84:318–23.


15. Albon J, Karwatowski WS, Easty DL. . Age related changes in the non-collagenous components of the extracellular matrix of the human lamina cribrosa. Br J Ophthalmol. 2000; 84:311–7.


16. Inoue R, Hangai M, Kotera Y. . Three-dimensional high-speed optical coherence tomography imaging of lamina cribrosa in glaucoma. Ophthalmology. 2009; 116:214–22.


17. Kagemann L, Ishikawa H, Wollstein G. . Ultrahigh-resolution spectral domain optical coherence tomography imaging of the lam-ina cribrosa. Ophthalmic Surg Lasers Imaging. 2008; 39:(4 Suppl). S126–31.


18. Strouthidis NG, Grimm J, Williams GA. . A comparison of op-tic nerve head morphology viewed by spectral domain optical co-herence tomography and by serial histology. Invest Ophthalmol Vis Sci. 2010; 51:1464–74.


19. Yeoh J, Rahman W, Chen F. . Choroidal imaging in inherited retinal disease using the technique of enhanced depth imaging opti-cal coherence tomography. Graefes Arch Clin Exp Ophthalmol. 2010; 248:1719–28.


20. Dell'Omo R, Costagliola C, Di Salvatore F. . Enhanced depth imaging spectral-domain optical coherence tomography. Retina. 2010; 30:378–9.
21. Zlotnik A, Ben-Yaish S, Zalevsky Z. Extending the depth of focus for enhanced three-dimensional imaging and profilometry: an overview. Appl Opt. 2009; 48:H105–12.


22. Imamura Y, Fujiwara T, Margolis R, Spaide RF. Enhanced depth imaging optical coherence tomography of the choroid in central se-rous chorioretinopathy. Retina. 2009; 29:1469–73.


23. Fujiwara T, Imamura Y, Margolis R. . Enhanced depth imaging optical coherence tomography of the choroid in highly myopic eyes. Am J Ophthalmol. 2009; 148:445–50.


24. Margolis R, Spaide RF. A pilot study of enhanced depth imaging optical coherence tomography of the choroid in normal eyes. Am J Ophthalmol. 2009; 147:811–5.


25. Spaide RF. Enhanced depth imaging optical coherence tomog-raphy of retinal pigment epithelial detachment in age-related mac-ular degeneration. Am J Ophthalmol. 2009; 147:644–52.


26. Wong IY, Koizumi H, Lai WW. Enhanced depth imaging optical coherence tomography. Ophthalmic Surg Lasers Imaging. 2011; 42:Suppl. S75–84.


27. Fleiss JL, Levin B, Paik MC. Statistical Methods for Rates and Proportions. 3rd ed. Hoboken, NJ: Wiley-Interscience;2003; 604.


28. Lee EJ, Kim TW, Weinreb RN. . Visualization of the lamina cribrosa using enhanced depth imaging spectral-domain optical co-herence tomography. Am J Ophthalmol. 2011; 152:87–95.e1.


30. Damji KF, Freedman S, Moroi SE. . Shields' Textbook of Glaucoma. 5th ed. Philadelphia: Lippincott Williams & Wilkins;2005; 73–115.
31. Burgoyne CF, Quigley HA, Thompson HW. . Early changes in optic disc compliance and surface position in experimental glaucoma. Ophthalmology. 1995; 102:1800–9.


32. Burgoyne CF, Morrison JC. The anatomy and pathophysiology of the optic nerve head in glaucoma. J Glaucoma. 2001; 10:(5 Suppl 1). S16–8.


33. Quigley HA, Brown A, Dorman-Pease ME. Alterations in elastin of the optic nerve head in human and experimental glaucoma. Br J Ophthalmol. 1991; 75:552–7.


34. Quigley HA, Guy J, Anderson DR. Blockade of rapid axonal transport. Effect of intraocular pressure elevation in primate optic nerve. Arch Ophthalmol. 1979; 97:525–31.


35. Savini G, Carbonelli M, Parisi V, Barboni P. Repeatability of optic nerve head parameters measured by spectral-domain OCT in healthy eyes. Ophthalmic Surg Lasers Imaging. 2011; 42:209–15.


36. Sharma A, Oakley JD, Schiffman JC. . Comparison of auto-mated analysis of Cirrus HD OCT spectral-domain optical coher-ence tomography with stereo photographs of the optic disc. Ophthalmology. 2011; 118:1348–57.


37. Abràmoff MD, Lee K, Niemeijer M. . Automated segmentation of the cup and rim from spectral domain OCT of the optic nerve head. Invest Ophthalmol Vis Sci. 2009; 50:5778–84.


38. Sung KR, Kim JS, Wollstein G. . Imaging of the retinal nerve fibre layer with spectral domain optical coherence tomography for glaucoma diagnosis. Br J Ophthalmol. 2011; 95:909–14.


39. Park SC, De Moraes CG, Teng CC. . Enhanced depth imaging optical coherence tomography of deep optic nerve complex structures in glaucoma. Ophthalmology. 2012; 119:3–9.


40. Quigley HA, Hohman RM, Addicks EM. . Morphologic changes in the lamina cribrosa correlated with neural loss in open- angle glaucoma. Am J Ophthalmol. 1983; 95:673–91.
41. Ogden TE, Duggan J, Danley K. . Morphometry of nerve fiber bundle pores in the optic nerve head of the human. Exp Eye Res. 1988; 46:559–68.


42. Jonas JB, Berenshtein E, Holbach L. Lamina cribrosa thickness and spatial relationships between intraocular space and cere-brospinal fluid space in highly myopic eyes. Invest Ophthalmol Vis Sci. 2004; 45:2660–5.


43. Ren R, Wang N, Li B. . Lamina cribrosa and peripapillary sclera histomorphometry in normal and advanced glaucomatous Chinese eyes with various axial length. Invest Ophthalmol Vis Sci. 2009; 50:2175–84.


44. Sigal IA, Flanagan JG, Tertinegg I, Ethier CR. Finite element mod-eling of optic nerve head biomechanics. Invest Ophthalmol Vis Sci. 2004; 45:4378–87.


46. Levy NS, Crapps EE. Displacement of optic nerve head in response to short-term intraocular pressure elevation in human eyes. Arch Ophthalmol. 1984; 102:782–6.


47. Radius RL. Anatomy of the optic nerve head and glaucomatous op-tic neuropathy. Surv Ophthalmol. 1987; 32:35–44.


48. Jonas JB, Berenshtein E, Holbach L. Anatomic relationship be-tween lamina cribrosa, intraocular space, and cerebrospinal fluid space. Invest Ophthalmol Vis Sci. 2003; 44:5189–95.


49. Yang H, Downs JC, Girkin C. . 3-D histomorphometry of the normal and early glaucomatous monkey optic nerve head: lamina cribrosa and peripapillary scleral position and thickness. Invest Ophthalmol Vis Sci. 2007; 48:4597–607.


50. Lee EJ, Kim TW, Weinreb RN. . Lamina cribrosa thickness is not correlated with central corneal thickness or axial length in healthy eyes: central corneal thickness, axial length, and lamina cribrosa thickness. Graefes Arch Clin Exp Ophthalmol. 2013; 251:847–54.
Figure 1.
Change in lamina cribrosa thickness (LCT, μ m) with age. Regression line : LCT = -1.614 × age + 320.191 μ m, 95% CI for slope -1.91 to -1.32, r2=0.572, p < 0.001. AvgLCT = average lamina cribrosa thickness.
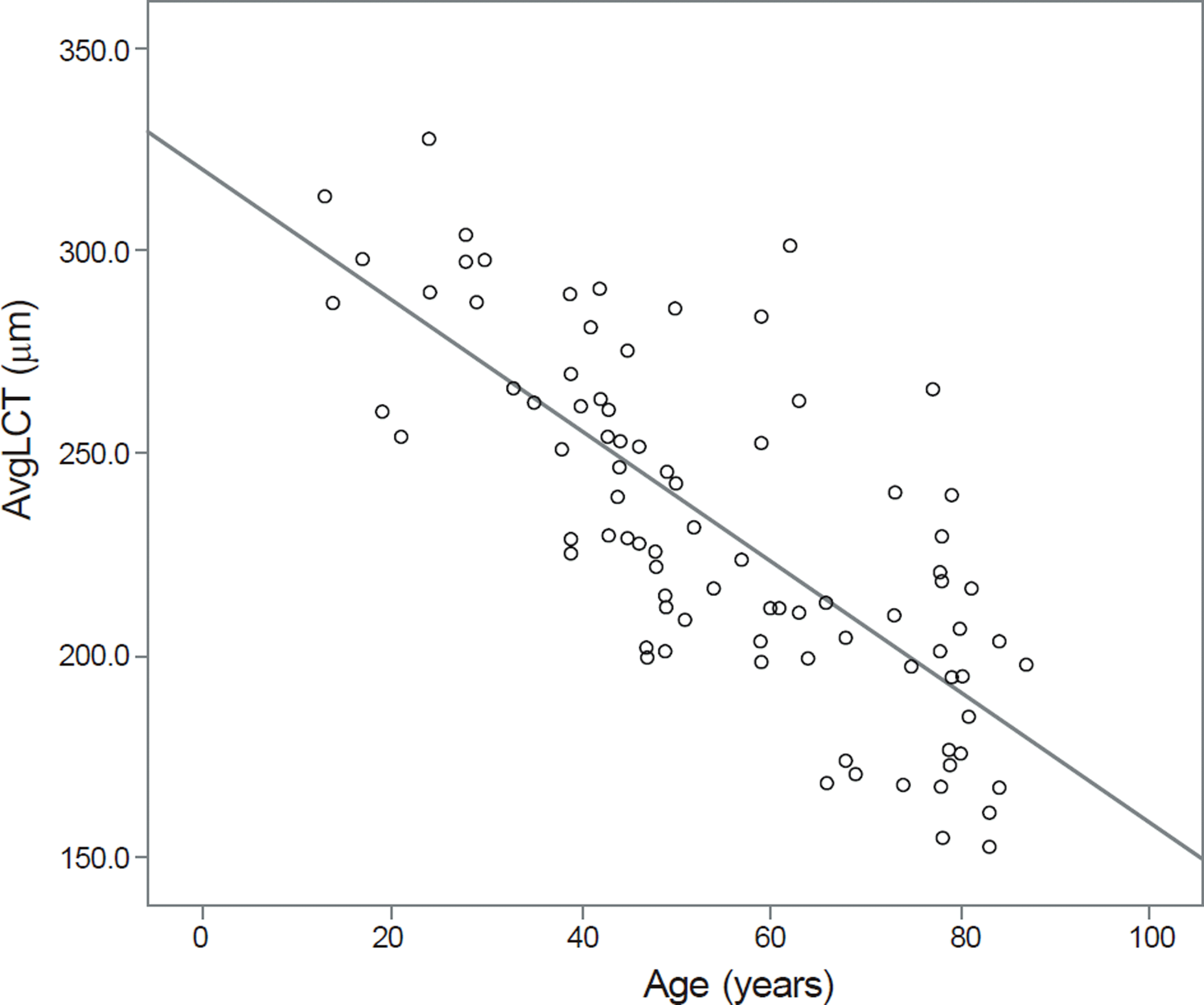
Figure 2.
Differences with respect to sex and age in lamina cri-brosa thickness (LCT, μ m) in each group. Group 1: 38-49 years,10 men and 10 women, mean 240.40 (SD 24.30) μ m; Group 2:78-87 years, 10 men and 10 women, mean 191.65 (SD 25.31) μ m; unpaired t-test, p < 0.01.
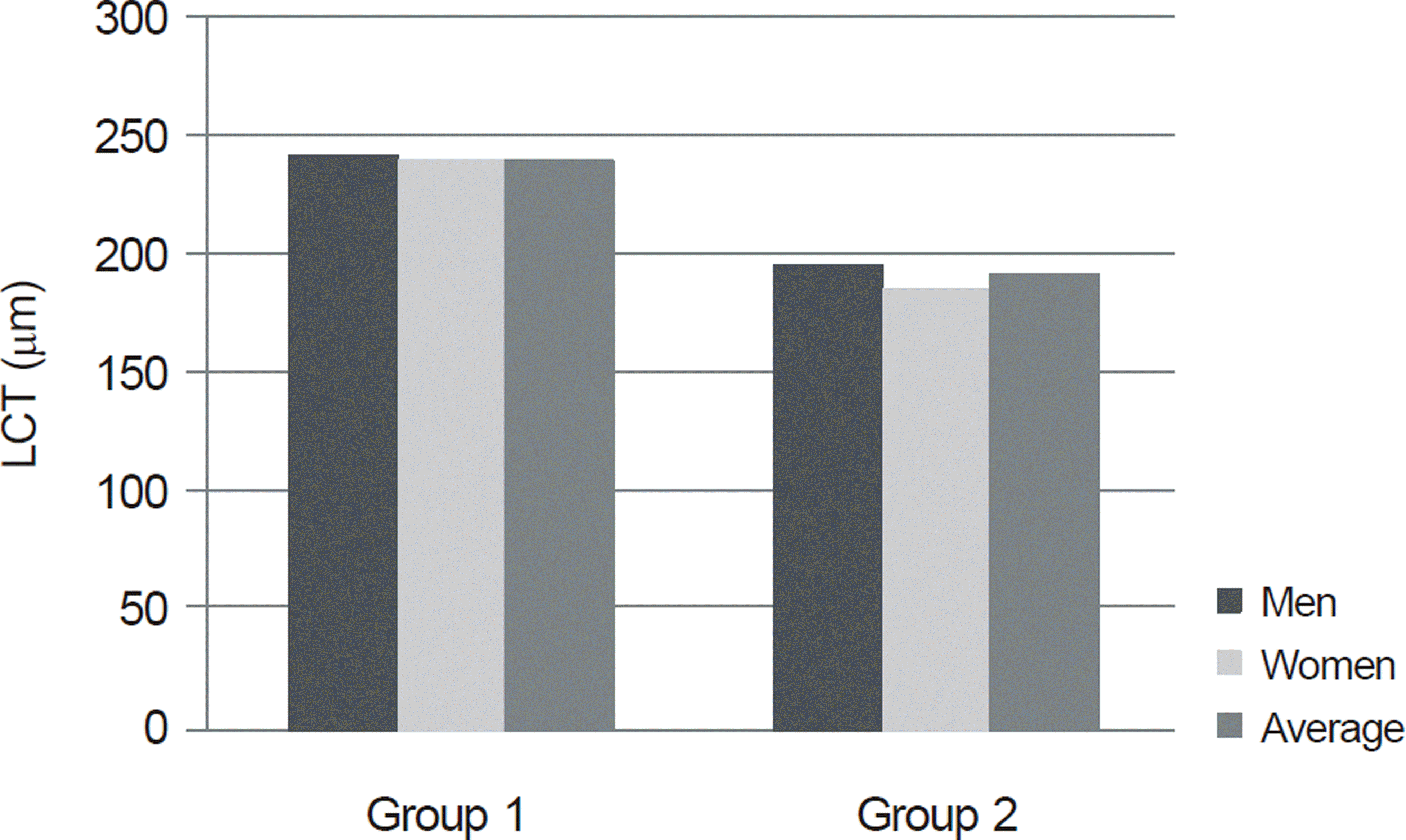
Table 1.
Characteristics of the overall subjects
Table 2.
Reproducibility of the lamina cribrosa thickness measurements
Mean (n = 87) | Standard deviation | ICC | |
---|---|---|---|
Intraobserver | |||
Observer 1 | 231.41 | 41.976 | 0.992 (0.987-0.994) |
Observer 2 | 231.28 | 41.408 |
Table 3.
Multiple comparison results
(I*) Group (years) | (J†) Group (years) | Mean difference (I-J) (μ m) | Standard error (μ m) | p-value |
---|---|---|---|---|
10-29 | 30-49 | 45.84677 | 10.85231 | 0.000 |
50-69 | 69.25000 | 11.46522 | 0.000 | |
70-89 | 95.19000 | 11.16542 | 0.000 | |
30-49 | 10-29 | -45.84677 | 10.85231 | 0.000 |
50-69 | 23.40323 | 8.43378 | 0.034 | |
70-89 | 49.34323 | 8.02147 | 0.000 | |
50-69 | 10-29 | -69.25000 | 11.46522 | 0.000 |
30-49 | -23.40323 | 8.43378 | 0.034 | |
70-89 | 25.94000 | 8.83304 | 0.022 | |
70-89 | 10-29 | -95.19000 | 11.16542 | 0.000 |
30-49 | -49.34323 | 8.02147 | 0.000 | |
50-69 | -25.94000 | 8.83304 | 0.022 |